Advancing Chemistry and Materials Science Through Software
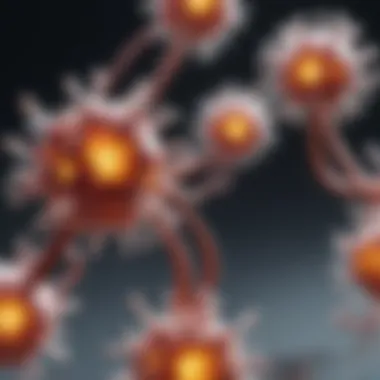
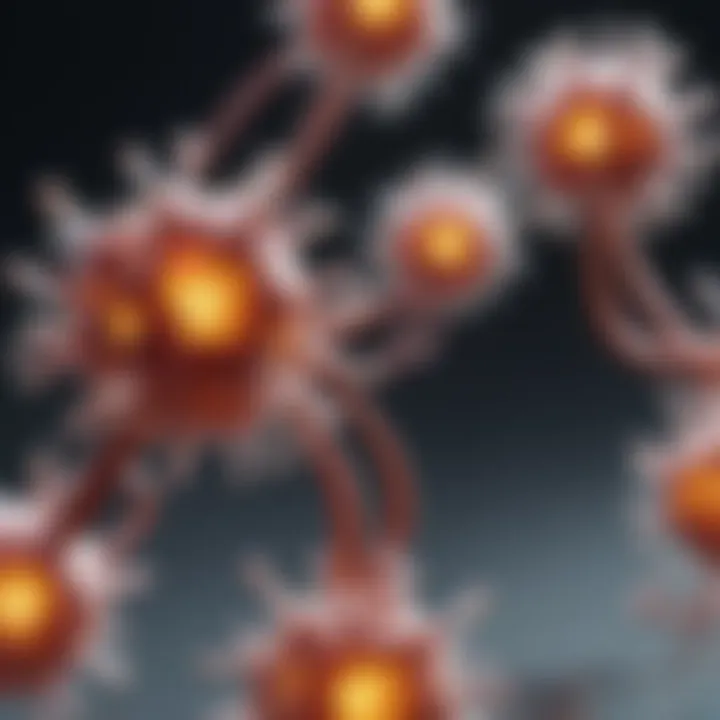
Article Overview
Purpose of the Article
The fusion of software with chemistry and materials science is not just a trend but a fundamental shift in how research is conducted in these fields. This article serves to highlight this intersection, underscoring how innovative software tools drive research capabilities and enhance our understanding of chemical phenomena. Our primary aim is to illustrate the pivotal role that computational methods play in experimental design, data analysis, and the predictive modeling of materials. Recognizing this relationship helps researchers draw connections between software capabilities and tangible advancements in science.
Relevance to Multiple Disciplines
The implications of this relationship stretch far beyond just chemistry and materials science. It touches on fields like physics, biology, and engineering, showcasing a multi-disciplinary approach to problem-solving.
- Chemistry: Software provides tools for molecular modeling, enabling chemists to predict molecular behavior before conducting experiments.
- Materials Science: Advanced data analytics transform how materials are designed, helping scientists focus on material properties that are advantageous for specific applications.
- Biology and Medicine: Tools developed for chemical analysis can lead to breakthroughs in drug discovery, showcasing chemical interactions that enhance therapeutic effects.
- Environmental Science: Software is instrumental in analyzing complex data sets which can inform sustainable practices and material life cycles.
Research Background
Historical Context
The use of software in chemistry isn't a novel concept, yet its evolution has been remarkable. It dates back to the advent of computational chemistry in the 20th century. Early efforts primarily focused on quantum mechanics to model atomic interactions, providing researchers with insights that were previously unattainable. As computers became more powerful, researchers could tackle larger systems and more complex problems. The last couple of decades have witnessed an explosion of software solutions, ranging from databases that catalog material properties to simulation tools that predict chemical reactions with unprecedented accuracy.
Key Concepts and Definitions
To fully grasp the role of software in these sciences, it's essential to define a few core concepts:
- Molecular Modeling: This involves computational techniques to represent the structure and behavior of molecules. It is crucial for predicting how compounds will interact.
- Materials Informatics: This emerging field uses data-driven approaches to predict the properties of materials. It combines machine learning with materials science to drive the discovery of new materials.
- Computational Chemistry: A branch of chemistry focused on using computer simulations to aid in solving chemical problems, providing insights that complement traditional laboratory methods.
In summary, the intertwining of software with chemistry and materials science serves as a catalyst for advancements that are both profound and far-reaching. As we delve deeper into specific software applications and case studies, the narrative will unveil how these tools are reshaping the research landscape.
Preface to Software in Chemistry
In the rapidly evolving worlds of chemistry and materials science, software plays a pivotal role in shaping research and innovations. With complex problems requiring diverse approaches, having the right software tools is akin to having a well-stocked toolbox for a craftsman. Software isn’t merely an accessory in this domain; it’s become fundamental—facilitating tasks from molecular modeling to data visualization and even predictive analytics. By enhancing accuracy and efficiency, these tools allow scientists to explore new avenues that would have been prohibitively time-consuming or even impossible without them.
The significance of software spans several dimensions within scientific inquiry: from modeling intricate chemical structures and reactions to managing vast databases of materials properties and experimental results. This connection not only underscores software's role in advancing our understanding of chemical phenomena but also highlights the collaborative nature of modern research, where different disciplines converge through shared software platforms.
In exploring the nuances of software in chemistry, one witnesses how such platforms contribute to significant breakthroughs, addressing challenges with a fresh perspective. Each advancement not only accelerates the research process but also fosters creativity, pushing the boundaries of how we understand materials and their interactions at a fundamental level.
Defining Software in Scientific Contexts
When discussing software in the realm of chemistry, it's crucial to articulate what we mean by the term. At its core, software encompasses a wide variety of programs and applications designed to assist scientists in modeling, analyzing, and visualizing chemical processes. This can range from simple spreadsheet programs, which aid in data handling, to complex simulation tools like Gaussian or VASP, which predict molecular behavior under various conditions.
The vibrant field of computational chemistry showcases diverse software types, each tailored to specific challenges. For instance, molecular dynamics software allows researchers to simulate the movements of atoms over time, while quantum chemistry packages enable the exploration of electron distributions. These tools provide an interface between theoretical models and real-world phenomena, fostering a comprehensive understanding of chemical behavior.
Historical Perspective on Computational Chemistry
The journey of computational chemistry is a fascinating tale. It’s a story that began with the rudimentary calculations of physical chemistry and evolved into a sophisticated array of software tools that were once the stuff of science fiction. In the late 1950s, the development of the first general-purpose computers opened the floodgates for researchers. Early pioneers utilized punch cards and rudimentary programs to explore and simulate simple systems, but the limitations were glaring. Simulations could be laborious and often did not yield results.
As technology advanced, so did the capabilities of software. With the advent of quantum computing concepts and increasing computational power by the late 20th century, it became feasible to tackle more complexity. The transformation from mere calculation tools to integrated models for exploring chemical phenomena was revolutionary. The transition enabled scientists to predict reaction outcomes, explore new materials, or even design drugs with precision.
Now, in 2023, computational chemistry and materials science are shaped by tools that integrate machine learning and databases on an unprecedented scale. By harnessing massive data sets, researchers can generate insight in real-time, leading to a leap in understanding across various branches of the science. The quest for efficiency and accuracy has never been more pronounced, and it continues to drive innovation in how we approach chemistry.
Categories of Chemistry Software
In the ever-evolving landscape of chemistry and materials science, software serves as an indispensable ally, enhancing research and pushing the boundaries of innovation. Understanding the categories of chemistry software is crucial, as these tools each play a distinct role in facilitating the work of scientists. This not only streamlines workflows but also optimizes the analysis of complex data, ultimately accelerating discoveries.
Software in chemistry can be broadly classified into several categories:
- Molecular Modeling Software: These tools are essential for simulating and visualizing molecular structures and interactions. They allow researchers to predict how molecules behave under various conditions, often saving time and resources during experimental phases.
- Chemical Database Management Systems: These systems organize vast repositories of chemical data, enabling users to retrieve, query, and analyze this information effectively. A well-structured database can significantly enhance research outcomes by providing quick access to relevant data.
- Computational Chemistry Packages: Often considered the backbone of theoretical chemistry, these packages offer robust algorithms for performing complex calculations that are essential to understand molecular phenomena and predict chemical reactions.
- Materials Informatics Tools: By leveraging data-driven methodologies, these tools support the development and characterization of new materials. They help chemists identify optimal conditions for material synthesis and contribute to the design of innovative materials for various applications.
Each category has its benefits and considerations. For instance, molecular modeling software can illustrate potential interactions, but might require robust computational resources. Chemical databases, while providing valuable insights, necessitate ongoing updates to ensure completeness and accuracy. Computational packages tend to require a steep learning curve, yet they enable highly precise calculations. Materials informatics tools promise efficiency but often rely heavily on the quality of input data.
Overall, navigating these categories equips researchers with the right tools to tackle the complexities of their work, making it paramount to select suitable software tailored to specific needs and applications.
"The true power of computational chemistry lies in its ability to transform detailed theoretical insights into practical applications."
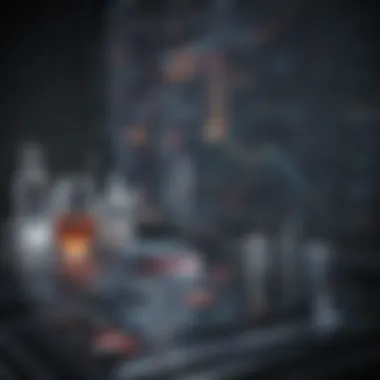
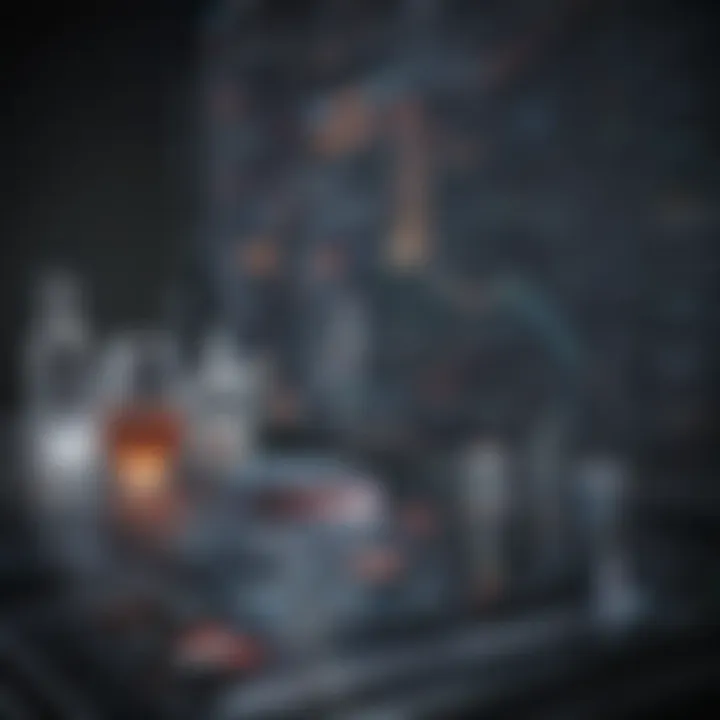
"The true power of computational chemistry lies in its ability to transform detailed theoretical insights into practical applications."
In summary, the categorization of chemistry software underscores its essential role in research and development, offering assorted functionalities that collectively propel advancements in the field.
Impact on Research and Development
The impact of software on research and development within chemistry and materials science is profound. Software tools have become essential in virtually every phase of research, from initial hypothesis generation to experiment design and analysis of results. Their role is not merely supportive; they are often the driving force behind breakthroughs that push the boundaries of scientific inquiry.
By automating complex calculations and simulations, software significantly reduces the time needed to move from concept to application. This accelerated process allows researchers to test ideas quickly, fostering an environment where innovation can thrive. For instance, when exploring new catalysts, a chemist can utilize molecular modeling software to predict how changes to structure affect activity, eliminating many rounds of trial and error in the lab. This not only saves precious time but also conserves resources which might otherwise be wasted on less promising avenues.
Furthermore, software plays a crucial role in data management and analysis, which are foundational to modern research. Sophisticated statistical tools can sift through vast amounts of data to uncover trends and insights that might otherwise remain hidden. Such capabilities are invaluable, particularly in materials science where complex interactions and properties often matter.
Another benefit of software in this realm is its ability to foster collaboration. Tools that support shared databases or cloud computing enable researchers across the globe to access and contribute to collective knowledge bases. This interconnectedness not only bolsters individual projects but also creates opportunities for interdisciplinary partnerships that can lead to groundbreaking discoveries.
To summarize, the integration of software into research and development not only streamlines processes but transforms them, equipping scientists with the tools needed to tackle intricate problems and advance the frontiers of knowledge.
Accelerating Discovery Processes
Software has drastically changed how discoveries are made in chemistry and materials science. One key aspect is the speed with which hypotheses can be tested.
- Simulation Tools: With molecular dynamics simulations, researchers can explore potential behaviors of substances without physical experimentation, greatly reducing the time frame for discovering new compounds. For example, researchers no longer need to synthesize every variant of a polymer. Instead, they can model them on computers, predicting properties and behaviors.
- Data Mining Techniques: Machine learning algorithms analyze historical experimental data to uncover patterns, enabling scientists to refine their searches for new materials or life-saving drugs. By employing these computational techniques, teams can significantly speed up the discovery process, identifying promising candidates rapidly.
The use of data-driven techniques leads not only to faster discoveries but also to more targeted strategies that enhance the quality of research outcomes.
Cross-Disciplinary Applications
Software's adaptability extends well beyond chemistry and materials science, impacting a broad range of disciplines. This cross-disciplinary nature amplifies the benefits derived from computational tools, reinforcing the importance of software in the scientific landscape.
- Bioinformatics: Software used for analyzing biological data leverages chemistry principles to innovate in fields such as drug discovery. For instance, molecular docking software assesses how drug molecules might bind to their targets, which is a crucial step in developing new medications.
- Environmental Modeling: In environmental science, software applications help in predicting chemical behavior in complex systems, such as pollutant dispersion in water bodies. Here, data from various fields come together, illustrating the collaborative potential of software to solve real-world issues.
As the lines between disciplines blur, the role of software as a facilitator of interdisciplinary collaboration will only continue to grow, making it a vital piece of the modern scientific puzzle.
"The future of research isn't just in the lab but in the seamless interplay of software and scientific inquiry."
"The future of research isn't just in the lab but in the seamless interplay of software and scientific inquiry."
Software is indeed positioned as a keystone to navigate the complexities of contemporary science, with its ability to advance research efficiently and effectively.
Data Analysis in Chemistry and Materials
In the modern landscape of chemistry and materials science, data analysis serves as a backbone, enabling scientists to make informed decisions across a multitude of applications. Data isn't just a collection of numbers or a stack of research results; it's the lifeblood that fuels discovery and innovation. The significance of data analysis lies in its ability to extract meaningful insights from complex datasets, which can often be more enigmatic than straightforward. This process can propel researchers towards breakthroughs, whether in drug development or material synthesis, and is integral to shaping experimental designs and strategic planning.
Several specific aspects highlight the value of data analysis in this context:
- Accuracy in Prediction and Modeling: Software tools facilitate advanced statistical techniques that allow for the establishment of models predicting chemical behavior or material properties. This can save time and resources by focusing efforts on more promising avenues.
- Discovery and Validation of Patterns: Analyzing large volumes of data can reveal hidden patterns and relationships that were not immediately apparent. This capacity can lead to novel hypotheses and, subsequently, experimental validation.
- Cross-Disciplinary Integration: Data analysis methods are often borrowed from other fields, such as statistics and computer science. This intermingling can lead to innovative approaches that might not have originated within traditional chemistry.
- Facilitating Collaboration: When data is analyzed and presented effectively, it opens doors for collaboration across disciplines, industries, and geographical locations. This sharing of insights can catalyze advancements that would be impossible in isolated silos.
Statistical Software in Chemistry
Statistical software has become increasingly essential in the world of chemistry, especially as datasets have grown in size and complexity. In practical terms, tools like R and Python libraries enable chemists to apply sophisticated statistical methods to their data. This allows penetration into deeper insights that elementary software simply cannot provide.
Some key benefits include:
- Hypothesis Testing: Statistical analysis aids in determining whether the effects observed in experiments are statistically significant. It can help avoid false conclusions that may arise from apparent trends.
- Data Interpretation: Complex datasets often contain noise; statistical software helps to filter out this noise, allowing researchers to focus on the signal that matters—the true relationships among variables.
- Regression Analysis: Chemists can leverage regression techniques to model relationships between different chemical entities or material properties, which can assist in predictive modeling.
As an illustrative example, a chemist examining the effectiveness of a new drug might use statistical software to analyze trial results, comparing various dosage effects while accounting for variables like age or sex. Such capabilities foster more robust conclusions that guide future research directions.
Visualization Tools for Complex Data
Visual representation of data is often as crucial as the data itself. Visualization tools provide a means of transforming intricate numbers and statistics into digestible forms, enabling stakeholders to grasp findings quickly and intuitively. Tools like Tableau, Python’s Matplotlib or Seaborn libraries, and R’s ggplot2 have reshaped how chemists interpret their results.
Consider the following advantages of visualization tools:
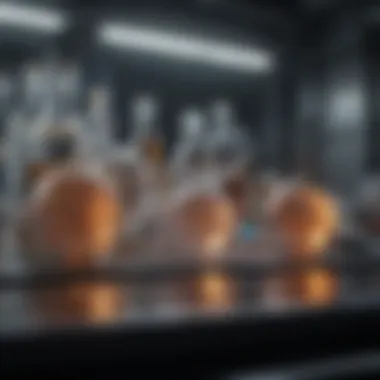
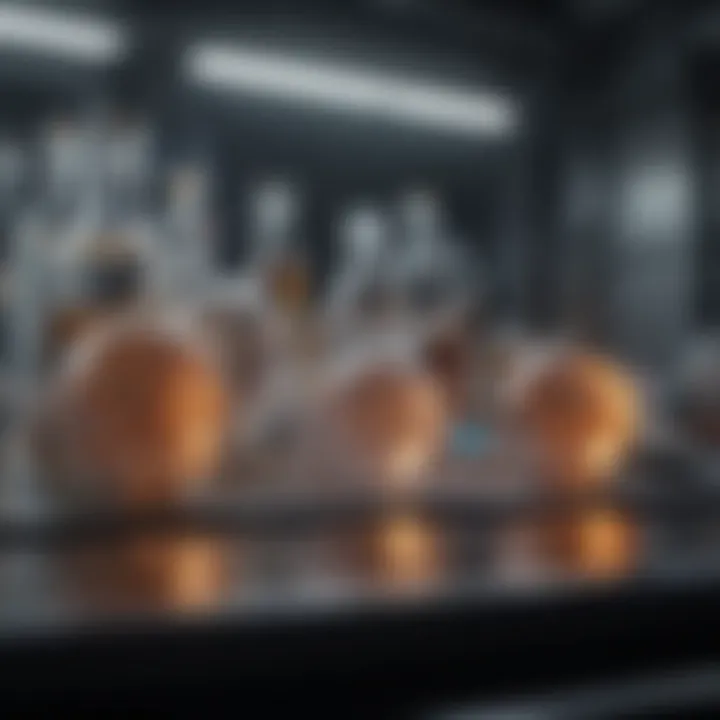
- Clarity and Insight: Graphs, heat maps, and interactive dashboards can expose trends and correlations that raw data might obscure. This visual approach enhances understanding and retention.
- Communication: Visualization serves as a powerful method for presenting findings to both scientific and non-scientific audiences. It demystifies complex information, making it accessible.
- Exploratory Data Analysis (EDA): Before diving deep into data modeling, chemists can use visualization tools to explore relationships, distributions, and anomalies, leading to more informed modeling choices.
For instance, a researcher working on nanomaterials might utilize a 3D scatter plot to demonstrate the relationship between particle size, surface area, and catalytic activity—a visual representation that would resonate more with stakeholders than columns of data.
"Good visualizations are not just supportive—they are essential to understanding complex data shaping the future of research and development."
"Good visualizations are not just supportive—they are essential to understanding complex data shaping the future of research and development."
The combination of statistical analysis and visualization can be a game changer for chemists and materials scientists. Together, they transform data into decisions driving the advancement of science.
Case Studies of Software Applications
Exploring case studies on software applications in chemistry and materials science is like holding a mirror to the innovative techniques that are shaping the future of these fields. These cases not only illustrate how software is utilized to solve real-world problems but also shine a light on the underlying principles driving these advancements. By examining specific instances, researchers and educators can gain insights into the practical implications and benefits of various software tools.
Molecular Dynamics Simulation Successes
Molecular dynamics (MD) simulations have become a pivotal strategy for studying complex molecular systems in real time. They offer a window into the microscopic dance of atoms and molecules as they interact under various conditions. For example, take the case of the development of new drug compounds targeting diseases. MD simulations allow researchers to predict how potential drug molecules will interact with biological targets, saving both time and resources in the early stages of pharmaceutical development.
In one instance, researchers employed MD simulations to investigate the binding efficiency of drug candidates against the proteins associated with COVID-19. By modeling the interactions, they could discern which candidates held the most promise before synthesizing physical samples. This not only expedited the discovery process but also reduced the likelihood of late-stage failures in drug trials.
Another significant success can be found in materials science, where MD simulations are used to understand properties of materials under stress. For instance, simulating the behavior of materials used in aerospace applications has become essential, as these materials need to withstand severe conditions. The simulations provide insights regarding how materials deform and fail, guiding engineers in designing more robust materials. This specific application underscores how MD simulations cling tightly to the realm of practical, impactful research.
Machine Learning in Material Discovery
Machine learning (ML) represents a frontier in the quest for new materials, offering tools that enable researchers to predict material properties and performance based on existing data. Unlike traditional methods, which often require extensive experimentation, ML can analyze vast datasets to identify patterns and correlations. This capability can lead to the discovery of novel materials with desired characteristics more quickly than conventional approaches.
A noteworthy example is the use of ML algorithms in the discovery of thermoelectric materials, which convert heat energy to electricity. Researchers trained algorithms on existing thermoelectric materials to identify trends that correlate structure with performance. This approach not only accelerated the discovery of new thermoelectric materials but also offered recommendations on how to synthesize them.
Moreover, ML assists in optimizing the properties of materials for specific applications. In a case study focused on battery technology, ML was utilized to predict the performance of various electrode materials. As a result, researchers were able to narrow down candidates for high-capacity, fast-charging batteries, which have broad applications in consumer electronics and electric vehicles.
In both cases—whether using MD simulations to model molecular interactions or harnessing ML to identify novel materials—the case studies underscore how these sophisticated software applications can revolutionize chemistry and materials science, enabling breakthrough discoveries that were once seen as far-fetched.
Future Directions for Software in Science
The ongoing evolution of software in chemistry and materials science reveals a landscape full of opportunities and challenges. As technology continues to advance, it becomes increasingly clear that software will play a pivotal role in shaping future scientific endeavors. This section aims to explore key areas where software is likely to influence the future of these fields, focusing on crucial integrations and considerations that will drive progress.
Integration with Artificial Intelligence
Artificial Intelligence (AI) and software are becoming inseparable allies in the quest to unravel complex scientific puzzles. In chemistry and materials science, the integration of AI into software frameworks presents several notable advantages. For one, AI can enhance data analysis capabilities, identifying patterns that are often imperceptible to human researchers. Imagine a chemist working with endless datasets—AI algorithms can discern subtle correlations, guiding research directions that would traditionally take years to explore.
Moreover, machine learning models can predict molecular behavior with remarkable precision. This predictive capability allows scientists to simulate potential outcomes before conducting costly experiments, effectively reducing trial and error. With the help of AI, software platforms can pinpoint the most promising compounds faster than ever.
However, despite its potential, this integration comes with cautions. For instance, the opacity of some AI models raises concerns about reproducibility and transparency in scientific findings. Researchers need software that not only provides accurate predictions but also elucidates the reasoning behind them. This balance is essential to maintain trust in the scientific process.
Open Source vs. Proprietary Tools
The debate between open-source and proprietary software tools continues to be a pertinent issue within scientific communities. Open-source software brings the benefit of collaboration and community-driven advancements. Researchers can freely access, adapt, and improve these tools, leading to rapid innovation and democratization of knowledge. For instance, platforms like Avogadro and ChemCollective have allowed countless scientists to leverage their capabilities without the heavy financial burden of licensing fees.
On the flip side, proprietary software such as ChemDraw or Materials Studio often provide robust features and dedicated support that can significantly streamline research processes. These tools are typically well-optimized, offering a polished user experience that can save time and enhance productivity.
Each option presents valid choices depending on the specific needs of researchers. Considerations regarding budget, usability, and desired features will dictate the path forward. Ultimately, the future of software in science may see a hybrid solution where the strengths of both open-source and proprietary systems can harmoniously coexist.
"The future will require a thoughtful consideration of the tools we choose—collaboration and innovation resulting from both open-source and proprietary solutions could reshape our scientific landscape."
"The future will require a thoughtful consideration of the tools we choose—collaboration and innovation resulting from both open-source and proprietary solutions could reshape our scientific landscape."
Challenges and Limitations
When discussing the impact of software in chemistry and materials science, it's vital to acknowledge the challenges and limitations associated with its use. Just as tools can empower research, they can also present significant hurdles that researchers, educators, and industry professionals must navigate. Understanding these challenges is not merely academic; it's crucial for developing effective strategies to overcome them and maximize the benefits that software offers to the scientific community.
Addressing Data Security and Intellectual Property
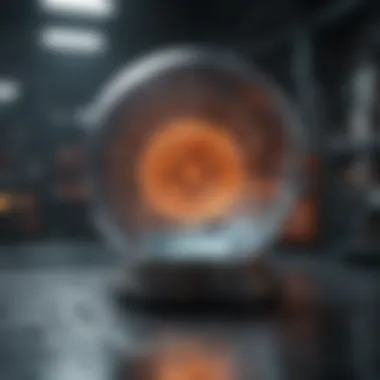
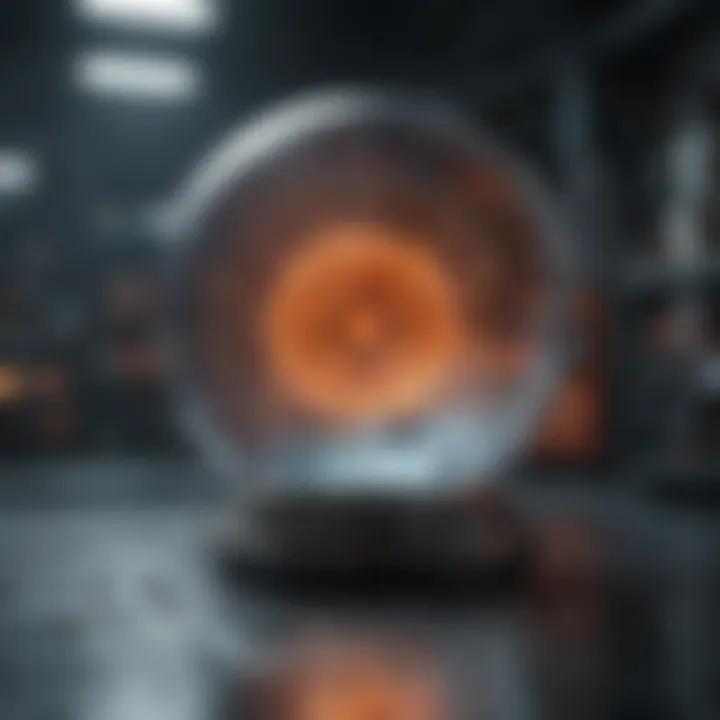
In an era where data drives discovery, the protection of sensitive information and intellectual property has become a cornerstone of research with software tools. Researchers often deal with proprietary datasets, innovative formulas, or experimental results that could be commercially valuable. With the rise of cloud computing and collaborative platforms, the risk of data breaches has increased significantly.
Consider the scenario where a team uses a shared software tool for molecular modeling. While collaboration can enhance scientific progress, it poses a risk that critical proprietary data may inadvertently be exposed to unauthorized individuals. Institutions are tasked with ensuring that robust security protocols exist to safeguard these assets.
To mitigate these issues, several approaches can be considered:
- Data encryption: Implementing strong encryption protocols for data at rest and in transit can help protect sensitive information from unauthorized access.
- Access control: Limiting data access to only those individuals who need it to perform their work can reduce the chances of accidental leaks.
- Regular audits: Conducting frequent assessments of software security measures ensures vulnerabilities are identified and addressed promptly.
By taking these steps, researchers can maintain trust in their digital environments and reinforce the integrity of their findings while developing software-based solutions for complex scientific challenges.
Navigating Software Usability Issues
While sophisticated software has revolutionized the field of chemistry and materials science, its effectiveness can be hampered by usability issues. For many, advanced software comes with a steep learning curve, which can leaad to inefficiencies and even errors in research. Imagine a chemist trying to run simulations on a molecular modeling program but struggling with unintuitive interfaces; the potential for miscommunication and mistakes looms large in such scenarios.
The inclusion of user-friendliness in software design is paramount for ensuring researchers can effectively utilize these tools. Some of the common usability challenges observed include:
- Complexity of interfaces: A cluttered interface can overwhelm users, leading to frustration. Simplifying the design can promote more intuitive use.
- Insufficient training resources: Many researchers find that the training provided doesn't cover all necessary aspects of software use. Comprehensive tutorials and responsive support systems can address this gap.
- Compatibility issues: The diverse range of computing environments complicates the seamless use of software. Ensuring software can easily integrate with existing systems can drastically enhance its adoption.
Thus, addressing usability challenges is not just about improving user experience; it's about enabling researchers to fully harness the capabilities of software tools in their scientific inquiries. By investing in better usability practices, the scientific community can foster a more inclusive environment, encouraging collaboration and innovation.
"In the race of scientific discovery, usability of software should be as critical as the data it processes."
"In the race of scientific discovery, usability of software should be as critical as the data it processes."
Engaging with these challenges effectively will help in realizing the full potential of software in chemistry and materials science.
The Role of Education in Software Proficiency
In the modern landscape of chemistry and materials science, a solid education in software proficiency is not just a luxury—it's a necessity. With advancements linking intricate data analysis and computational modeling, there's a growing realization that today's chemists and materials scientists must be well-versed in these digital tools. This equips them to tackle complex scientific puzzles that were once formidable challenges. The implications stretch beyond mere theoretical knowledge; they influence how future scientists will engage with ongoing research, collaborate across disciplines, and innovate in unexpected ways.
Education systems need to prioritize software instruction that aligns with real-world applications. This is not to say traditional chemical principles should be sidelined; rather, the integration of software skills can enhance understanding and creativity in scientific inquiry. Analytical thinking, problem-solving, and data interpretation are directly tied to software skills. Thus, developing these proficiencies can be transformative for science students.
Curriculum Development for Future Scientists
Designing a curriculum that balances core chemistry concepts with software proficiency is crucial. Educational institutions should consider infusing computational chemistry into their programs. Courses that focus on molecular modeling, chemical databases, and statistical analysis can be integrated into undergraduate and graduate studies. Each module could include hands-on training with software tools that are prominent in the field, like Gaussian for computational chemistry or Python for scripting analyses.
Moreover, partnerships with industry can provide students with project-based learning opportunities, where they can apply software skills to real problems. One idea is to have guest lecturers from companies that utilize these tools in their daily operations, exposing students to the software's practical applications. This approach, where academia meets industry needs, ensures graduates aren’t just theoretical experts but practical whizzes who can hit the ground running.
Public Access and Community Resources
Access to community resources also plays a significant role in enhancing software proficiency. Open-source tools and databases can empower aspiring chemists and materials scientists to explore without the constraints posed by high software licensing fees. Initiatives that promote access to resources like the OpenChemistry project or the Protein Data Bank make it feasible for students from diverse backgrounds to engage with sophisticated software.
Online communities further supplement this learning. Platforms like Reddit and dedicated forums allow users to share tips, troubleshoot problems, and explore advanced techniques collaboratively. By leveraging these resources, learners can take command of their education, fostering a culture of shared knowledge within the scientific community.
In summation, establishing a robust educational framework that prioritizes software skills is fundamental to nurturing the next generation of chemists and materials scientists. With a combination of thoughtfully designed curricula and widespread access to tools, we can foster a workforce primed for innovation.
In summation, establishing a robust educational framework that prioritizes software skills is fundamental to nurturing the next generation of chemists and materials scientists. With a combination of thoughtfully designed curricula and widespread access to tools, we can foster a workforce primed for innovation.
Epilogue
In the realm of chemistry and materials science, the contributions of software are nothing short of transformative. As we close this exploration, it is evident that the integration of computational tools stands at the heart of advancement in these fields. The role of software—whether in data analysis, molecular modeling, or materials informatics—has ushered in efficiencies that are reshaping research processes, fostering collaboration, and accelerating the pace of discovery.
Recapitulating the Importance of Software
Firstly, it bears noting that software is no longer a supplementary tool; it has become indispensable in the scientific toolkit. Researchers now rely heavily on computational software to simulate environments that were previously only conceptual. For instance, molecular modeling software allows chemists to visualize molecular interactions at unprecedented detail, illuminating pathways for new drug discovery and materials innovation. This capacity translates into significant time savings and cost efficiency in the development stages of research.
Secondly, the analytical prowess of software enables scientists to sift through massive datasets, identifying patterns that might go unnoticed in manual analysis. Statistical software packages are pivotal in interpreting experimental results and modeling potential outcomes, ultimately guiding informed decision-making. This, in turn, enhances the repeatability and reliability of scientific research.
"In the fast-paced world of scientific research, the ability to rapidly analyze and interpret data is essential for staying ahead."
"In the fast-paced world of scientific research, the ability to rapidly analyze and interpret data is essential for staying ahead."
Finally, incorporating software into educational curricula prepares future chemists and materials scientists for the demands of modern scientific inquiry. In classrooms and laboratories, students learn to wield these powerful tools, equipping them with competence that is increasingly vital in academic and industry settings. This emphasizes the cyclical nature of software advancements—whereby education drives innovation, which loops back into academia and industry.
Vision for the Future of Chemistry and Materials Software
Looking forward, we must acknowledge the ongoing evolution of software technology and its implications for chemistry and materials science. One significant direction is the strengthening of software's synergy with artificial intelligence. By harnessing machine learning algorithms, we can bolster predictive modeling capabilities, identifying potential materials or reactions that may have previously eluded researchers. This intersection promises not only faster discoveries but also groundbreaking solutions to pressing global challenges.
Moreover, as software development continues to grow, the debate on open source versus proprietary tools becomes ever more critical. Open-source software facilitates wider accessibility and collaborative improvement, which is essential for a community-driven approach to scientific discovery. However, proprietary applications often provide robust, specialized tools that can offer unique functionalities. Striking the right balance will be paramount as we navigate the future landscape of chemistry and materials software.