Advancements in Protein Mass Spectrometry Techniques
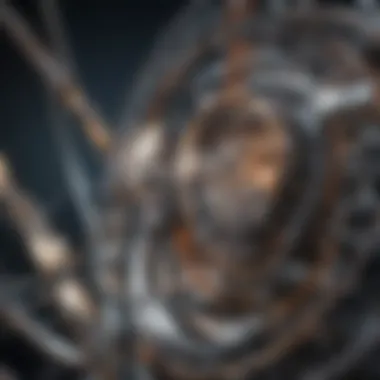
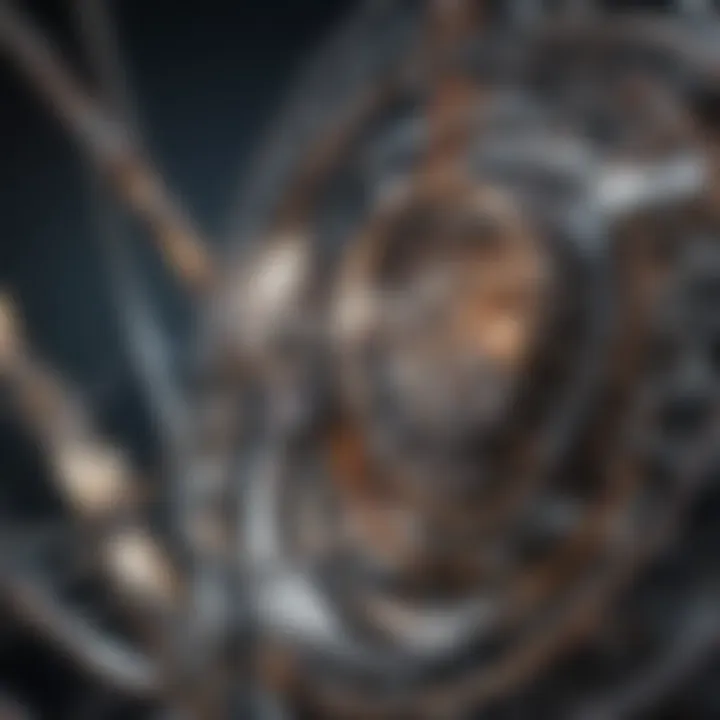
Article Overview
Purpose of the Article
The intention behind this article is to elucidate the intricate methodologies and applications of protein mass spectrometry. In doing so, it seeks to articulate how this pivotal technique serves as a backbone within modern biochemistry, paving the way for advancements in research and industry alike.
Relevance to Multiple Disciplines
Protein mass spectrometry holds significance not just within the realms of biochemistry but also intersects with fields such as molecular biology, pharmacology, and personalized medicine. This interplay opens doors for collaborative insights and applications, making it an invaluable tool for diverse scientific endeavors.
Research Background
Historical Context
To appreciate the depth of protein mass spectrometry, it’s essential to understand its evolution. The technique first saw the light of day in the early 20th century, but the real game changer came in the 1980s with the introduction of soft ionization methods like Electrospray Ionization (ESI) and Matrix-Assisted Laser Desorption/Ionization (MALDI). These advancements significantly broadened the scope and applicability of mass spectrometry, allowing scientists to analyze larger biomolecules and complex mixtures with far greater precision.
Key Concepts and Definitions
Mass spectrometry, at its core, operates on three key principles: ionization, separation, and detection.
- Ionization involves converting molecules into ions, which can then be manipulated by electric and magnetic fields.
- Separation refers to the process where ions are distributed in space and time based on their mass-to-charge ratio.
- Detection is the final step where these ions are converted into a measurable signal, allowing for analysis.
Understanding these principles is crucial for delving into the nitty-gritty of analytical methods utilized in protein characterization, including both advantages and challenges.
"Mass spectrometry has transformed the way we understand proteins and their roles in living organisms, offering unparalleled insights into their structure and function."
"Mass spectrometry has transformed the way we understand proteins and their roles in living organisms, offering unparalleled insights into their structure and function."
In subsequent sections, we will explore various analytical approaches, case studies demonstrating the impact of this technique, and its implications for the future of biochemistry and beyond.
Prologue to Protein Mass Spectrometry
Protein mass spectrometry holds a crucial place in the realm of modern biochemistry. It serves as a powerful analytical technique that allows scientists to dissect and understand proteins' composition, structure, and functions. This introduction outlines the significance of this technology, illustrating how it shapes contemporary research and development across various fields.
The importance of protein mass spectrometry can’t be overstated. It opens doors for breakthroughs in proteomics, which studies the entire set of proteins produced in an organism. As we dig deeper into the molecular world, understanding the dynamics of proteins becomes integral for fields like drug development, diagnosis of diseases, and personalized medicine. Mass spectrometry enables researchers to obtain precise data about proteins, including their mass, structure, and interactions, all of which are critical in uncovering biological pathways.
In examining protein mass spectrometry, several elements merit attention. First, the high sensitivity and specificity of the technique make it an invaluable tool for characterizing proteins in complex mixtures, often containing thousands of different entities. Second, it provides not only qualitative but also quantitative data, facilitating comparative studies that reflect the biological fluctuations under various experimental conditions. Lastly, advancements in instrument technology and methodologies continue to enhance the robustness and efficacy of mass spectrometry, keeping it at the forefront of scientific inquiry.
Nevertheless, there are considerations to keep in mind. Selecting the appropriate ionization technique or mass analyzer can significantly influence the data obtained. Researchers must also grapple with die complexities of data interpretation, often requiring sophisticated software and statistical analysis to draw meaningful conclusions.
Overall, grappling with the depths of protein mass spectrometry is not just a technical endeavor; it is instrumental in pushing the scientific envelope. By laying the groundwork in this section, we will seamlessly transition into the profound definitions and historical backdrop that shaped this field.
Definition and Overview
Protein mass spectrometry can be defined as an analytical method that measures the mass-to-charge ratio of charged particles. In practical terms, it involves the ionization of proteins and subsequent analysis of the resulting ions to determine their mass, often with very high precision. This technique has evolved as a critical part of modern biochemical research, enabling the identification and characterization of proteins in unprecedented detail.
To put it simply, mass spectrometry provides information about the molecular weight of proteins, which can then be correlated with their sequence and structure. This is enabled through various ionization techniques, which transform proteins into ions that can be manipulated and analyzed. By capturing the mass of these ions and analyzing their fragmentation patterns, researchers can deduce the identity and structure of the original molecules.
Historical Background
The history of protein mass spectrometry is a fascinating journey that reflects the evolution of technology and scientific thought. In the mid-20th century, the roots of mass spectrometry can be traced back to breakthroughs in physics, specifically the development of the first mass spectrometers. Early devices were rudimentary and offered limited functionality, primarily suited for small molecules.
However, it wasn’t until the advent of soft ionization techniques in the 1980s, like Electrospray Ionization (ESI) and Matrix-Assisted Laser Desorption/Ionization (MALDI), that the field experienced a significant leap. These innovations allowed scientists to analyze larger biomolecules, such as proteins, without breaking them apart into fragments. This opened new avenues in biological research, propelling mass spectrometry into a prominent role in proteomics.
Fast forward to today, mass spectrometry continues to experience rapid advancements, driven by technological innovations and increasing computational power. The introduction of high-resolution mass spectrometers and advanced data analysis techniques has transformed how proteins are studied, providing insights that were once thought impossible. As the world of biochemistry continues to expand, the historical foundation laid by early pioneers remains crucial to understanding the potential of protein mass spectrometry.
Fundamental Principles of Mass Spectrometry
Mass spectrometry stands at the forefront of analytical techniques, crucial for elucidating biological phenomena at the molecular level. Grasping the fundamental principles of this technology is essential to understand its potential and limitations in various scientific realms. This section discuses key elements such as ionization techniques, mass analyzers, and detection methods that serve as the backbone of mass spectrometry. With these principles, researchers leap into the unknown, uncovering insights that were once shrouded in mystery.
Ionization Techniques
Electrospray Ionization
Electrospray ionization (ESI) has become a bedrock technique pivotal to the realm of mass spectrometry. Particularly, it enables the analysis of biomolecules and large proteins that are otherwise challenging to handle. Essentially, ESI involves spraying a solution of analytes through a charged capillary. The resulting fine droplets evaporate, leading to ionized molecules that can be analyzed by the mass spectrometer.
A primary characteristic of ESI is its ability to produce ions from very dilute solutions, making it exceptionally useful for analyzing samples that contain proteins in trace amounts. This aspect makes ESI a favored choice in proteomic applications where sample availability often limits the discovery of potential biomarkers.
A unique feature of electrospray ionization is its compatibility with liquid chromatography, permitting online coupling and high-throughput capabilities. However, the technique can sometimes introduce variability in ion abundance due to ion suppression effects, influenced by the complex mixture often present in biological samples.
Matrix-Assisted Laser Desorption/Ionization
On another front lies matrix-assisted laser desorption/ionization (MALDI), another vital ionization method in mass spectrometry. This approach employs a laser to strike a matrix material mixed with the analyte. The laser energy absorbed by the matrix leads to desorption and ionization of the sample.
MALDI is particularly lauded for its efficiency in generating singly charged ions, which simplifies the analysis of complex biomolecules. The technique is known for its speed and the minimal sample preparation it requires, making it quite beneficial for rapid analyses. A unique quality of MALDI is its potential to analyze large biomolecules, helping to interpret the structure of proteins and their interactions.
However, while very effective, MALDI may possess some limitations, such as lower sensitivity compared to other methods like ESI, particularly with less abundant proteins. The matrix interference can also complicate the interpretation if not handled carefully.
Mass Analyzers
Time-of-Flight (TOF)
The time-of-flight mass analyzer has emerged as an indispensable tool in mass spectrometry. This analyzer determines the mass-to-charge ratio by measuring the time it takes for ions to travel a specific distance. In essence, lighter ions traverse faster than heavier ones, enabling the differentiation of molecular weights.
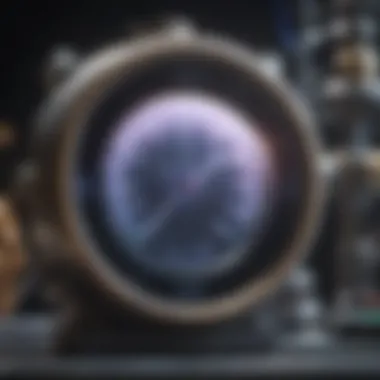
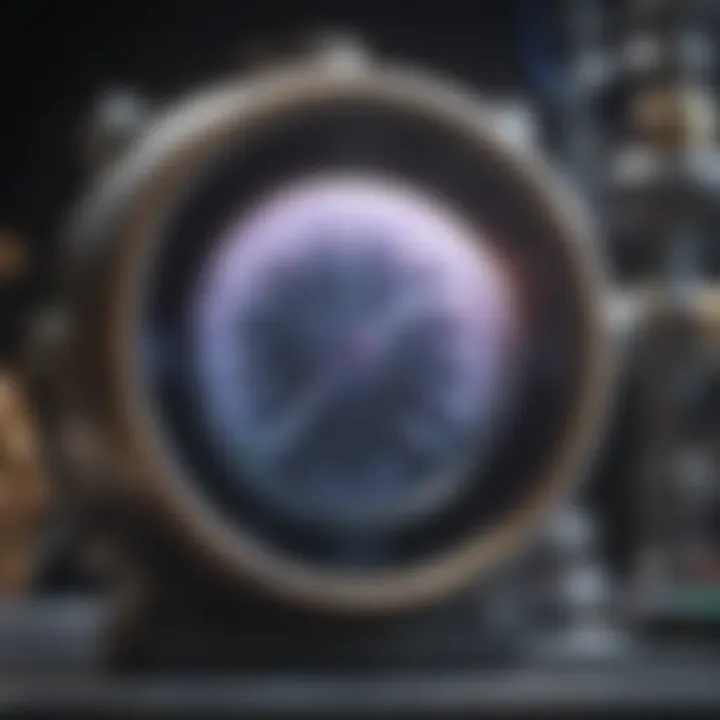
What sets TOF apart is its high-resolution capabilities, making it quite popular for complex biomolecular analyses. One major advantage is its ability to deliver rapid results, essential for high-throughput analysis.
Unlike other analyzers, TOF can analyze a wide range of mass ratios, accommodating small peptides to larger protein complexes.
Nonetheless, TOF systems can be susceptible to variations in spatial and temporal resolution, which may lead to less consistent results among differently sized proteins or ions. The necessity for precise calibration is also a hurdle.
Quadrupole Mass Filter
Quadrupole mass filters offer a different approach by using oscillating electric fields to filter ions based on their mass-to-charge ratio. This scientific technique allows for selective ion monitoring or scanning over a defined mass range, making it versatile in applications from environmental testing to proteomics.
A significant characteristic of this filter is its ability to operate in both tandem mass spectrometry (MS/MS) and do a single analyte scan efficiently. Researchers find this advantageous, as it allows extensive data collection while focusing on specific ions of interest.
On the flip side, quadrupole mass filters can exhibit limitations in resolution and mass range when compared to TOF. Moreover, their complexity can render them less user-friendly, especially for those new to mass spectrometry.
Ion Trap
Ion trap technology aids in capturing ions for sequential analysis, leveraging electric and magnetic fields to store ions in a confined space. This make them quite valuable for qualitative and quantitative applications.
One appealing feature of ion traps is their capacity to accumulate ions, allowing for enhanced sensitivity. This is particularly useful in instances where sample amounts are limited or diluted. Their ability to conduct MS/MS experiments also contributes to revealing structural information about complex biomolecules.
However, ion traps do present certain challenges, such as a lower mass range and the potential for ion instability. Their sensitivity to ion ejection and complex operational parameters can complicate their use in certain applications.
Detectors and Data Acquisition
Detection methods in mass spectrometry trend toward innovative solutions for separating and identifying ions generated from various ionization techniques. Different detectors, such as electron multiplier, Faraday cup, or microchannel plate, each with their own approach, serve the purpose of converting ions into measurable signals.
With high demand for accurate data and sensitivity paired with rapid analyses, this integral part of mass spec instrumentation often sets the stage for how successful a mass spectrum will be. Adopting advanced detectors paves the unique path for mass spectrometry to slice through the fog of complex biological data, allowing researchers to address the intricacies of protein characterization efficiently.
Experimental Setup and Workflow
Understanding the experimental setup and workflow in protein mass spectrometry is vital for achieving accurate, reproducible, and meaningful results. It encompasses several crucial steps that begin the moment a sample is prepared and extends to data collection and analysis. Each component plays a significant role in the overall success of the mass spectrometric analysis, and any slight oversight might skew the results. Thus, having a well-structured and meticulously executed workflow can make all the difference.
Sample Preparation Techniques
Protein Extraction
Protein extraction serves as the foundation for any mass spectrometry analysis. This process involves isolating proteins from biological samples, which might include tissues, cells, or biological fluids. The significance of protein extraction lies in its ability to obtain high-purity samples, ensuring that contaminants do not mislead analysis.
One key characteristic of protein extraction methods is their diversity. Techniques like sonication or solvent extraction stand out for being popular, offering a balance between efficiency and purity. Choosing the right extraction method is essential; it influences not only the quantity of protein recovered but also the integrity of the sample. Some methods can denature proteins, impacting subsequent analyses.
The unique feature of protein extraction is that it can be tailored to specific types of matrices. For instance, using detergents may be advantageous for membrane proteins. However, the choice of the extraction method is not without drawbacks; some methods might require additional steps, and they may even pose safety hazards. Therefore, understanding the specific requirements of the sample is fundamental to successful protein extraction.
Digesting Proteins
After extraction, the next step is digesting proteins, which prepares them for mass spectrometric analysis. This step often involves the enzymatic cleavage of proteins into smaller peptides. The importance of digesting proteins cannot be overstated, as it enhances the resolution of mass spectra and allows for easier identification during analysis.
A vital aspect of digestion is the use of enzymes like trypsin, which hydrolyzes proteins at specific amino acid sites. This specificity yields predictable peptide sizes, thereby simplifying the interpretation of complex spectra. Given that mass spectrometry typically analyzes peptides rather than whole proteins, this step is indeed a beneficial one.
However, digesting proteins has its unique challenges. For instance, incomplete digestion can lead to peptide heterogeneity, complicating data analysis. Additionally, the enzyme's activity could be affected by the sample environment or the presence of inhibitors. Thus, one must calibrate these conditions carefully to obtain the most constructive results.
Instrument Calibration and Maintenance
Calibrating and maintaining mass spectrometry instruments are critical to ensuring accuracy and reliability in measurements. Regular calibration reduces systematic errors, allowing for the consistent quantification of proteins. Moreover, adhering to a stringent maintenance schedule can prolong the lifespan of the equipment and prevent costly downtimes. A well-maintained instrument yields high-quality data, leading to more actionable insights.
Data Analysis Strategies
With the experimental setup in place, data analysis strategies come into play. They enable researchers to extract significant information from complex datasets.
Deconvolution of Mass Spectra
Deconvolution of mass spectra is a mathematical technique used to interpret the overlapping signals in mass spectrometry data. This process allows researchers to disentangle complex mixtures of proteins or peptides, providing a clearer picture of the sample composition. It significantly contributes to the goal of identifying and quantifying proteins accurately.
The key characteristic of deconvolution is its ability to enhance resolution, which becomes critical in highly complex biological systems. Using algorithms to separate overlapping peaks can reveal insights that might otherwise go unnoticed. Thus, this technique is undeniably a popular choice among researchers looking to optimize their analysis.
While deconvolution offers many advantages, it also requires proficient analysis and computational resources. The accuracy of the algorithms heavily depends on the quality of the input data; if the raw spectra are of poor quality, the results will equally suffer. Therefore, proper sample preparation and instrument calibration remain crucial.
Quantitative Analysis
Quantitative analysis provides vital insights into protein levels within biological specimens. This process involves measuring concentrations and understanding variations between samples. It allows researchers to compare protein expressions under different conditions or treatments, thus contributing to the overall understanding of biological processes.
Quantitative analysis is beneficial because it renders comparisons possible between different samples, thereby illuminating changes in biological states. Notably, methods such as relative quantification or absolute quantification can be employed, giving flexibility depending on research goals.
However, the unique feature here is that quantitative results are influenced by numerous factors, such as sample preparation, instrumental settings, and even data analysis protocols. Thus, great care must be exercised during every stage to ensure the integrity of quantification. Missteps can lead to variance that complicates interpretations, potentially leading researchers down the wrong path.
In summary, a robust experimental setup and workflow alongside effective data analysis strategies are the backbone of successful protein mass spectrometry research. They enable researchers to navigate through complex biological data, ultimately advancing our understanding of molecular biology.
In summary, a robust experimental setup and workflow alongside effective data analysis strategies are the backbone of successful protein mass spectrometry research. They enable researchers to navigate through complex biological data, ultimately advancing our understanding of molecular biology.
Applications in Biological Research
The realm of biological research benefits tremendously from the intricate methodologies employed in protein mass spectrometry. This powerful technique serves as a cornerstone in various scientific inquiries, offering a bridge between basic biology and its applications in health and disease. The versatility of protein mass spectrometry allows it to aid in understanding complex biological systems, paving the way for innovations that can alter the course of medical research.
Proteomics and Protein Identification
In the evolving field of proteomics, protein mass spectrometry is often regarded as the linchpin. It enables scientists to identify proteins in complex mixtures, such as tissue samples or cell lysates, with remarkable precision. The ability to dissect the proteome—the complete set of proteins expressed by a genome—brings forth insights into biochemical pathways and cellular functions.
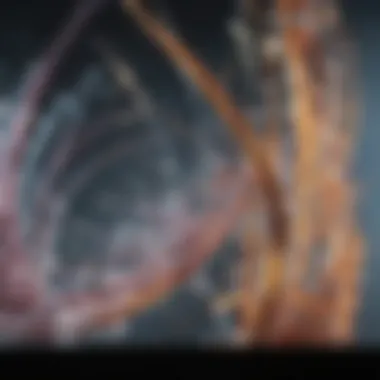
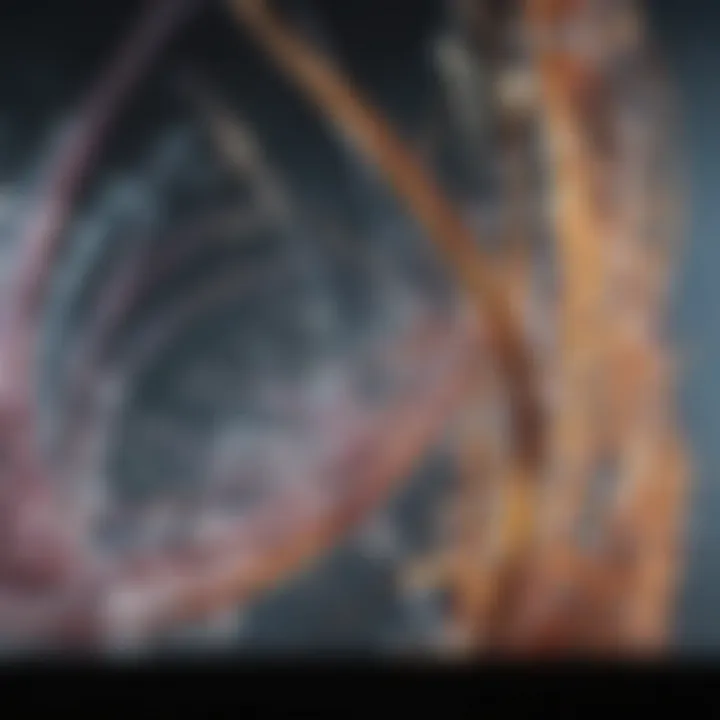
Here are some notable aspects of using mass spectrometry in proteomics:
- High-throughput analysis: It allows for simultaneous identification and quantification of thousands of proteins in a single experiment.
- Sensitive detection: Even minute amounts of proteins can be analyzed effectively, thus unveiling biomarkers related to various diseases.
- Dynamic range: The method exhibits an extensive dynamic range, accommodating proteins expressed at vastly different levels in the sample.
By applying such robust techniques, researchers can map out protein interactions and networks that are essential for cellular function. This understanding is crucial for drug design and therapeutic strategies, especially in fields like oncology and neurology.
Post-translational Modifications Analysis
Another invaluable application of protein mass spectrometry lies in its capacity to analyze post-translational modifications (PTMs). These modifications, which occur after protein synthesis, can significantly influence a protein's function, activity, and localization.
For example, phosphorylation is a common PTM that plays a vital role in signal transduction. Mass spectrometry helps to identify specific phosphorylation sites on proteins, thus unraveling the dynamics of cellular signaling pathways. Other types of modifications, such as glycosylation, ubiquitination, and acetylation, can also be meticulously studied with this technique.
Some key benefits of analyzing PTMs include:
- Understanding regulatory mechanisms: Insight into how PTMs regulate protein functions can illuminate underlying mechanisms of diseases.
- Target identification: Discovering how modifications affect drug targets can lead to more precise pharmaceutical interventions.
- Biomarker discovery: Certain PTMs are associated with specific diseases, allowing mass spectrometry to aid in developing diagnostic tools.
Structural Biology and Interaction Studies
Mass spectrometry plays an equally important role in structural biology and interaction studies of proteins. These studies are fundamental in unraveling the three-dimensional configurations of proteins, which determine their functionalities.
For instance, when proteins interact, understanding their binding sites and affinities becomes critical. Mass spectrometry can aid in probing these interactions under various conditions, thus offering insights that can be crucial for drug discovery.
- Mapping interactions: Techniques such as crosslinking mass spectrometry can provide detailed maps of protein-protein interactions.
- Studying complexes: The formation of larger protein complexes and their conformational changes can also be examined.
- Characterizing stability: By analyzing the stability of protein folds, insights into folding diseases, such as Alzheimer's, can be achieved.
In summary, the applications of protein mass spectrometry in biological research are extensive. From proteomics to the study of post-translational modifications and structural interactions, this technique is vital for advancing our understanding of biology and medicine. It continues to lead the charge in revealing intricate details that lay the groundwork for therapeutic advancements and innovative research.
Advancements in Mass Spectrometry Technologies
Advancements in mass spectrometry technologies have reshaped the landscape of biochemical research. With an ever-increasing focus on precision and accuracy, these developments bring a plethora of knowledge to the table. Emphasizing high-throughput applications and real-time analysis, these revolutionary techniques allow researchers to tackle complex biological questions that were once considered out of reach.
These technological enhancements open the door to a more nuanced understanding of protein structures and functions, with implications spanning proteomics, drug discovery, and personalized medicine.
In the following subsections, we will pinpoint various facets of these advancements, shedding light on what they mean for the scientific community.
Emerging Techniques in Ionization
The area of ionization techniques in mass spectrometry is rapidly evolving, leading to the development of more efficient and effective methods for ion generation. In particular, newer strategies—like desorption electrospray ionization and sonic spray ionization—are proving to be game-changers. By providing sensitive detection and improved resolution, researchers can analyze even the smallest quantities of biomolecules.
This is especially crucial when dealing with biomolecules that are fragile or non-volatile, as traditional methods may fail to provide sufficient insights.
Benefits of Emerging Ionization Techniques:
- Increased Sensitivity: The latest techniques facilitate the detection of proteins at low concentrations, making it possible to study rare proteins or those present in small amounts.
- Diverse Applications: These developments cater to a range of samples, from complex mixtures to single-molecule studies.
- Reduced Sample Preparation: The need for extensive sample preparation is minimized, which can save time and resources.
High-Resolution Mass Spectrometry
High-resolution mass spectrometry takes precision to an entirely new level. It allows for the distinction of ions that are very close in mass, enabling the identification of complex mixtures with precision. This characteristic is especially valuable when analyzing isobaric compounds or distinguishing between post-translational modifications.
In high-resolution setups, various mass analyzers like Orbitraps and FT-ICRs are frequently utilized due to their exceptional performance.
"High-resolution mass spectrometry is like finding a needle in a haystack, but ensuring you can see every individual straw."
"High-resolution mass spectrometry is like finding a needle in a haystack, but ensuring you can see every individual straw."
Considerations in High-Resolution Mass Spectrometry:
- Cost: High-resolution instruments can be significantly more expensive than their lower-resolution counterparts.
- Training Requirements: Expertise is crucial to interpret the detailed data these instruments generate, requiring a skilled workforce.
- Data Volume: The sheer amount of data produced can be overwhelming, necessitating advanced data analysis techniques and strategies.
Integration with Other Analytical Methods
Mass spectrometry doesn’t operate in a vacuum; it can be enhanced by collaborating with other analytical techniques. Methods like chromatography, nuclear magnetic resonance (NMR), and X-ray crystallography complement mass spectrometry by providing additional layers of information about molecular structures and interactions.
Integrating mass spectrometry with techniques such as liquid chromatography (LC-MS) leads to significant improvements in data quality and throughput. For instance, coupling mass spectrometry with high-performance liquid chromatography (HPLC) allows for the separation and identification of complex mixtures, further enriching the analytical capacity available to researchers.
Benefits of Integration Include:
- Comprehensive Analysis: Capturing qualitative and quantitative data simultaneously.
- Increased Efficiency: Streamlining workflows, saving time and resources.
- Improved Data Interpretation: Offering clearer insights into biological processes and mechanisms.
Overall, advancements in mass spectrometry technologies represent a pivotal evolution in scientific research, pushing our understanding of biochemistry to exciting new heights.
Case Studies in Protein Mass Spectrometry
In the ever-evolving field of biochemistry, case studies in protein mass spectrometry serve as trails leading to a deeper understanding of its practical applications. They highlight how this sophisticated technology is leveraged to solve real-world problems, be it in medical research or drug discovery. Each case study not only illustrates a groundbreaking advancement but also brings to light the nuances and challenges of employing mass spectrometry in various contexts. This section underscores the importance of these studies by dissecting their core contributions to our scientific landscape.
Innovations in Drug Discovery
The realm of drug discovery has faced myriad challenges, from identifying viable drug targets to developing effective therapeutic agents. Utilizing protein mass spectrometry has proven to be an invaluable tool in this quest. For example, using mass spectrometry, scientists are able to analyze the proteomic landscape of disease states. This means they can pinpoint specific proteins that either contribute to or are affected by various conditions.
"With protein mass spectrometry, researchers can directly observe the interactions between drugs and their targets, shedding light on mechanisms previously obscured from view."
"With protein mass spectrometry, researchers can directly observe the interactions between drugs and their targets, shedding light on mechanisms previously obscured from view."
One notable case is the development of kinase inhibitors—medications that target enzymes influencing cell growth and proliferation. Through mass spectrometry, researchers can scrutinize the phosphorylation states of proteins and how these are altered in cancerous cells, leading to innovative treatment options.
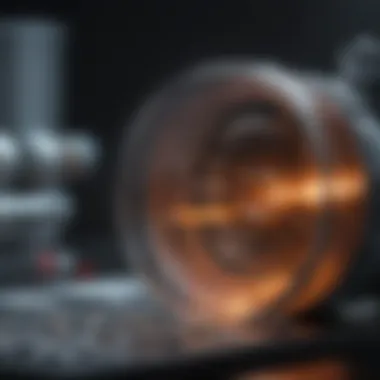
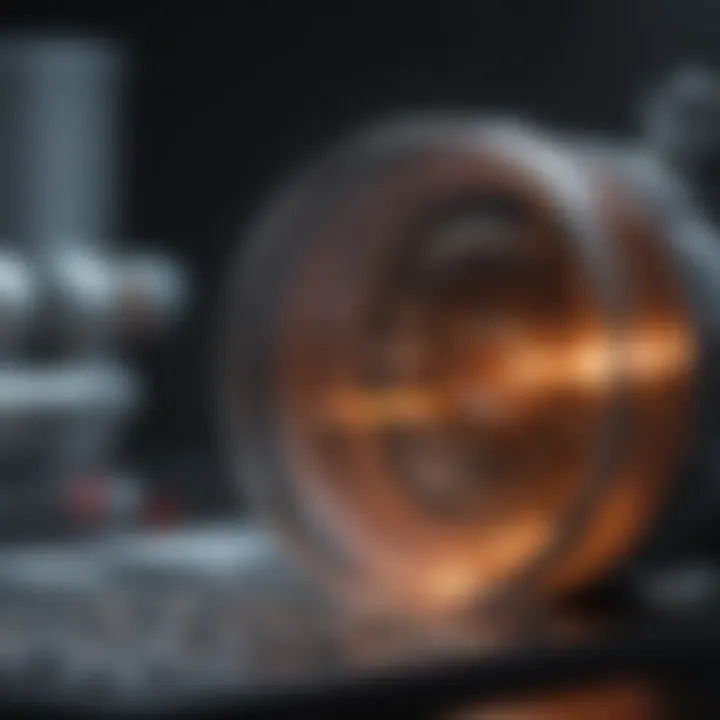
Additionally, the identification of biomarkers through mass spectrometry facilitates early diagnosis and targeted therapy, ensuring that patients receive more personalized treatments. Such developments mark significant progress and underscore the relationship between technology advances and tangible outcomes in healthcare.
Unlocking Disease Mechanisms
Deciphering the underlying mechanisms of diseases often feels like navigating a labyrinth. Yet, mass spectrometry has emerged as a guiding light in this regard. By analyzing protein-protein interactions and post-translational modifications, scientists can gain insights into cellular pathways that trigger diseases.
For instance, in neurodegenerative diseases like Alzheimer's, researchers utilize mass spectrometry to investigate amyloid-beta protein aggregates. This technique enables them to determine how these proteins misfold and accumulate in brain tissue, contributing to neuronal damage.
This approach allows for the discovery of potential therapeutic targets that were once hidden in the fog of scientific uncertainty. The ability to visualize dynamic protein interactions significantly enhances our understanding and provides a robust foundation for developing novel treatments.
Personalized Medicine Applications
Personalized medicine stands at the forefront of modern healthcare, tailoring treatments based on individual patient profiles. Mass spectrometry plays a significant role in this paradigm shift. By analyzing a patient’s proteome—essentially the unique set of proteins present—doctors can design customized therapies that are more effective and carry fewer side effects.
For example, in cancer treatment, mass spectrometry can identify specific mutations in the proteins of tumor cells. By doing so, oncologists can prescribe drugs that specifically target those mutations, thus increasing the chances of treatment success.
Also, mass spectrometry can be used in pharmacogenomics to assess how a patient’s unique genetic makeup affects their response to drugs. By tailoring medication doses accordingly, healthcare providers can avoid adverse reactions and enhance therapeutic efficacy.
This personalized approach not only optimizes patient outcomes but also minimizes the trial-and-error nature of traditional medicine, ultimately creating a more effective healthcare system.
Overall, case studies in protein mass spectrometry demonstrate the profound impact of this technology across various fields. They illustrate not just the capabilities of mass spectrometry but also its role in driving innovations that bridge the gap between research and real-world applications.
Challenges and Limitations
In the realm of protein mass spectrometry, recognizing the challenges and limitations of this potent analytical technique is crucial for comprehending its practical application and enhancement in research. Just as a coin has two sides, protein mass spectrometry reveals a world of possibilities while simultaneously posing certain barriers that analysts must navigate. Challenges can stem from the technical intricacies of the instrumentation used or the inherent complexities of biological samples. An in-depth understanding of these challenges not only aids in improving methodologies but also ensures integrity and accuracy in the results.
Sensitivity and Specificity Issues
Sensitivity and specificity stand as the hallmark indicators of a mass spectrometry method's reliability. Sensitivity refers to a method's ability to detect low-abundance proteins among a vast mixture, while specificity defines how well it distinguishes between similar molecules. Even minor fluctuations in these areas can lead to significant ramifications in data outcomes.
One common issue is the struggle to detect low-abundance proteins, particularly within complex biological systems like plasma or tissue extracts. For instance, imagine trying to locate a needle in a haystack when that needle is merely a whisper in a clamorous crowd. If a protein is key to a certain biological function or disease mechanism, failing to detect it could mean missing out on vital insights or therapeutic targets.
Moreover, false positives can arise when the instrument misidentifies a peptide or protein due to overlapping mass signals. This situation emphasizes the necessity for robust analytical validation and the adoption of meticulously refined protocols to enhance both sensitivity and specificity in mass spectrometry.
"A spectrum is not just a data set; it is the voice of the molecule - we must listen carefully."
"A spectrum is not just a data set; it is the voice of the molecule - we must listen carefully."
Data Complexity and Interpretation
As we traverse deeper into the realm of protein mass spectrometry, the complexity of data emerges as yet another formidable hurdle. The analytical outputs from mass spectrometers can be daunting. A standard mass spectrum often generates mountains of data, each peak representing a potential protein or peptide. However, this deluge of information does not come without its own set of headaches.
Interpreting this information demands not only expertise in mathematical models but also an in-depth knowledge of biology. Misinterpretation can easily occur if a researcher lacks proper training or familiarity with the method. For example, a researcher may misclassify a proteomic signature associated with one disease as a marker for another if they do not capture the context of how these proteins interact.
Furthermore, the integration of mass spectrometry data with other platforms poses additional complications, such as the melding of genomics and proteomics. While this integration is vital for comprehensive analyses, it increases the pathways for potential errors while also multiplying the analytic work.
Amidst these hurdles, the field is continually evolving. Researchers are implementing advanced software solutions to facilitate more accurate data interpretation and foster better communication between diverse analytical approaches. It’s an ongoing battle to balance data depth with clarity, and the merging of data types will likely continue shaping the future landscape of protein mass spectrometry.
Future Directions and Perspectives
As the landscape of protein mass spectrometry evolves, it's imperative to explore the future directions of this pivotal analytical tool in biochemistry. Not only does an understanding of prospective trends clave into the continuous improvement of techniques and applications, but it also equips researchers and industry professionals with insights necessary to navigate the murky waters of scientific advancements. Here, we delve into significant elements, benefits, and vital considerations that should be kept in mind as this field advances.
Trends in Mass Spectrometry Research
In recent years, several trends have emerged that suggest where mass spectrometry may he heading in the next decade:
- Increased Automation: Automation of the mass spectrometry processes is on the rise, leading to higher throughput and reduced human error in protein characterization. This can be pivotal in clinical settings where rapid analysis is crucial.
- Miniaturization of Instruments: With the push for portability and ease of use, lighter, smaller devices that maintain high sensitivity and accuracy are becoming more common. This opens new avenues for in-field testing and diagnostics.
- Data-Intensive Approaches: The integration of big data analytics has become a game-changer. Researchers are now utilizing machine learning algorithms to handle vast datasets generated by mass spectrometry. This can unlock deeper insights into protein interactions and functions that may have gone unnoticed eating into traditional analysis timelines.
"The marriage of mass spectrometry and intelligent analytics represents a paradigm shift in how we approach protein research."
— Informed Analyst
"The marriage of mass spectrometry and intelligent analytics represents a paradigm shift in how we approach protein research."
— Informed Analyst
These trends promise to enhance the capabilities of protein mass spectrometry, promoting diverse applications across research and clinical fields.
Potential for Interdisciplinary Collaborations
Interdisciplinary collaboration stands as a beacon for future prospects in mass spectrometry. The essence of modern research is that often the most profound insights emerge when disciplines intersect. Here are a few aspects highlighting this potential:
- Biotechnology and Bioinformatics: By aligning mass spectrometry with computational studies, researchers can better interpret mass spectra while correlating protein data with genomic information.
- Clinical Applications and Pharmaceutical Development: As personalized medicine gains traction, collaborations between mass spectrometrists and clinicians could optimize drug formulations based on individual protein profiles, thereby enhancing efficacy.
- Environmental Sciences: Application of mass spectrometry in studying environmental samples can result in novel biomarker discovery for pollutants, allowing for improved assessments of public health impacts.
The potential for interdisciplinary collaborations presents pathways that could redefine the applicability of mass spectrometry. It’s not merely about analyzing proteins anymore; it's also about how these analyses can sculpt our understanding across varied scientific realms.
Culmination
Concluding our exploration into the world of protein mass spectrometry, it’s clear this technique stands as a cornerstone of modern biochemistry. The depth of insights gained from mass spectrometry extends far beyond mere identification; it’s a gateway to understanding the very fabric of biological processes. From the nuances of protein interactions to elucidating post-translational modifications, protein mass spectrometry opens a window into the complexity of cellular machinery.
Summary of Key Insights
Summarizing our journey, we’ve traversed through varied aspects of protein mass spectrometry:
- Historical Significance: Initiating with its roots, the evolution of mass spectrometry showcases a progression that mirrors advancements in technology and scientific inquiry.
- Fundamental Principles: We delved into the essential techniques of ionization and the diverse range of mass analyzers that shape the outcomes of experiments. Understanding these fundamentals equips researchers to get the most out of their analyses.
- Diverse Applications: The application in proteomics, drug discovery, and the understanding of diseases underlines its unparalleled versatility. Mass spectrometry isn’t only a technique; it’s a bridge connecting theoretical research with practical advancements in health and industry.
- Advancements and Future Directions: Innovations in the realm of mass spectrometry continue to push the boundaries. There’s an ongoing dialogue about integrating mass spectrometry with other analytical platforms—this could lead to holistic insights that drive scientific knowledge further.
The Role of Mass Spectrometry in Advancing Science
Mass spectrometry is more than just a tool; it embodies a critical turning point in scientific advancement. As we’ve seen throughout this article, the precision and depth it offers in understanding biomolecules is unmatched.
The role of mass spectrometry in advancing science includes:
- Enhanced Experimental Accuracy: By providing exact molecular weights, scientists are armed with precise data that influence hypotheses and experimental designs.
- Bridging Disciplines: The crossover into fields like personalized medicine and systems biology emphasizes its role in interdisciplinary research, as scientists from varied backgrounds converge to solve complex biological puzzles.
- Innovative Therapeutic Approaches: With the insights gained, the research paves the way for novel drug development strategies that are tailored to individual patient profiles, leading to better health outcomes and minimizing side effects.
"In a world where scientific inquiry is paramount, mass spectrometry stands as key to unlocking the potential of biological research."
In essence, protein mass spectrometry is fundamental to propelling not just biochemistry, but the broader spectrum of scientific understanding. As we move forward, the insights from this technique will play a critical role in addressing pressing biomedical challenges, shaping the future of healthcare, and refining our understanding of life at a molecular level.
"In a world where scientific inquiry is paramount, mass spectrometry stands as key to unlocking the potential of biological research."
In essence, protein mass spectrometry is fundamental to propelling not just biochemistry, but the broader spectrum of scientific understanding. As we move forward, the insights from this technique will play a critical role in addressing pressing biomedical challenges, shaping the future of healthcare, and refining our understanding of life at a molecular level.