Cathode Active Materials: Composition and Innovations
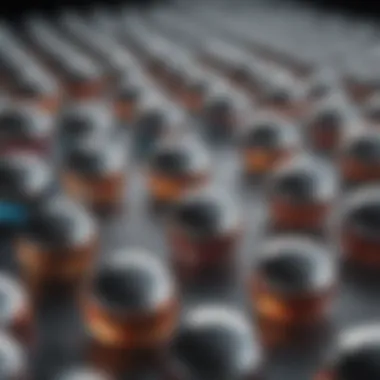
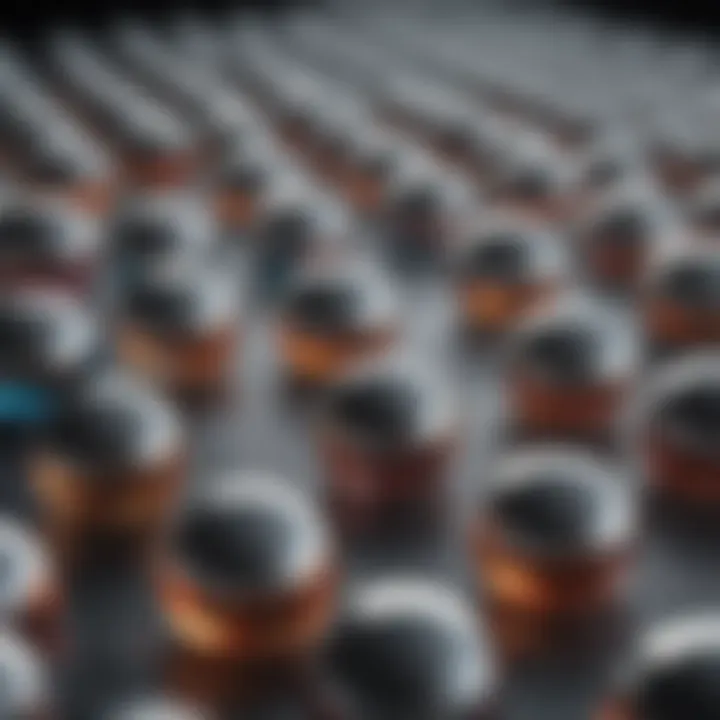
Intro
In the rapidly evolving world of battery technology, cathode active materials are often undeservingly overshadowed by anode development and electrolyte composition. However, these materials are the unsung heroes, establishing the very foundation upon which battery performance stands. Steering electric vehicles and portable electronics, cathode materials facilitate significant chemical reactions that ultimately unleash energy for consumption. As such, comprehending their composition and the associated challenges becomes paramount for anyone interested in energy storage solutions.
Article Overview
Purpose of the Article
This article aims to illuminate the complexities of cathode active materials by dissecting their composition, key characteristics, and the critical role they play within battery systems. Additionally, it will delve into the ongoing challenges that researchers confront while innovating these materials, covering recent advancements that may redefine future energy storage capabilities.
Relevance to Multiple Disciplines
The implications of advancements in cathode materials span far beyond just battery technology. Areas like environmental science, mechanical engineering, and materials science are intricately linked to the innovations of cathode systems. Students and professionals in these sectors can glean valuable insights, fostering interdisciplinary collaboration that enhances both research and application.
Research Background
Historical Context
The journey of cathode materials began with simple compositions like lead dioxide in lead-acid batteries. Over time, as technology progressed, so did the demand for materials that delivered greater energy density, stability, and efficiency. Lithium-ion batteries emerged as a game changer, bringing with them a slew of new materials such as lithium cobalt oxide and nickel manganese cobalt (NMC) that set the stage for future exploration and improvement.
Key Concepts and Definitions
When discussing cathode active materials, it's essential to grasp a few pivotal concepts:
- Energy Density: Refers to the amount of energy stored per unit mass or volume. It influences how long a battery can run on a single charge.
- Cycle Life: The number of charge and discharge cycles a battery can undergo before its capacity diminishes significantly.
- Thermal Stability: Critical for safety, this aspect determines how well the material can withstand temperature fluctuations during operation.
"The battery's cathode is like the heart of a living organism – it keeps the life force flowing, making knowledge about its structure and challenges all the more vital."
"The battery's cathode is like the heart of a living organism – it keeps the life force flowing, making knowledge about its structure and challenges all the more vital."
Understanding these key factors will pave the way for a deeper examination of current materials and future innovations in the realm of energy storage.
Prelude to Cathode Active Materials
Cathode active materials play a crucial role in the development and functioning of batteries, impacting everything from energy storage capacity to overall performance and durability. As society's reliance on battery technology continues to grow due to the proliferation of electric vehicles, renewable energy storage systems, and portable electronics, understanding these materials becomes essential for innovation and improvement in various applications.
At their core, cathode active materials determine how efficiently energy can be stored and released. They are the soul of the battery, influencing voltage levels and capacity retention over multiple charge cycles. A well-designed cathode can mean the difference between a battery that lasts for years and one that falters after a few months of use. Thus, the importance of this topic cannot be overstated.
In exploring the composition, challenges, and innovations associated with these materials, this article aims to provide insight into not just the science behind cathodes but also their practical implications in real-world situations. For students and researchers delving into battery technology, it is vital to understand the intricate balance of material properties, performance, and cost-effectiveness.
Considerations of safety and sustainability are also integral to the conversation around cathode materials. With the evolution of technologies, the focus is shifting towards greener alternatives, enhancing the efficiency of lithium-ion systems while minimizing environmental impacts. It’s a thrilling time for the field, and knowledge here paves the way for future breakthroughs.
"Battery technology is not just about performance metrics; it's about integrating sustainability into our everyday lives."
"Battery technology is not just about performance metrics; it's about integrating sustainability into our everyday lives."
Whether it be an engineer designing the next generation of cathodes or a researcher striving to address material degradation, the dialogue around these active materials encompasses multiple perspectives and disciplines. In sum, this section lays the groundwork for a deeper exploration of a topic that is both fascinating and vital as we transition into an era increasingly defined by energy storage capabilities.
Fundamental Composition of Cathode Active Materials
The fundamental composition of cathode active materials lays the groundwork for understanding battery technology. It encompasses a variety of materials, each playing a distinct role in enhancing the efficiency, safety, and versatile applications of energy storage solutions. Crucial to creating batteries that can withstand rigorous conditions and provide reliable performance, the right materials can make or break the functionality of battery systems.
Here’s why comprehending the composition is vital:
- Energy Density Optimization: Different materials provide various energy densities, which is essential for applications ranging from electric vehicles to portable electronic devices.
- Cost Implications: The selection of components can significantly affect manufacturing costs, directly influencing market pricing of the end products.
- Environmental Impact: Understanding the origin and sustainability of materials leads to better decisions regarding the recycling and disposal of batteries.
Overview of Common Materials
The cathode active materials showcase a range of common ingredients, each of which plays a pivotal role in battery performance.
Lithium Cobalt Oxide
Lithium Cobalt Oxide, often known in the industry as LCO, stands tall among the choices for lithium-ion batteries. One key characteristic of LCO is its high energy density, making it a compelling choice for devices requiring compact energy storage, like smartphones and laptops. Its crystal structure provides stability during charge and discharge cycles, positively affecting battery lifespan.
However, LCO has its caveats. Material scarcity and rising cobalt prices stem from ethical concerns and geopolitical issues. This can drive up production costs and pose sustainability challenges.
Nickel Manganese Cobalt
The popularity of Nickel Manganese Cobalt, or NMC, isn’t without reason. It smartly blends three elements, leading to enhanced thermal stability and safety. This compound allows manufacturers to adjust the ratios of nickel, manganese, and cobalt to customize energy density and power output. NMC has carved its niche in the electric vehicle industry, as it offers a balanced trade-off between energy density and cycle stability.
A unique aspect of NMC is its moderate thermal stability, which can sometimes be improved by optimizing the material’s composition. Thanks to this flexibility, NMC continues to gain traction as a hybrid material in various applications.
Lithium Iron Phosphate
Then there's Lithium Iron Phosphate, abbreviated as LFP, known for its exceptional safety and thermal stability properties. Ideal for applications where longevity is valued over energy density, LFP batteries can endure many charge-discharge cycles without substantial degradation.
The unique feature of LFP is its capacity for sustained high-temperature performance while remaining resistant to thermal runaway. On the downside, its energy density is lower compared to competing materials, which can be a detractor in weight-sensitive applications like drones or electric bikes.
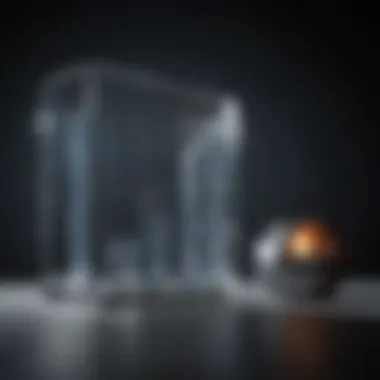
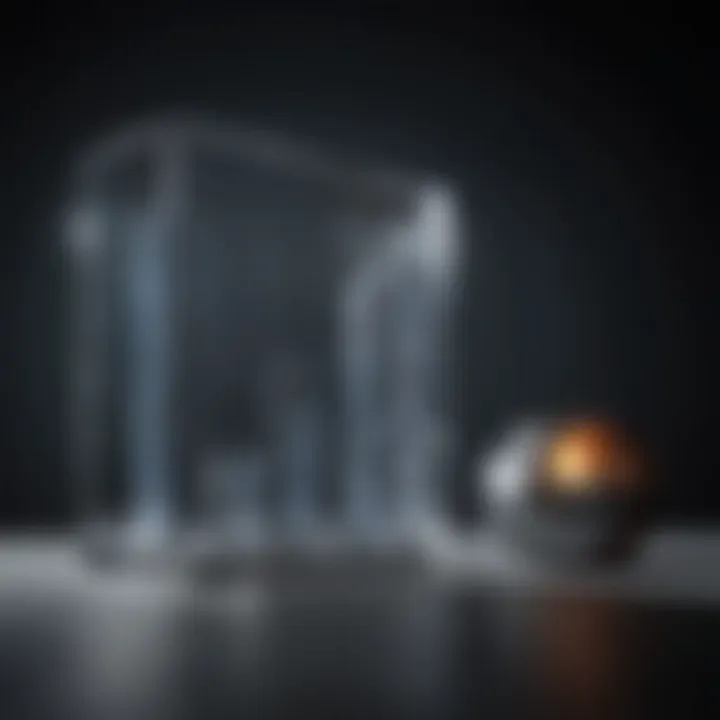
Role of Conductive Additives
Moving beyond the battery composition itself, conductive additives play a crucial role in performance enhancement. They bridge the gap between cathode materials and overall battery efficiency.
Carbon Black
Carbon Black serves as a vital conductive additive in cathode formulations. Its primary function is to improve electrical conductivity, ensuring that electrons have an efficient pathway during the charge and discharge processes. This material is cost-effective yet remarkably efficient, often incorporated at low percentages to enhance overall performance. Additionally, it provides a mechanical support system for the other materials, further boosting durability.
However, a downside to Carbon Black is its potential for contamination if not sourced correctly, which can affect battery performance in the long run.
Graphene
Graphene brings a new game to the table as a revolutionary material for conductive additives. Its astounding surface area and conductivity make it a highly desirable option for modern battery applications. Its unique structural strength not only enhances the electrical pathways but also provides mechanical strength to the electrode materials.
Despite high manufacturing costs and challenges associated with large-scale production, the potential of Graphene in improving the performance of cathode active materials is too significant to ignore. As research advances, we may very well see it break into mainstream battery technology, reshaping how we think about energy storage.
"An in-depth understanding of the composition of cathode materials is pivotal for leading future innovations in battery technology."
"An in-depth understanding of the composition of cathode materials is pivotal for leading future innovations in battery technology."
By focusing on these materials and additives, the article seeks to unravel the complexity behind cathode active materials, delving into various aspects that interplay to create efficient energy storage solutions.
Physical and Chemical Properties
The physical and chemical properties of cathode active materials lay the groundwork for their performance in energy storage systems. A thorough grasp of these characteristics is key for advancement in battery technology. Specifically, electrical conductivity and thermal stability stand as two pillars that determine how effectively these materials can function under various conditions. Understanding these elements not only aids in material selection but also guides innovations aimed at enhancing overall battery efficiency and lifespan.
Electrical Conductivity
Electrical conductivity refers to a material's ability to allow electric charge to flow through it. In the context of cathode materials, this property is crucial, as it directly impacts the efficiency of energy transfer during charging and discharging processes. Good conductivity ensures that ions can move freely between the cathode and electrolyte, thus maintaining a robust current flow.
When evaluating the conductivity of different materials, lithium cobalt oxide, for instance, generally offers higher conductivity compared to its counterparts like lithium iron phosphate. However, variances in conductivity can also be influenced by factors such as particle size and the presence of conductive additives.
Consider the role of additives like carbon black and graphene, which are often used to enhance conductivity. These materials facilitate easier ion transport, ultimately improving battery performance. Conductive pathways formed by these additives create a synergistic effect that enhances the material's conductive properties. Here’s a breakdown of key points regarding electrical conductivity in cathode materials:
- Ionic Mobility: Higher ionic mobility translates to faster charging times.
- Additive Selection: The choice of conductive additives can significantly modify the overall conductivity.
- Temperature Effects: Conductivity can vary with temperature, making it essential to consider operating conditions.
"The efficiency of a battery rests heavily on the electrical pathways constructed by cathode materials and their constituents."
"The efficiency of a battery rests heavily on the electrical pathways constructed by cathode materials and their constituents."
Thermal Stability
Thermal stability is another cornerstone in assessing the performance of cathode materials. Essentially, it evaluates how well these materials can maintain structural integrity and functionality at varied temperatures. Poor thermal stability can lead to overheating, material degradation, or even catastrophic failure, all of which can compromise the operational safety of a battery.
Lithium iron phosphate, for example, is known for its good thermal stability compared to lithium cobalt oxide, making it a favorable choice for applications where overheating is a concern. Stability under high temperatures allows for prolonged usage without the risk of thermal runaway events, thereby enhancing safety in battery applications.
Factors influencing thermal stability include:
- Material Composition: Different elemental compositions exhibit varying thermal behaviors.
- Particle Morphology: The shape and size of the particles can affect thermal pathways.
- Environmental Conditions: External factors such as humidity and surrounding temperatures also play a role.
Overall, understanding the interrelation between electrical conductivity and thermal stability is paramount. It paves the way for developing high-performance cathode materials that are not only efficient but also reliable under practical operating conditions.
Performance Metrics of Cathode Materials
When it comes to evaluating cathode materials, performance metrics are paramount. They determine how effectively these materials will function in batteries, directly impacting energy storage technologies. Understanding these metrics allows researchers and manufacturers to optimize currently used materials while seeking innovative alternatives. Key areas of focus include capacity, energy density, cycle life, and stability.
Capacity and Energy Density
The terms "capacity" and "energy density" often pop up as critical performance metrics in the realm of cathode materials. Capacity refers to the amount of electric charge a battery can store, typically measured in ampere-hours (Ah). Energy density, on the other hand, is a measure of how much energy a battery can deliver for a given weight or volume, generally expressed in watt-hours per kilogram (Wh/kg).
These two metrics are fundamentally intertwined. Higher capacity usually translates to greater energy density, enabling devices to run longer without requiring a recharge. For instance, lithium cobalt oxide (LiCoO2), a common cathode material, generally boasts high energy density, making it desirable for applications where weight and size matter, like in smartphones and electric vehicles. This demand elevates the material's role significantly, as its inherent properties influence the overall battery efficiency.
However, achieving high capacity isn't purely a matter of selecting the right materials. Factors such as electrolyte composition, operating temperature, and charge/discharge rates can all influence performance. As a result, researchers continuously probe the labyrinth of materials science to enhance these characteristics. Some emerging alternatives, like nickel manganese cobalt (NMC) oxides, promise similar or improved metrics, albeit with additional benefits like increased thermal stability.
Cycle Life and Stability
Next up, cycle life and stability stand as vital indicators of a cathode material's longevity and reliability. Cycle life refers to the number of complete charge-discharge cycles a battery can undergo before its capacity falls below a given percentage of its original capability, often noted at 80%. Stability pertains to how well the material retains its properties under various conditions.
A cathode material's cycle life can have drastic implications, especially in devices that rely on consistent and prolonged power supply. For example, lithium iron phosphate (LiFePO4) is known for its exceptional cycle life, often exceeding a couple thousand charging cycles. That makes it exceedingly suitable for applications like electric buses which require durable batteries able to withstand repeated use without significant degradation.
Stability is critical as well. A stable cathode material can endure fluctuations in temperature and varying charge rates without compromising its performance. This is especially important for applications in fluctuating environments, such as renewable energy storage systems. If a battery can maintain performance under stressful conditions, it's that much closer to becoming a go-to solution for practical implementation.
In summary, scrutinizing these performance metrics isn't just academic detachment; it is the crux of innovation that influences user experiences across technology sectors. It is essential to focus on the synergy between capacity, energy density, cycle life, and stability for the advancement of cathode materials.
Manufacturing Techniques
The manufacturing techniques used in the production of cathode active materials are vital for their performance and reliability in battery applications. The methods chosen can significantly affect the properties of the materials such as their conductivity, stability, and overall energy density. As energy demands escalate globally, the importance of robust and efficient manufacturing processes can't be overstated. These techniques encompass the approaches that either enhance the material quality or improve production scalability and cost-effectiveness.
Synthesis Methods
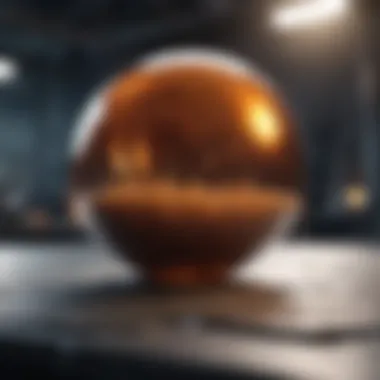
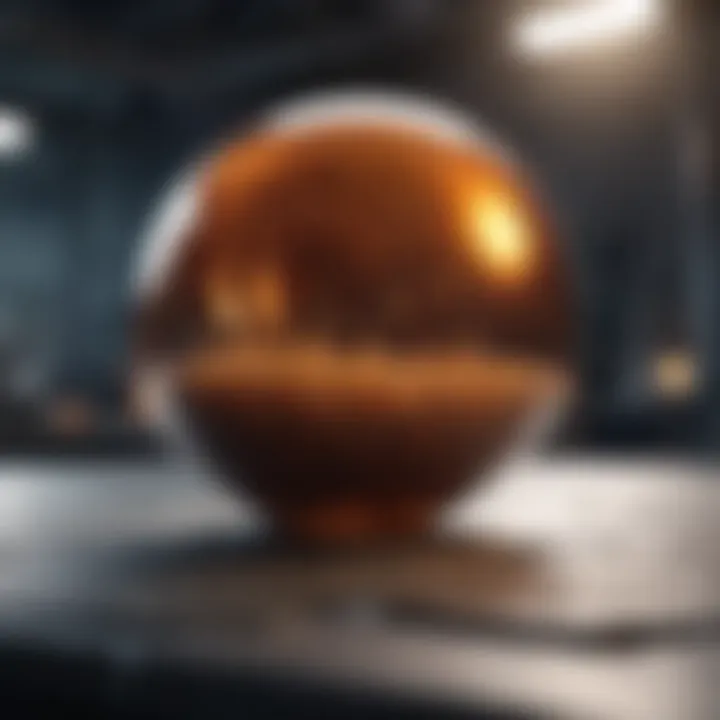
Solid-State Synthesis
Solid-state synthesis is a widely adopted approach for developing cathode materials, particularly due to its ability to produce high-purity compounds. This method typically involves mixing solid precursor materials at high temperatures. The main characteristic that stands out about solid-state synthesis is its capability to control stoichiometry effectively, ensuring that the desired composition is achieved with minimal impurities.
Solid-state synthesis is often seen as a reliable choice in the production line because it doesn't require solvents, making it more environmentally friendly compared to other methods. However, the key challenge with this technique lies in the high temperature requirement, which can be an energy-intensive operation.
The unique feature of solid-state synthesis is how it fosters a strong crystal structure, essential for the efficiency and longevity of battery performance. When executed properly, this method can result in > 90% of the theoretical capacity of the material being achieved, which makes it an attractive option in the industry.
However, the associated disadvantage of this method is that it can be less scalable for mass production compared to other techniques. Nevertheless, the quality achieved often offsets the higher energy costs incurred during production.
Solvothermal Synthesis
Solvothermal synthesis, on the other hand, offers a different angle, utilizing liquid solvents under elevated temperatures and pressures to enable chemical reactions. This method can yield materials with better homogeneity and the opportunity for developing nanostructured materials with tailored properties. A significant aspect of solvothermal synthesis is its versatility; it can be adjusted to synthesize a wide array of compound formulations according to the requirement.
The compelling characteristic of solvothermal synthesis is its relatively low temperature operation compared to solid-state synthesis. This quality not only reduces energy consumption but also minimizes thermal degradation of the compounds.
Unique in its ability to produce materials with fine control over morphology, solvothermal synthesis allows for the creation of nanoscale structures that can significantly enhance the surface area and ionic conductivity, both critical for improving battery performance.
Nevertheless, complexities arise when considering the requirements for solvent recovery and purification. This can add to the overall process costs, which may make it less appealing in economic terms.
Quality Control Measures
Quality control measures are essential in ensuring that the final cathode materials meet required specifications. They help mitigate risks associated with defects, impurities, and inconsistencies in performance.
Effective quality control relies on a stringent testing regimen throughout production, including raw material verification, in-process monitoring, and final product assessments. Each step in this sequence narrows down potential issues, ensuring that only the best quality materials make it to the market.
In crafting high-performance batteries, it's not just about selecting the right materials; it’s crucial to ensure that every aspect of production is carefully controlled. If a cathode material underperforms, it can have downstream effects, impacting the overall efficiency and lifespan of a battery.
Challenges in Cathode Material Development
The evolution of battery technologies hinges on the advancements made in cathode materials. The challenges faced in this realm are not just technical hurdles; they embody significant factors that can steer or stall progress in energy storage solutions. Understanding these challenges is paramount for researchers and manufacturers alike. It’s not merely about overcoming barriers but also about seizing opportunities that arise in addressing these pressing issues.
Material Degradation
One of the pivotal challenges in cathode material development is material degradation. Over time and usage, cathode materials can undergo various forms of degradation, negatively impacting a battery’s performance. Take, for example, lithium cobalt oxide, a popular choice for many rechargeable batteries. While it boasts excellent energy density, its structure is susceptible to deterioration after a number of charge cycles. After a while, it can lead to a capacity fade, a phenomenon where the maximum charge a battery can hold diminishes drastically.
Material degradation can stem from different processes:
- Mechanical Stress: Repeated expansion and contraction during charge and discharge cycles can create cracks or even collapse in the material's structure.
- Electrochemical Reactions: Unwanted reactions may occur at the interface of the electrolyte and cathode, leading to the formation of barriers that hinder lithium ion movement.
- Temperature Effects: High temperatures can accelerate chemical reactions that lead to further degradation.
"The long-term reliability of cathode materials remains a primary concern for automotive and consumer electronics sectors, where battery life significantly influences user satisfaction."
"The long-term reliability of cathode materials remains a primary concern for automotive and consumer electronics sectors, where battery life significantly influences user satisfaction."
Understanding these degradation mechanisms is essential for researchers. The search for new materials or innovative coatings that can mitigate these risks is ongoing, and the success of such initiatives could revolutionize battery longevity.
Cost-Effectiveness
Then there’s the question of cost-effectiveness. The price of raw materials, manufacturing processes, and the associated infrastructure can influence the feasibility of cathode materials being adopted at a larger scale. With the rising demand for batteries, particularly for electric vehicles and renewable energy storage, keeping costs in check has become critical.
Consider the case of lithium iron phosphate (LiFePO4). While it is a cost-effective choice with enhanced safety and thermal stability, its lower energy density compared to other materials like nickel cobalt manganese can deter its adoption in high-performance applications. Balancing cost and performance is a tightrope walk for manufacturers. They must evaluate:
- Raw Material Availability: Some materials are scarce or challenging to procure, which can drive up costs.
- Manufacturing Complexity: Complex synthesis methods can increase production costs, making them less attractive.
- Market Dynamics: External factors, such as global economic conditions and trade policies, can influence material prices and availability.
Many researchers are exploring alternative materials, such as sodium-ion batteries, which could potentially offer both lower costs and enhanced performance metrics.
Innovations in Cathode Technology
When it comes to the future of battery technology, innovations in cathode materials are undeniably pivotal. As the demand for sustainable energy storage solutions rises, researchers and manufacturers are on a quest to develop more efficient and effective materials that can enhance battery performance. This section sheds light on promising advancements, focusing particularly on two notable emerging materials: sodium-ion batteries and solid-state batteries. Additionally, we will delve into the role of nanotechnology applications in elevating cathode performance.
The importance of such innovations cannot be overstated, as they not only promise to improve energy density and lifespan but also address persistent issues like safety and environmental impact. In exploring these advancements, we can appreciate how they contribute significantly to the overarching goal of optimizing energy storage technologies.
Emerging Materials
Sodium-ion Batteries
Sodium-ion batteries have been gaining traction as an alternative to the prevalent lithium-ion technology. The standout feature of sodium-ion batteries is the abundance and low cost of sodium compared to lithium. This affordability presents a compelling case, particularly in regions where lithium resources are scarce or expensive.
Their key characteristic lies in the capability to operate efficiently in lower temperatures, which can be beneficial in diverse climates. However, sodium-ion batteries do come with their challenges. For instance, their energy density typically falls short when compared to lithium counterparts. This is where ongoing research aims to bridge the gap, potentially offering enhancements in electrode materials to elevate performance.
"Sodium-ion technology holds the potential to democratize energy storage solutions by making them accessible to wider demographics."
"Sodium-ion technology holds the potential to democratize energy storage solutions by making them accessible to wider demographics."
Solid-State Batteries
Solid-state batteries represent one of the most exciting developments in the field of energy storage. Unlike their liquid electrolyte-based predecessors, solid-state batteries utilize a solid electrolyte, which can significantly improve energy density. This makes them particularly attractive for applications where space and weight are at a premium, such as in electric vehicles.
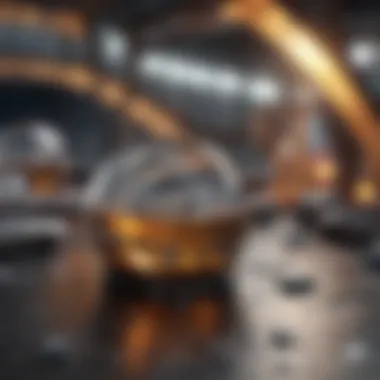
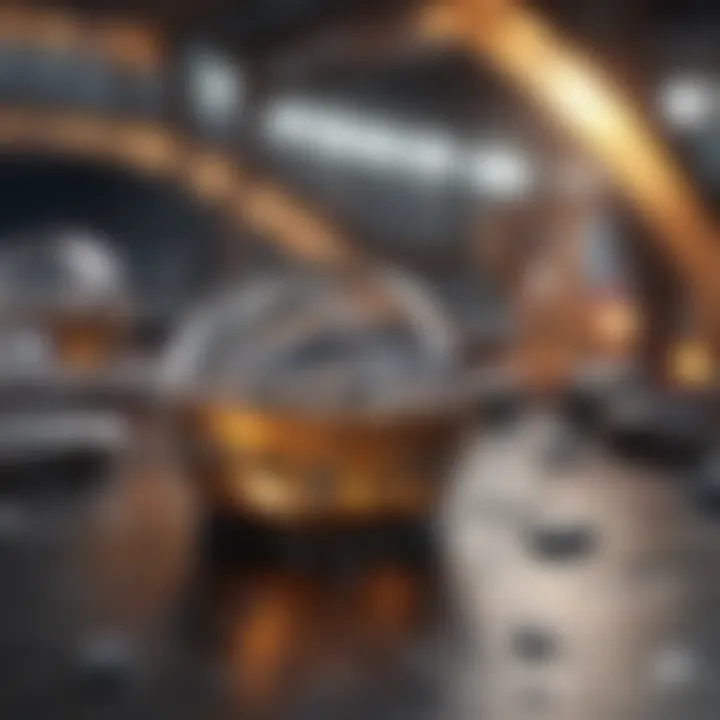
Their most impressive quality is safety. Solid-state batteries are less prone to leakage and thermal runaway, risks associated with liquid electrolytes. Nonetheless, challenges persist, especially around the cost of materials and scalability of production. Researchers are working diligently to overcome these hurdles, as solid-state technology could usher in a new era of high-performance batteries that prioritize user safety and environmental considerations.
Nanotechnology Applications
Nanotechnology has begun to revolutionize cathode active materials in more ways than one. By manipulating materials at the nanometer scale, researchers enhance properties such as conductivity, stability, and energy storage capacity. Through the incorporation of nanostructured materials, manufacturers can achieve uniform distribution of active materials, which leads to better performance in battery applications.
Moreover, carbon-based nanomaterials like graphene and carbon nanotubes are becoming essential components in enhancing the electrical conductivity of cathodes. Their unique structures not only improve charge transfer but also promote faster electron mobility. This, in turn, contributes to higher efficiency and longer lifecycle of batteries, which is crucial in meeting the demands of modern energy systems.
Innovations in cathode technology offer a glimpse into the future of energy storage. As we embrace new materials and applications, the drive toward sustainability and efficiency becomes clearer. Keeping abreast of these developments will be vital for stakeholders across the spectrum, whether in research or industry, as the narrative of energy storage continues to unfold.
Environmental Considerations
The significance of environmental considerations in the realm of cathode active materials cannot be overstated. This section sheds light on the need for sustainable practices within the battery industry, encompassing the entire lifecycle of materials. As society pushes towards renewable energy and electric vehicles, understanding the environmental impact of cathodes becomes crucial. Environmental considerations aren’t merely regulatory tick boxes; they are essential to the long-term viability of energy storage technologies and the overall reduction of our ecological footprint.
Sustainability of Supply Chains
Sustainability in supply chains directly influences the environmental footprint of cathode active materials. The sourcing and transportation of raw materials often leave a hefty carbon trail. To address this, manufacturers and researchers are now prioritizing the use of locally sourced materials whenever feasible. For instance, lithium extraction nations are examining methods to minimize environmental degradation. By leveraging more sustainable practices, such as using brine instead of hard rock mining, the industry can lower energy consumption and environmental risks.
Additionally, the practice of ethical sourcing has gained traction. Brands are increasingly set on verifying that their suppliers adhere to responsible mining practices. This essentially means avoiding environmental damage and respecting labor rights.
- Companies should consider:
- Assessing the carbon footprint of their supply chains.
- Investing in alternative materials that have less environmental impact.
- Engaging with local communities to ensure their practices benefit them as well.
In essence, a robust focus on sustainability can not only lessen environmental damage but also enhance the reputation of manufacturers. An eco-conscious brand tends to resonate well with today’s environmentally aware consumers.
Recycling Cathode Materials
The recycling of cathode materials is an area ripe with potential and necessity. With the increasing number of batteries reaching the end of their life, the notion of recycling isn't just good practice—it’s essential. Recycling not only helps in reclaiming valuable materials like lithium and cobalt but also minimizes waste and environmental hazards associated with discarded batteries.
Unlike traditional disposal methods that often end up in landfills, recycling offers a second life to these materials. Advanced processes now exist that can effectively extract active materials from spent batteries, paving the way for circular economies.
"Recycling proves to be a key player in reducing the carbon footprint of battery manufacturing while ensuring that valuable resources are reused effectively."
"Recycling proves to be a key player in reducing the carbon footprint of battery manufacturing while ensuring that valuable resources are reused effectively."
The benefits of recycling are monumental. They include:
- Conservation of Resources: Reducing the need for new raw materials cuts down on mining activity, which is often met with ecological opposition.
- Energy Savings: Recycled materials generally require less energy to process than raw materials, leading to lower emissions.
- Economic Opportunities: Establishing recycling plants could create jobs, driving local economies.
In summary, integrating recycling practices into the cathode production ecosystem is vital for achieving a balanced approach to environmental preservation and resource management. Advancing both recycling technologies and policies will, in turn, drive forward the sustainability of cathode active materials.
Future Directions in Research
The exploration of future directions in cathode active materials research is crucial as the demand for efficient energy storage solutions surges. With increasing reliance on renewable energy sources and the electrification of transportation, addressing the limitations of current materials is paramount. New approaches not only enhance performance but also align with sustainability goals.
Interdisciplinary Approaches
When it comes to innovating cathode technologies, interdisciplinary approaches present a remarkable leap forward. Combining knowledge from different fields like chemistry, materials science, and engineering fosters novel insights that drive breakthroughs. For instance:
- Chemistry brings forth new bonding techniques and compositions that can elevate the efficiency of cathode materials.
- Materials science provides an understanding of material behavior under various conditions, which is essential for developing more durable and effective materials.
- Engineering plays a pivotal role in scaling up laboratory successes for real-world applications, ensuring that innovations can be practically implemented.
These synergies can lead to exciting advancements, like the discovery of dual-ion batteries, which utilize both cations and anions to boost energy density.
"Interdisciplinary collaboration not only pushes the boundaries of scientific discovery but also paves the way for practical applications that can solve pressing energy challenges."
"Interdisciplinary collaboration not only pushes the boundaries of scientific discovery but also paves the way for practical applications that can solve pressing energy challenges."
Collaborative Innovations
Collaboration between academia, industry, and governmental agencies serves as a catalyst for transformative innovations in cathode materials. Together, they create an ecosystem of support and knowledge-sharing, enhancing research productivity. Some key elements of collaborative innovations are :
- Joint Research Initiatives: Partnerships enable sharing of resources and expertise, resulting in focused research endeavors that tackle specific challenges in cathode technology.
- Funding and Grants: Joint ventures unlock funding opportunities, leading to enhanced research capacity and access to high-tech laboratories.
- Technology Transfer: Communications between academia and industry facilitate the transition of research findings into market-ready products. This bridging can considerably reduce the time from discovery to application.
- Global Networks: Engaging in collaborative networks strengthens research integrity and diversity. This allows researchers to tap into a wealth of global insights and methodologies.
For example, initiatives like the European Battery Alliance illustrate a robust attempt at developing a competitive battery industry through collaboration, focusing on sustainability and regional resource utilization.
Culmination and Implications
The exploration of cathode active materials doesn't merely present a litany of technicalities and research findings; it serves as a crucial venture into an area that underpins the very functionality of today’s most prevalent energy storage solutions. The implications of this research stretch across various domains, from technological advancement to environmental sustainability.
Understanding the composition of these materials is not just academic; it plays a significant role in the trajectory of battery technology itself. As the shift toward electric vehicles and renewable energy sources intensifies, the efficiency, longevity, and cost-effectiveness of cathode materials become paramount. For example, lithium iron phosphate, with its unique stability and safety profile, is carving a niche in applications that prioritize durability while reducing flammability risk, thus offering an avenue for safer energy solutions.
Benefits of Comprehensive Knowledge
Several benefits arise from a detailed comprehension of cathode active materials:
- Performance Enhancement: Familiarity with various materials can lead to innovations that significantly boost energy density and cycle life. Understanding performance metrics allows researchers to make informed decisions about material choices that enhance overall battery performance.
- Cost Management: The exploration of alternative materials, such as sodium-ion solutions, hints at the possibility of reducing dependency on lithium and cobalt - materials often linked to volatile pricing and ethical sourcing concerns.
- Environmental Stewardship: With heightened awareness of the environmental impact of resource extraction and waste management, research into recycling cathode materials has emerged as not just a suggestion but a necessity. Bullish on sustainability, future developments likely include closed-loop systems aimed at minimizing waste and reinforcing resource cycles in battery production.
Considerations Moving Forward
As we gaze into the horizon of cathode material research, several considerations must guide our path forward:
- Interdisciplinary Collaboration: Scientists, engineers, and environmentalists must come together, combining their expertise to tackle the multifaceted challenges of battery technology. This collaboration can guide the exploration of next-generation materials that meet both performance and sustainability standards.
- Regulatory Standards and Safety Protocols: With new materials and technologies come the need for robust regulatory frameworks that ensure safety and efficiency without stymying innovation. Crafting these standards will require input from diverse stakeholders, including manufacturers, researchers, and consumers.
- Continued Innovation: Lastly, a commitment to continuous innovation is non-negotiable. There must be an open-mindedness towards newer forms of cathode materials, including solid-state batteries, which promise to offer unprecedented performance metrics. This avenue deserves attention, not only for its technological prowess but also for the potential it holds in addressing current material limitations.
In summary, the intricate landscape of cathode active materials is rich with possibilities and challenges. By deepening our understanding and fostering collaborative innovation, we stand poised at the brink of breakthroughs that could redefine energy storage and consumption for years, if not decades, to come. The discussions sparked by this article are just the beginning of what must be a broader exploration of how we can harness these materials to better serve both technological progress and our planet's well-being.