Advanced Techniques in Bacterial DNA Extraction
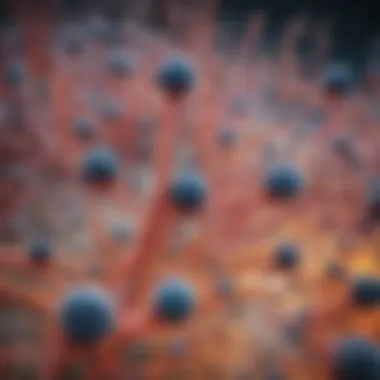
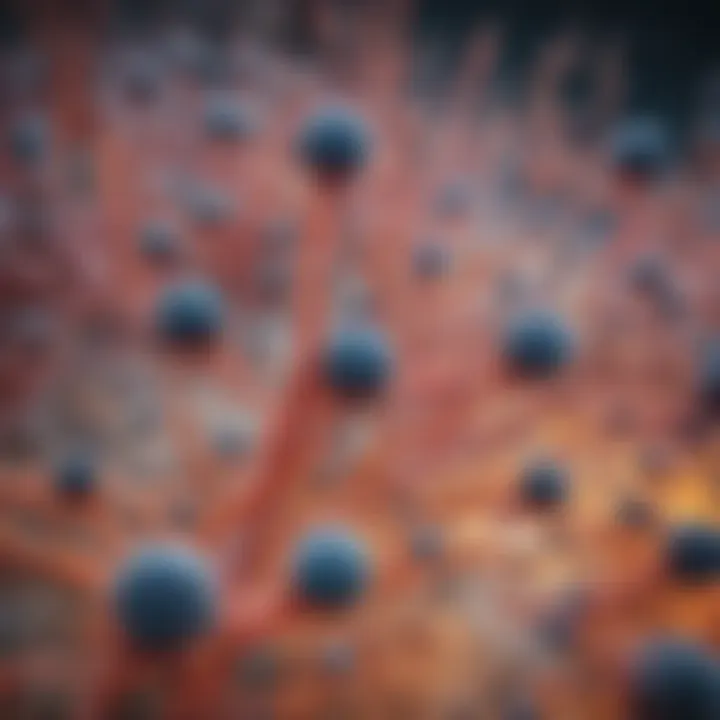
Article Overview
Purpose of the Article
The primary aim of this article is to elucidate the various methodologies involved in the extraction of genomic DNA from bacterial sources. The focus lies on the precision required during isolation, ensuring that the subsequent analyses yield reliable and accurate results. Researchers engage with these techniques to optimize their approaches, enhancing both the quality and the reproducibility of their data.
Relevance to Multiple Disciplines
Genomic DNA extraction is a crucial process in several fields such as microbiology, genetics, molecular biology, and biotechnology. In microbiology, understanding bacterial genomes contributes to antibiotic resistance research. In genetic studies, DNA extracted from bacteria can be pivotal for understanding genetic variations and evolutionary biology. Moreover, applications in biotechnology, like the production of recombinant proteins, rely heavily on these techniques. Therefore, the insights shared in this guide are pertinent across diverse disciplines, making it a valuable resource for various stakeholders, including researchers and educators.
Research Background
Historical Context
The processes of genomic DNA extraction have evolved significantly over the decades. Initially, methods were rudimentary, often relying on mechanical disruption. However, advances in molecular biology have led to the development of specialized techniques that improve yield and purity. Key breakthroughs in the understanding of cellular structures and biological molecules have propelled these methods to become more efficient and standardized.
Key Concepts and Definitions
Understanding some fundamental concepts can enhance the reader's comprehension of DNA extraction techniques:
- Genomic DNA: This refers to the complete set of DNA, including all of its genes, present in an organism.
- Lysis: The process of breaking down the cell wall or membrane to release cellular contents.
- Purification: Steps taken to isolate DNA from other cellular materials and contaminants.
These concepts provide a foundational knowledge base for further exploration into the methodologies and challenges associated with genomic DNA extraction from bacteria.
"Precision in isolating genetic material is crucial for accurate downstream applications in genomics and biotechnology."
"Precision in isolating genetic material is crucial for accurate downstream applications in genomics and biotechnology."
Understanding the fundamentals of genomic DNA extraction will empower researchers to adopt best practices and methodological rigor as they pursue their scientific inquiries.
Prelude to Genomic DNA Extraction
Genomic DNA extraction from bacteria is a pivotal process in various fields, including microbiology, biotechnology, and genetic research. It involves isolating DNA from bacterial cells to enable further analysis and experimentation. Understanding the methods and considerations involved in this extraction can significantly affect the quality of the genomic DNA, which in turn influences downstream applications such as cloning, sequencing, and synthetic biology.
The importance of DNA extraction cannot be understated. High-quality DNA is essential for the success of molecular techniques. These techniques are often sensitive and require pure DNA free from contaminants. Extracting DNA with precision allows researchers to obtain reliable results, which can lead to significant discoveries in understanding biological processes and developing new technologies. Furthermore, genomic DNA extraction is fundamental for bioinformatics analyses, which rely on accurate data for modeling and prediction purposes.
Researchers must be cognizant of the specific characteristics of bacterial cells to optimize their extraction protocols. These cells possess unique structures, such as the cell wall, which can impede the extraction process. The complexity of the bacterial genome also requires tailored approaches to yield high-quality DNA. Thus, scientists must critically evaluate their extraction techniques and understand the nuances associated with different bacterial species.
Bacterial Cell Structure and Its Implications
Understanding bacterial cell structure is foundational in genomic DNA extraction. Each part of a bacterial cell plays a role in how DNA can be accessed and isolated. Comprehending this structure helps optimize extraction techniques, making them more efficient and effective.
Bacteria come in various shapes, sizes, and structures, but all share certain features. Notably, the composition of the cell wall and the characteristics of the cytoplasmic membrane are critical. These features influence the choice of extraction methods, as they determine how easily the cellular components can be disrupted.
Cell Wall Composition
The cell wall is a unique structure in bacteria, primarily made of peptidoglycan in many species. This material gives the cell wall its strength and shape. There are two main types of bacterial cell walls: Gram-positive and Gram-negative.
- Gram-positive bacteria have thick layers of peptidoglycan. This structure can retain the crystal violet stain used in Gram staining, thus appearing purple under a microscope.
- Gram-negative bacteria, on the other hand, have a comparatively thin peptidoglycan layer and an outer membrane composed of lipopolysaccharides. These bacteria do not retain the crystal violet stain but take on a pink color after counterstaining with safranin.
The cell wall composition affects the efficiency of cell lysis methods. Chemical methods may require additional steps to breach the outer membrane of Gram-negative bacteria. In contrast, physical methods can more effectively disrupt Gram-positive bacteria due to their robust cell wall.
Cytoplasmic Membrane Characteristics
The cytoplasmic membrane, also known as the plasma membrane, is crucial for maintaining the integrity of the cell. It is a phospholipid bilayer that regulates the transport of substances in and out of the cell. This membrane also contains proteins that can facilitate various functions, including signaling and nutrient transport.
The characteristics of the cytoplasmic membrane influence the choice of lysis and extraction protocols. Some techniques may rely on disrupting the membrane to release DNA, while others might use enzymatic treatments that target specific membrane components.
In summary, recognizing the significance of bacterial cell structure is vital for any genomic DNA extraction initiative. The distinction between Gram-positive and Gram-negative bacteria and understanding the role of the cytoplasmic membrane provides essential insights. These elements impact the choice of extraction methodology, thus ensuring greater success when isolating bacterial DNA for further analysis.
Common Techniques for DNA Extraction
In the field of molecular biology, the extraction of genomic DNA from bacteria forms the foundation for numerous research and diagnostic applications. It is crucial to select an appropriate technique for DNA extraction, as this will greatly influence the quality and quantity of the extracted DNA. Each method comes with unique benefits and challenges, influenced by the specific type of bacterial cells and the intended downstream applications. This section outlines the main techniques—chemical lysis methods, physical disruption techniques, and enzymatic digestion approaches—used to effectively isolate DNA from bacterial samples.
Chemical Lysis Methods
Chemical lysis methods involve the use of detergents or lytic agents to disrupt the cellular membranes of bacteria. This process allows for the release of DNA into the solution. Commonly used agents include sodium dodecyl sulfate (SDS) and cetyltrimethylammonium bromide (CTAB). The advantages of chemical lysis are numerous. They are typically more straightforward to implement and can be optimized for various bacteria types. For instance, SDS is often preferred for Gram-negative bacteria as it solubilizes membrane proteins and helps to disrupt the outer membrane.
However, one must consider the specific cell wall composition of the bacteria in question. Not all chemical substances will yield favorable results. Moreover, residual chemicals can affect the purity of the extracted DNA and influence subsequent experimental results. Therefore, careful selection of chemicals is paramount.
Physical Disruption Techniques
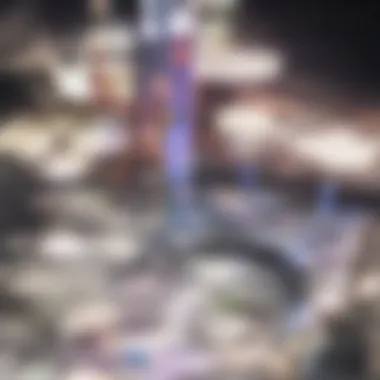
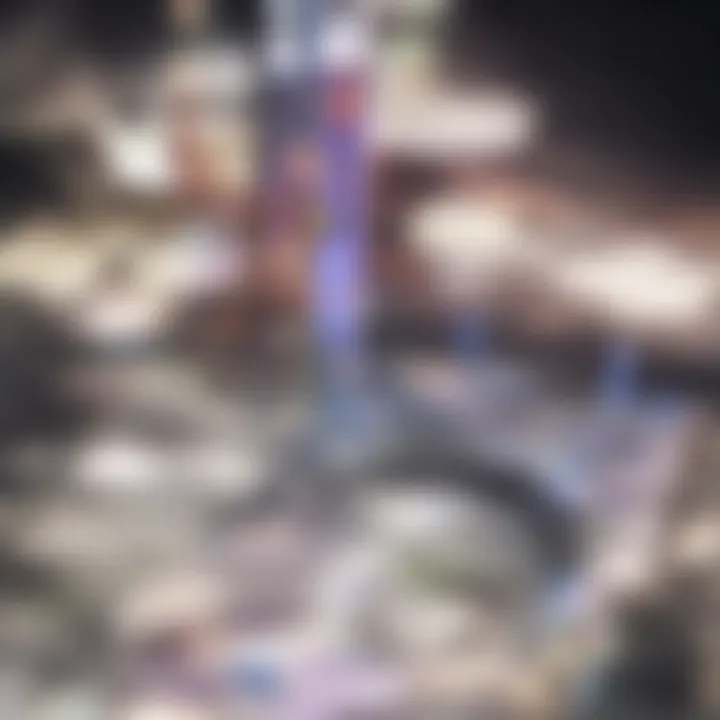
Physical disruption techniques refer to mechanical methods employed to lyse bacterial cells. These can involve bead beating, sonication, or homogenization. For instance, bead beating utilizes small beads to agitate the sample vigorously, leading to the mechanical breaking of cell membranes. This method is particularly effective for bacteria with tough cell walls, such as Gram-positive species.
The advantage of physical methods is their ability to deal with a wide variety of bacterial strains and can often achieve higher yields of DNA due to complete cell lysis. On the downside, these techniques can be quite labor-intensive and may require specialized equipment. Additionally, they run a risk of shearing the DNA, which could complicate subsequent analyses.
Enzymatic Digestion Approaches
Enzymatic digestion methods utilize specific enzymes to break down the cell wall and membrane components, such as lysozyme, proteinase K, and RNase. Lysozyme, in particular, is effective against Gram-positive bacteria due to its ability to degrade peptidoglycan in the cell wall. This method often pairs well with chemical lysis for comprehensive and effective DNA extraction.
The main benefit of enzymatic methods lies in their specificity and gentleness, leading to higher-quality DNA with fewer contaminants. This quality is essential when performing sensitive downstream applications like PCR or sequencing. However, the use of enzymes can introduce variability in the results depending on the incubation conditions and the purity of the enzymes used.
In summary, choosing the right DNA extraction technique is essential for achieving reliable results in genomic studies. Each method has its strengths and weaknesses. Therefore, understanding the bacterial structure and the desired application is crucial in making an informed decision.
In summary, choosing the right DNA extraction technique is essential for achieving reliable results in genomic studies. Each method has its strengths and weaknesses. Therefore, understanding the bacterial structure and the desired application is crucial in making an informed decision.
Step-by-Step Protocol for DNA Extraction
The extraction of genomic DNA from bacteria is a crucial step for a variety of molecular biology applications. Following a systematic and well-defined protocol helps to ensure the yield and quality of DNA, which is essential for downstream analyses such as sequencing, cloning, or PCR amplification. This section will provide a detailed look into the specific elements involved in the DNA extraction process, with an emphasis on the necessary preparations, the cell lysis stage, and the purification of the DNA product.
Preparation of Samples
Sample preparation is the first and critical stage in the DNA extraction protocol. It involves selecting the correct bacterial strains intended for extraction and optimizing their conditions. This includes ensuring that the samples are fresh or properly stored to maintain bacterial viability.
A few key considerations during the preparation phase include:
- Selection of bacterial culture: Choose cultures that are in the exponential growth phase for optimal yield.
- Inoculation methods: Utilize methods such as colony picking and liquid broth culturing to enhance sample quality.
- Cell concentration: Aim for a high cell density, as increased cell numbers generally improve DNA recovery rates.
Careful handling of samples and adherence to hygiene protocols are essential to prevent contamination. Additionally, maintaining sterility throughout the preparation process minimizes the risk of introducing unwanted genetic material.
Cell Lysis Process
The cell lysis stage is fundamental in releasing the genomic DNA from the bacterial cells. Here, various methods can be employed, such as chemical, physical, or enzymatic approaches. Each method has its own advantages and challenges, depending on the bacterial type and extraction goals.
- Chemical lysis: This method often involves detergents like sodium dodecyl sulfate (SDS), which disrupts the cell membrane and allows for DNA release. The choice of buffer is also significant, as it requires being compatible with the desired downstream applications.
- Physical lysis: Techniques such as bead beating or high-pressure homogenization can also be effective. These approaches apply physical force to shear cell membranes, releasing DNA. However, care must be taken to prevent shearing the DNA strands themselves, which can compromise the integrity of the genetic material.
- Enzymatic lysis: This involves the use of enzymes such as lysozyme, which breaks down the peptidoglycan layer of the cell wall in Gram-positive bacteria. It is a gentler technique, preserving DNA integrity better than harsher methods.
Effective lysis is paramount, as incomplete lysis may result in low yields of extracted DNA.
Purification of DNA
Purification follows cell lysis and is vital for isolating high-quality DNA free from cellular debris and contaminants. This can be accomplished through various techniques, such as phenol-chloroform extraction or using commercial DNA purification kits.
During this stage, it is important to:
- Remove proteins: Proteins and other contaminants can inhibit subsequent analyses. A proteinase treatment or a precipitation step is often employed to separate these components from the DNA.
- Precipitation: Ethanol or isopropanol is commonly used for DNA precipitation. The DNA precipitates out of the solution and can be collected via centrifugation.
- Washing steps: Washing the precipitated DNA with ethanol or other solvents helps in removing residual salts and impurities.
A final resuspension step in a suitable buffer or sterile water is necessary to stabilize the purified DNA for storage or analysis.
Successful purification of DNA is essential to ensure accurate results in downstream applications, making careful execution of this step critical.
Successful purification of DNA is essential to ensure accurate results in downstream applications, making careful execution of this step critical.
By following this structured step-by-step protocol for DNA extraction, researchers can optimize their workflows to achieve high yields and quality of genomic DNA suitable for various applications.
Quality Control of Extracted DNA
Quality control is a pivotal part of the genomic DNA extraction process. This phase ensures that the extracted DNA is suitable for downstream applications such as sequencing, cloning, and PCR amplification. Ensuring both the quantity and quality of the extracted DNA is essential, as deficiencies may lead to unreliable results in experiments. Therefore, rigorous quality checks should form an integral part of any extraction protocol.
In this section, we will discuss two main aspects of quality control in DNA extraction: assessing DNA quantity and evaluating DNA purity.
Assessing DNA Quantity
Determining the quantity of extracted DNA is crucial for understanding the success of the extraction process. Various methods can be employed for this purpose. Common techniques include:
- Spectrophotometry: This method measures the absorption of UV light at specific wavelengths (260 nm for nucleic acids), enabling quantification based on the Beer-Lambert law.
- Fluorometry: This technique involves applying fluorescent dyes that specifically bind to DNA. The increase in fluorescence is proportional to the amount of DNA present and offers higher sensitivity than spectrophotometry.
- Agarose Gel Electrophoresis: By running the extracted DNA on a gel, researchers can visually estimate the quantity compared to a known standard or DNA ladder.
Each method offers advantages and limitations regarding sensitivity, specificity, and ease of use. A proper method should be chosen based on the intended downstream applications and the concentration of DNA expected.
Evaluating DNA Purity
The next step in quality control is assessing the purity of the extracted DNA. Contaminants such as proteins, phenol, or salts can significantly impair subsequent applications. The purity of DNA is typically evaluated using the A260/A280 ratio derived from spectrophotometric analysis. An ideal ratio is around 1.8 to 2.0, indicating high purity. If the ratio is significantly lower, it suggests protein contamination; if higher, it may indicate contamination with RNA or other substances.
Other methods for evaluating DNA purity include:
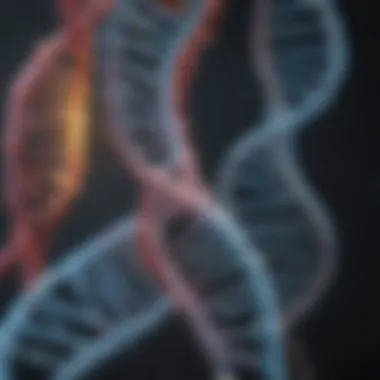
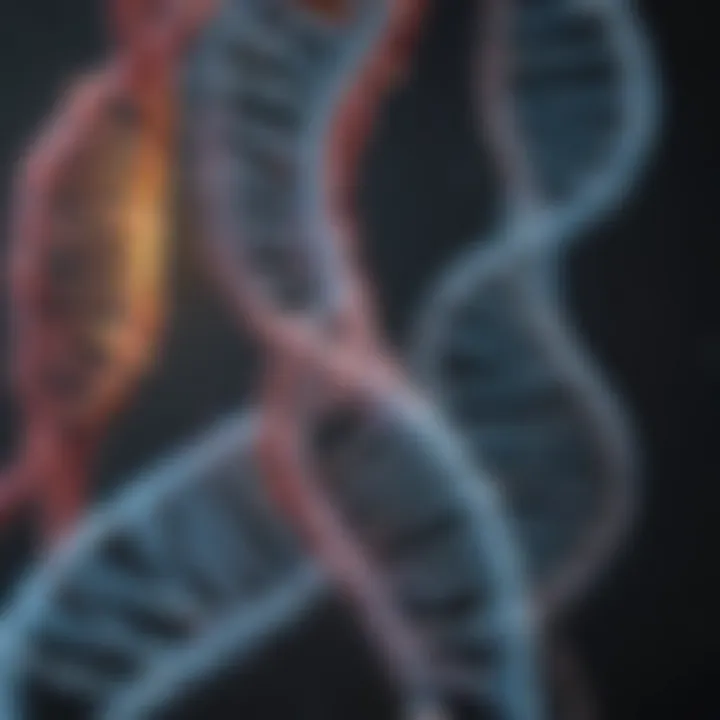
- Agarose Gel Electrophoresis: This can also provide a visual representation of DNA integrity and size.
- Nanodrop Analysis: This quick method requires only a minuscule sample size and also reports on additional anomalies in purity that might go undetected by other means.
It is important to incorporate both DNA quantity and purity assessment into the routine workflow post-extraction. Together, they help in validating the extraction procedures and ensuring the reliability of experimental results.
"Quality control in DNA extraction should not be an afterthought but a fundamental component of the entire workflow."
"Quality control in DNA extraction should not be an afterthought but a fundamental component of the entire workflow."
Successful genomic DNA extraction enhances research quality and reliability in genomics. Researchers should routinely document these quality metrics for ongoing evaluation and method improvement.
Challenges in DNA Extraction
DNA extraction from bacterial sources is a critical procedure in molecular biology. However, it can be fraught with challenges that researchers must navigate. Addressing these challenges is essential for producing high-quality genomic DNA suitable for downstream applications. Understanding the nuances of these obstacles can significantly enhance extraction protocols and ensure the reliability of subsequent genetic analyses.
Contamination Issues
One of the foremost challenges in DNA extraction is contamination. Contaminated samples can lead to erroneous results and misleading conclusions. There are various sources of contamination.
- Reagents: reagents used in the extraction process can introduce foreign DNA into the samples. Using high-purity reagents is crucial.
- Equipment: surfaces and tools that are not sterilized can harbor unwanted DNA, risking cross-contamination. It is vital to adopt stringent sterilization protocols.
- Environmental Factors: airborne contaminants can settle on samples or reagents. Conducting procedures in clean environments, such as laminar flow hoods, can help mitigate this risk.
To reduce contamination, researchers can implement several strategies, including:
- Utilizing dedicated equipment for DNA extraction.
- Running negative controls during the extraction process to detect any contamination.
- Establishing a contamination-free workspace.
Implementing these measures is not just about obtaining clean samples but also about ensuring the integrity of the research.
Low Yield of Extracted DNA
Another significant challenge is the low yield of extracted DNA. Low yields can result from many factors, complicating the extraction process further. Some key considerations include:
- Cell Lysis Efficiency: Ineffective lysis can lead to insufficient DNA recovery. Choosing the right lysis method that breaks down cell walls is essential.
- Sample Type: Different bacteria have varying cell wall structures, which can hinder the extraction process. For example, Gram-positive bacteria typically require more rigorous lysis conditions than Gram-negative bacteria.
- Extraction Methodology: The chosen method may not be optimized for specific bacterial species, leading to inadequate yields. Thus, tailoring the technique based on the bacterial strain can enhance results.
Low yield issues can be addressed by:
- Optimizing lysis conditions based on the bacterial type being studied.
- Carefully following the extraction protocol to avoid procedural errors.
- Using techniques that enhance DNA recovery, such as silica-based methods or magnetic bead techniques.
Ultimately, overcoming these challenges in DNA extraction is fundamental for researchers aiming to achieve reliable and high-quality genomic material.
"Contamination and yield issues are not merely technical hurdles but critical factors that shape the reliability of genomic studies."
"Contamination and yield issues are not merely technical hurdles but critical factors that shape the reliability of genomic studies."
Focusing on these challenges equips researchers with the knowledge to refine their protocols, ensuring that the genomic DNA extracted is both clean and in sufficient quantity for further analysis.
Applications of Extracted Genomic DNA
The extraction of genomic DNA from bacteria is not just a technical procedure; it serves as the foundation for a multitude of applications in various fields. Understanding these applications illustrates the paramount importance of precision and consistency in the extraction process. Researchers and practitioners must recognize the value of high-quality genomic DNA, as it directly influences the reliability of subsequent analyses and the validity of experimental outcomes. Applications can be grouped primarily into genomic sequencing, molecular cloning, and polymerase chain reaction (PCR) amplification. Each of these applications highlights unique benefits and considerations that can significantly impact research efforts.
Genomic Sequencing
Genomic sequencing refers to the process of determining the complete DNA sequence of an organism's genome. In the context of bacteria, this application is particularly significant due to the pathogen's diverse adaptations and rapid evolution. High-quality extracted DNA is crucial for accurate sequencing results. Typically, Next Generation Sequencing (NGS) technologies are employed, enabling researchers to analyze vast amounts of genetic data efficiently.
A well-extracted bacterial genomic DNA serves as the starting point for sequencing projects aimed at:
- Identifying new strains of bacteria that may have clinical relevance.
- Studying genomic variations that could provide insights into antibiotic resistance.
- Investigating phylogenetic relationships among different bacterial species.
The implications of these activities extend beyond academia, as they pave the way for advancements in medical diagnostics and public health responses. Accurate genomic data leads to better-targeted treatments in clinical settings.
Molecular Cloning
Molecular cloning entails the insertion of a specific DNA sequence into a vector, which can then be replicated within a host organism. This technique relies on high-quality extracted genomic DNA to ensure that the desired fragments are accurately amplified and maintained in the host's genome.
Applications of molecular cloning in bacterial DNA include:
- Creating recombinant proteins: These proteins may possess therapeutic properties or have industrial applications.
- Gene expression studies: Understanding how genes function in various conditions allows researchers to explore gene regulation and expression patterns.
- Development of genetically modified organisms: These organisms can be tailored for specific purposes, including agriculture and bioremediation.
The success of molecular cloning largely hinges on the initial quality of the extracted DNA. Contaminants can negatively influence transformation efficiency and, consequently, the success rate of subsequent experiments.
PCR Amplification
Polymerase Chain Reaction (PCR) amplification is a cornerstone technique in molecular biology. It allows for the specific amplification of a targeted DNA sequence, making it possible to analyze small amounts of DNA efficiently. This technique is widely used across various fields, from forensic science to clinical diagnostics.
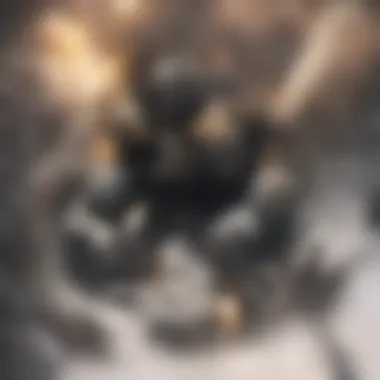

In bacterial genomic studies, PCR can:
- Detect specific pathogens in clinical samples, aiding in timely diagnoses.
- Amplify genetic material for further analysis, including sequencing or cloning.
- Perform quantitative analyses of bacterial populations in environmental studies.
It is critical to use high-quality extracted DNA free from inhibitors to achieve reliable PCR results. Substandard DNA can lead to false negatives or inconsistent results, compromising the integrity of the study.
High-quality extracted genomic DNA is essential for reliable results in genomic sequencing, molecular cloning, and PCR amplification.
High-quality extracted genomic DNA is essential for reliable results in genomic sequencing, molecular cloning, and PCR amplification.
In summary, the applications of extracted genomic DNA are diverse and impactful across multiple domains. Each application not only highlights the importance of meticulous extraction techniques but also emphasizes the need for continuous advancements in methodologies to ensure that researchers obtain the best possible results in their scientific endeavors.
Emerging Technologies in DNA Extraction
Emerging technologies in DNA extraction are revolutionizing how researchers obtain genomic material from bacterial sources. These innovations are not just about efficiency; they also enhance the quality and reliability of the extracted DNA. As the field of genomics continues to expand, the integration of novel techniques offers unique solutions to longstanding challenges in DNA extraction processes.
Advantages of Emerging Technologies
Modern techniques like microfluidic devices and automated extraction systems promise significant improvements. They can minimize contamination risks, reduce the time and labor involved, and yield higher purity of DNA. Adopting these technologies can facilitate reproducibility in experiments, making findings more reliable. Additionally, they can help to streamline workflows, enabling researchers to focus on analysis rather than tedious manual steps.
Microfluidic Devices
Microfluidic devices utilize small-scale liquid handling systems to manipulate fluids at the microscale. This approach allows for precise control over reaction conditions and reduces reagent consumption. One of the main advantages of microfluidics in DNA extraction is the potential for high-throughput processing. Researchers can extract DNA from multiple samples simultaneously, saving both time and resources.
Moreover, microfluidic devices can enhance automation in the extraction process. This can significantly decrease human error, leading to more consistent results. Another benefit is the ability to integrate different steps of DNA extraction in one platform, from lysis to purification.
"Microfluidics allows researchers not only to reduce sample sizes but also to increase the efficiency of DNA extraction, creating opportunities for innovations in genomics."
"Microfluidics allows researchers not only to reduce sample sizes but also to increase the efficiency of DNA extraction, creating opportunities for innovations in genomics."
However, there are considerations to keep in mind. The development of these systems requires specialized knowledge in both biology and engineering. In addition, the initial investment cost may be higher compared to traditional methods.
Automated Extraction Systems
Automated extraction systems represent another significant advancement in the field. These systems reduce the workload on laboratory personnel by automating repetitive steps in the DNA extraction protocol. This not only boosts productivity but also limits the risk of contamination associated with manual processing.
Automated systems can standardize procedures, leading to more reproducible results. Many biotechnological companies have developed commercially available systems that can process numerous samples simultaneously, which is crucial for large-scale studies. Some systems utilize magnetic bead technology for efficient DNA capture, resulting in purer extracts.
While the benefits are clear, there are also limitations. The performance of these systems can vary based on the type of bacterial sample and the specific requirements of the extraction method. Additionally, users need sufficient training to operate these complex machines effectively.
In summary, emerging technologies like microfluidic devices and automated extraction systems are reshaping DNA extraction processes. They offer numerous advantages that cater to the evolving needs of researchers in genomics and biotechnology. As these technologies continue to develop, they are likely to play a critical role in future studies involving bacterial RNA and DNA.
Future Directions in Genomic DNA Extraction
Future directions in genomic DNA extraction from bacteria are essential to explore due to the rapid advances in technology and an increasing need for high-quality genetic material. As research methodologies evolve, the techniques for extracting DNA must also adapt to retain efficacy and efficiency. In this section, we delve into two significant areas: innovations in protocols and the integration of artificial intelligence in DNA extraction.
Innovations in Protocols
The methodology for genomic DNA extraction is continuously being refined. Innovations often focus on enhancing yield, minimizing contamination, and simplifying protocols to make them suitable for diverse bacterial species. New protocols might involve novel chemical reagents or optimized physical disruption methods that improve lysis efficiency.
- Optimization of Chemical Reagents: Advances in chemical agents are vital as they can demonstrate improved cell lysis and reduced toxicity to DNA. For instance, using specialized buffers that stabilize DNA during extraction can lead to higher-quality genomic material.
- Streamlined Procedures: Recent protocols emphasize speed without compromising quality, often through multi-step processes that combine lysis, purification, and analysis into fewer steps. This streamlined approach reduces handling time and potential sample loss.
- Green Chemistry: There is a growing movement toward environmentally friendly extraction methods that utilize less hazardous materials. This minimization not only lowers risks in laboratory settings but also aligns with sustainability goals in scientific research.
Integration of AI in DNA Extraction
Artificial intelligence (AI) is transforming many fields, and genomic DNA extraction is no exception. The incorporation of AI technologies promises to enhance the accuracy and efficiency of extraction protocols significantly.
AI can assist in various phases of the extraction process:
- Predictive Analysis: Machine learning algorithms can analyze historical extraction data to predict the most efficient techniques for specific bacterial strains. This level of customization can improve overall yields.
- Process Optimization: AI can optimize protocols in real time, adjusting parameters based on feedback from extraction runs, thus reducing variability associated with human error. This intelligence-based feedback could lead to higher reproducibility in results.
- Automated Systems: AI integrates with automated lab systems, enhancing both throughput and sample handling. Automation not only speeds up the processes but can also implement standardized conditions for all samples, fostering consistency in results.
The intersection of AI and DNA extraction techniques harbors the potential for unprecedented advancements, significantly improving the quality of genomic studies.
The intersection of AI and DNA extraction techniques harbors the potential for unprecedented advancements, significantly improving the quality of genomic studies.
Finale
The conclusion serves as a pivotal segment in this article, distilling the wealth of information on genomic DNA extraction from bacteria into cohesive insights. This discussion reflects the intricacies of the extraction process, along with its implications for wider scientific applications. It underlines both the advancements achieved in the field and the challenges that remain.
Summarizing Key Insights
In summary, the article has highlighted several key dimensions of DNA extraction that are essential for researchers and practitioners. These insights include:
- Importance of DNA Extraction: Understanding the role of DNA extraction as a gateway to various applications in genomics and biotechnology. Precise extraction techniques ensure high-quality DNA, crucial for subsequent analyses.
- Diverse Extraction Techniques: The evaluation of chemical, physical, and enzymatic methods showcases that selecting the right technique can significantly impact yield and purity of extracted DNA.
- Quality Control Measures: Assessments of DNA quantity and purity are vital steps in ensuring the reliability of results. Techniques for maintaining quality must not be overlooked, as they directly influence the success of downstream applications.
- Challenges and Innovations: Knowledge of potential contamination and low yield issues informs better experimental design. Awareness of emerging technologies such as microfluidic devices and automated systems provides avenues for future improvements in extraction efficiency.
- Future Directions: Insights into innovations in protocols and the integration of artificial intelligence illustrate the dynamic nature of the field. These developments promise to refine extraction techniques and enhance the quality of outcomes.
Overall, this article emphasizes that genomic DNA extraction is not merely a procedural task but an essential component of contemporary biological research. By synthesizing information from various sectors, readers can appreciate the complexities and significance of DNA extraction, paving the way for informed decisions in their scientific endeavors.
"Understanding the fundamentals of DNA extraction processes is paramount for any researcher aiming to delve into genomics and molecular biology."
"Understanding the fundamentals of DNA extraction processes is paramount for any researcher aiming to delve into genomics and molecular biology."
The continuous evolution in techniques underlines an ever-growing promise for advancements in research, emphasizing the importance for professionals in the field to stay updated with the latest frameworks and methodologies.