In-Depth Insights on Cell Culture Production Techniques
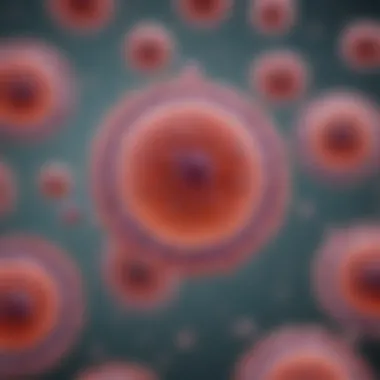
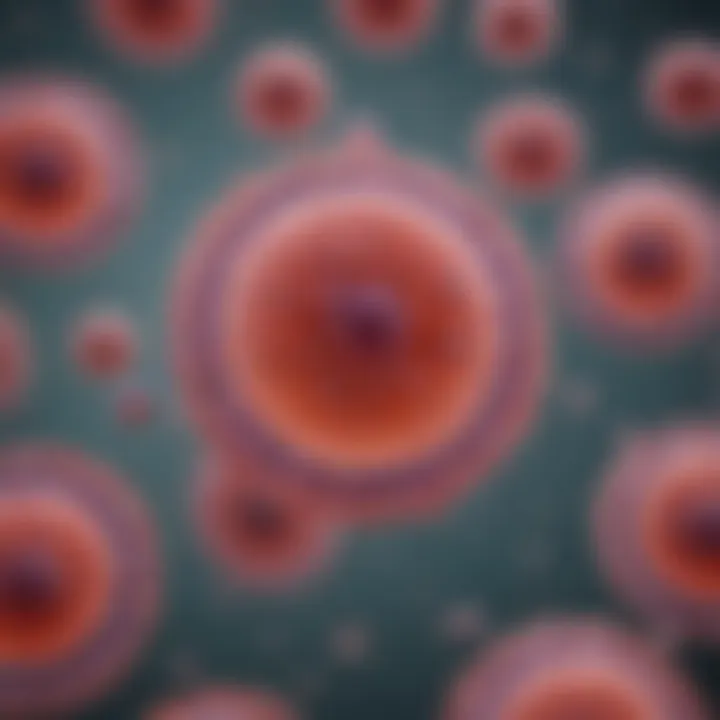
Article Overview
Purpose of the Article
The purpose of this article is to deliver a lucid and thorough examination of cell culture production, a critical aspect of biotechnology and pharmaceuticals. The exploration will encompass various methodologies employed in the field, the types of cells that are cultured, the intricacies involved in preparing culture media, and the significant environmental factors that impact cell growth. Understanding these elements is essential for anyone involved in drug development, vaccine production, or tissue engineering.
Relevance to Multiple Disciplines
Cell culture production spans numerous disciplines, directly influencing biotechnology, medicine, and environmental sciences. Researchers can utilize these methods to:
- Investigate disease mechanisms.
- Develop biological therapies.
- Conduct toxicity testing.
- Create biopharmaceuticals and diagnostics.
This relevance underscores the interdisciplinary nature of cell culture and its implications for advancements in medicine and science.
Research Background
Historical Context
The use of cell culture dates back to the early 20th century when pioneers such as Alexis Carroll developed techniques for cultivating cells outside their natural environment. Over the decades, advancements in technology have significantly changed how cells are cultured. Early challenges included contamination and maintaining sterility, which have been greatly mitigated through modern protocols and equipment.
Key Concepts and Definitions
Understanding cell culture production requires familiarity with several key concepts:
- Cell lines: These are populations of cells derived from a single cell, allowing for consistent experiments.
- Culture media: The nutrient solution used to support cell growth, containing essential nutrients, vitamins, and minerals.
- Sterility: Maintaining a contamination-free environment crucial for successful cell growth.
- Passaging: The process of transferring cells to fresh culture media to continue growth.
Additionally, cell culture techniques can be subdivided into adherent and suspension methods, each utilizing different approaches to support cellular growth.
Cell culture is not merely about growing cells; it is about understanding their behavior and interactions in a controlled environment, providing insights that cannot be achieved through other methodologies.
Cell culture is not merely about growing cells; it is about understanding their behavior and interactions in a controlled environment, providing insights that cannot be achieved through other methodologies.
Intro to Cell Culture Production
Cell culture production serves as the foundation of many innovative developments in biotechnology and pharmaceuticals. Understanding its significance is crucial for researchers, educators, and professionals in the field. This section will delve into what cell culture is and why it is essential in modern science.
Definition and Importance
Cell culture is the process of cultivating cells in a controlled environment outside their natural habitat. This method allows scientists to study cell behavior, develop drugs, produce vaccines, and engineer tissues. The importance of cell culture production cannot be overstated. It provides a model to understand cellular functions, diseases, and drug interactions.
Furthermore, cell culture plays a key role in the reproducibility of experiments. By using standardized conditions, researchers can ensure that results are consistent and reliable. This reproducibility is vital for both academic research and industrial applications.
Moreover, advancements in cell culture techniques have led to significant improvements in production efficiency. For instance, high-density cell cultures enable larger yields of proteins and antibodies, essential for therapeutic applications. Every progress in this area translates to better healthcare solutions.
Historical Context
The history of cell culture traces back to the early 20th century when scientists first successfully isolated and maintained cells in vitro. Initial experiments primarily focused on single-cell organisms, but over the decades, the field expanded to include mammalian and other cell types.
In 1950, George Beadle and Edward Tatum's research laid the groundwork for understanding gene function in cellular development. Further advancements followed throughout the 1960s, marked by the development of specific culture media tailored to optimize cell growth. The introduction of serum-free media in the 1980s marked a paradigm shift, allowing for more ethical and controlled experimentation by minimizing the reliance on animal products.
Today, cell culture is integral to biomedical research, drug formulation, and vaccine development. Its evolution reflects the ongoing quest for innovation and understanding within the scientific community.
Types of Cells Used in Culture
Understanding the types of cells used in culture is crucial for various applications within biotechnology and pharmaceuticals. Each cell type offers distinct advantages, making it necessary to choose the right cell line for specific research or production goals. This section aims to outline the most common types of cells utilized in cell culture, detailing their specific characteristics, benefits, and considerations.
Mammalian Cells
Mammalian cells are among the most prominent types of cells used in culture. Their significance lies primarily in their ability to accurately mimic human biological processes. These cells are often derived from various tissues, providing researchers with a versatile platform for studying human diseases, drug responses, and other biological phenomena.
- Commonly Used Cell Lines: Well-known cell lines like HeLa, CHO, and HepG2 are frequently utilized due to their high transfectability and resilience in culture. HeLa cells, for example, are immortalized human epithelial cells that are ideal for research in cancer biology.
- Benefits: They are capable of post-translational modifications that are similar to those found in human tissues. This makes them suitable for producing complex biopharmaceuticals, including monoclonal antibodies.
- Considerations: The complexity of mammalian cell cultures often requires specific growth media, temperature settings, and CO2 levels. The need for these controlled conditions adds layers of complexity to the culturing process, inherently demanding more investment in terms of time and resources.
Insect and Avian Cells
Insect and avian cells provide an alternative approach in cell culture, particularly in the field of biotechnology. Insect cells, such as those derived from Sf9 or Sf21 lines, are commonly employed for recombinant protein expression.
- Applications in Bioproduction: The baculovirus expression system, based on insect cells, has the ability to produce large quantities of proteins efficiently. This method is advantageous for proteins that require post-translational modifications that mimic those of mammalian cells.
- Advantages: Rapid growth rates and easy adaptability to culture conditions make these cells beneficial in various production scenarios. Furthermore, their usage often results in lower production costs compared to mammalian cells.
- Challenges: Limited understanding of their genetics and physiology compared to mammalian cells can impact robustness and predictability in experiments. Also, avian cells used in culture have specific requirements and may not always be adaptable to broader experimental needs.
Microbial Cells
Microbial cells, including bacteria and yeast, form the backbone of many industrial processes. They are utilized for various applications where speed, cost-effectiveness, and simplicity are valued.
- Common Microbial Types: Escherichia coli (E. coli) is a widely used bacterial cell line. Yeast cells, like Saccharomyces cerevisiae, are also popular for protein expression and fermentation.
- Benefits: Microbial cells reproduce rapidly and can grow in simple media, reducing production costs significantly. Their genetic manipulability allows for the production of a wide range of substances, from enzymes to biofuels.
- Limitations: The primary limitation of microbial cell systems is their inability to perform complex eukaryotic post-translational modifications. This restricts their use in producing certain biologically active compounds that require modifications found in higher organisms.
In summary, the choice of cell type in culture directly influences the outcomes of research and production efforts. Each cell type, whether from mammalian, insect, avian, or microbial origins, presents unique advantages and challenges that researchers must navigate to achieve their goals.
In summary, the choice of cell type in culture directly influences the outcomes of research and production efforts. Each cell type, whether from mammalian, insect, avian, or microbial origins, presents unique advantages and challenges that researchers must navigate to achieve their goals.
Cell Culture Techniques
Cell culture techniques play a vital role in the field of biotechnology, impacting various applications such as pharmaceuticals, tissue engineering, and cellular research. Understanding the nuances of these methodologies is essential for maximizing cell growth and productivity. Each technique offers unique benefits and challenges, which need to be considered in the context of specific research aims or product development.
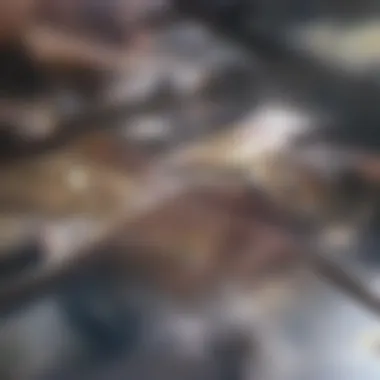
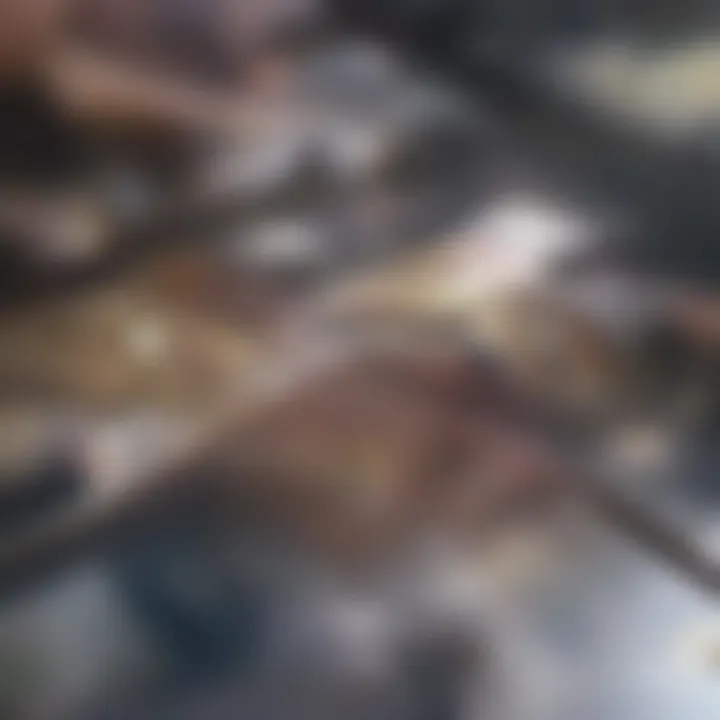
Adherent vs. Suspension Culturing
Adherent and suspension culturing are two primary methods for growing cells in laboratory settings.
- Adherent Culturing: In this method, cells are attached to a surface, often a culture dish. This mimics the cells' natural environment allowing them to grow and behave more like they would in vivo. The benefits of this technique include ease of observation and manipulation of the cells. However, it can be a limiting factor when scaling up production, as the surface area can restrict cell density.
- Suspension Culturing: Here, cells grow freely in a liquid medium. This method facilitates a higher cell density and is often preferred for large-scale production processes. Suspension culture is particularly advantageous for particular cell lines like CHO or hybridoma cells. Nevertheless, it requires specific conditions to maintain cell growth and viability, such as careful monitoring of nutrient levels.
Monolayer and Spheroid Culture
When discussing cell culture structures, monolayer and spheroid cultures stand out as two distinct approaches.
- Monolayer Culture: It involves spreading cells in a single layer on a culture dish. This type of culture is easy to manage and often allows for straightforward analysis of cell behavior. However, cells grown in a monolayer can have altered behaviors compared to those in a complex tissue structure.
- Spheroid Culture: This method, conversely, allows cells to grow in three-dimensional aggregates. Spheroids provide a more accurate representation of in vivo cell interactions and can better mimic the tumor microenvironment or tissue structures. One drawback is the difficulty in maintaining nutrient and oxygen diffusion in larger spheroids, which can affect the viability of inner cells.
3D Cell Culture Systems
3D cell culture systems represent a significant advancement in cell culture technology. In contrast to traditional two-dimensional cultures, 3D systems incorporate cells into a three-dimensional scaffold or matrix, allowing for more complex cell interactions and behaviors. This technique can be fundamental in evaluating drug responses and studying disease mechanisms.
The implications of this technology can be profound:
- Enhanced cell behavior: Cells in 3D culture models often exhibit increased functionality and greater resemblance to in vivo conditions compared to 2D cultures.
- Improved drug testing: By better mimicking physiological processes, 3D cultures allow for more predictive and accurate results in pharmacological studies.
- Tissue engineering applications: These systems are crucial for bioengineering tissues for regenerative medicine.
"Three-dimensional cell culture may prove essential for future advancements in both research and clinical applications."
"Three-dimensional cell culture may prove essential for future advancements in both research and clinical applications."
Overall, each cell culture technique has unique advantages and challenges that researchers must consider when planning experiments or scaling up production. From adherent to 3D culture systems, these methodologies offer a range of options to meet diverse scientific needs.
Preparation of Culture Media
The preparation of culture media is a fundamental aspect of cell culture production. The quality and composition of the media can greatly influence cell growth, metabolism, and overall health. Properly formulated culture media provides essential nutrients and a conducive environment for cells to thrive. Therefore, understanding the composition and the role of various elements in culture media becomes crucial for successful cell cultivation.
In this section, key elements will be discussed, along with considerations on how they contribute to the development of effective culture media. The efficiency of cell culture practices is largely determined by how well the media is tailored to meet the needs of specific cells, requiring careful attention to detail.
Essential Nutrients
Essential nutrients are the backbone of any culture media. They support cell metabolism, energy production, and cellular functions. Commonly used essential nutrients include:
- Amino Acids: They are vital for protein synthesis. For instance, L-glutamine is an important amino acid that serves as an energy source for cells.
- Vitamins: These compounds function as co-factors in metabolic pathways. Examples include B vitamins, which play roles in cell growth and division.
- Minerals: Elements like calcium, potassium, and magnesium are crucial for various cellular processes, including signaling and maintaining osmotic balance.
The synthesis of culture media often involves balancing these nutrients to ensure optimal conditions for cell growth. Care must be taken to source high-quality ingredients to avoid contamination and variability in results.
Serum and Serum-Free Media
Serum has historically been used in culture media as a source of hormones, growth factors, and additional nutrients. Fetal bovine serum (FBS) is one of the most commonly used serums. It is rich in growth-promoting factors, making it advantageous for many cell types. However, reliance on serum can lead to batch-to-batch variability and ethical considerations regarding animal welfare.
Serum-free media have been developed to address these concerns. These media are formulated to supply all necessary factors without using serum. They can be tailored to suit specific cell lines. For example, media for stem cells or hybridomas can require specific growth factors to promote desired characteristics. The advantages of serum-free media include:
- Reduced Contamination Risks: Serum can introduce viral or bacterial contaminants.
- Consistent Results: Formulated media lead to reduced variability in cell growth and behavior.
Choosing between serum and serum-free media requires careful consideration of the cell type and the intended application of the cultured cells.
Additives and Supplements
Additives and supplements serve to enhance the performance of culture media further. They may include:
- Growth Factors: These proteins can stimulate cellular proliferation and differentiation. Common examples are EGF (epidermal growth factor) and FGF (fibroblast growth factor).
- Antibiotics: Used to prevent bacterial contamination, it is vital to use them judiciously, as excessive use can lead to resistant strains of bacteria.
- Buffers: Maintaining pH is critical for cell viability. Sodium bicarbonate is often added as a buffering agent to stabilize pH levels.
When incorporating these additives, it’s essential to evaluate the specific needs of the cells being cultured. Too many supplements can lead to unforeseen alterations in cell behavior, affecting experimental outcomes.
In summary, the thoughtful preparation of culture media, including a focus on essential nutrients, the choice between serum and serum-free options, and strategic use of additives, plays a pivotal role in successful cell culture practices. Understanding these components helps in optimizing the growth conditions for various cell types, thus enhancing the efficiency and accuracy of cell culture production.
Environmental Conditions for Cell Culture
The environmental conditions for cell culture are essential to ensure healthy cell growth and productivity. This aspect involves several critical factors, including temperature, pH, gas exchange, humidity, and sterility. Each of these elements plays a fundamental role in providing a suitable environment for cells, which are sensitive to changes in external conditions. Therefore, understanding and controlling the environmental parameters are paramount for successful cell culture production and its applications in biotechnology.
Temperature and pH Control
Temperature control is vital in cell culture as different cell types have specific requirements. Most mammalian cells grow optimally at 37°C, while other cells, such as insect cells, may prefer lower temperatures. Maintaining the right temperature ensures metabolic processes proceed efficiently, affecting cell proliferation and viability. Fluctuations in temperature can lead to stress, resulting in altered gene expression and, subsequently, reduced cell function.
Moreover, pH is a critical factor. The ideal pH for culturing most cells generally ranges from 7.2 to 7.4. Deviations from this range can significantly impact cellular functions and lead to undesirable changes in metabolism or apoptosis. To regulate pH, buffering agents are commonly added to the culture media. Frequent monitoring of both temperature and pH is necessary, and systems such as CO2 incubators help maintain these parameters consistently.
Gas Exchange and Humidity
Gas exchange is another crucial aspect of cell culture environments. Cells require oxygen for respiration and metabolic activities, while they produce carbon dioxide as a metabolic byproduct. Inadequate oxygen levels can hinder cell growth and lead to cell death. To facilitate sufficient gas exchange, it is important to use culture vessels that allow for adequate air circulation without causing contamination.
Humidity levels must also be controlled. Excessive evaporation from culture media can cause concentration of nutrients and alter osmotic balance negatively. Typically, a humidity level around 80% is maintained within incubators to prevent media evaporation. Techniques such as using sealed culture plates or keeping water in the incubator can help maintain these humidity levels.
Sterility and Contamination Prevention
Maintaining sterility is one of the greatest challenges in cell culture. Contaminants such as bacteria, fungi, and mycoplasma can quickly compromise cell cultures, leading to inaccurate results and loss of cell batches. Therefore, strict aseptic techniques are required during all processes, from preparation to handling. This includes using sterile equipment, working in laminar flow hoods, and routinely cleaning surfaces with disinfectants.
Also, monitoring cell cultures for signs of contamination is essential. Regular observation through microscopy can help detect any irregular changes in cell morphology or growth patterns. Implementing quality control measures can protect cell cultures against contaminants and ensure robust experimental outcomes.
Proper environmental controls not only protect the integrity of cell cultures but also improve reproducibility in experiments, a crucial aspect in scientific research and biotechnological applications.
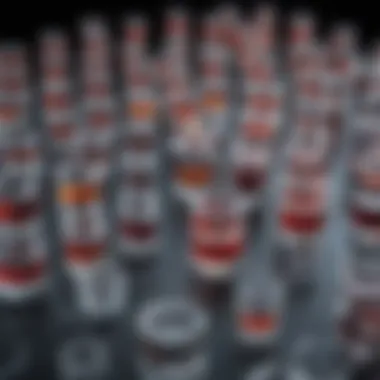
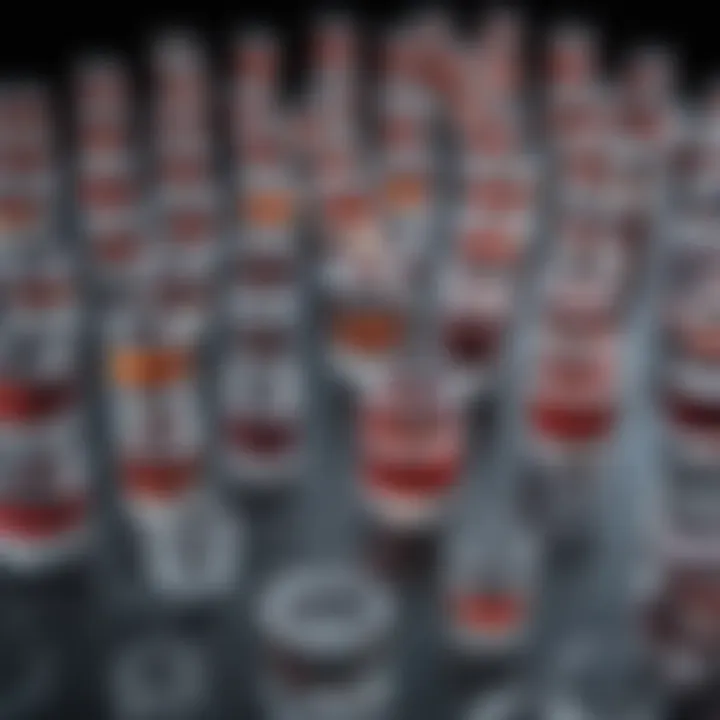
Proper environmental controls not only protect the integrity of cell cultures but also improve reproducibility in experiments, a crucial aspect in scientific research and biotechnological applications.
Understanding and implementing precise environmental conditions is crucial for anyone involved in cell culture production. Each of these factors must be harmonized to create the ideal conditions for cells to thrive, ultimately enhancing the reliability of experimental results and the efficacy of applications in the field.
Scaling Up Cell Culture Production
Scaling up cell culture production is an essential aspect of biotechnological advancements. The importance of this topic stems from the need to meet the increasing demand for therapeutic products, vaccines, and biomaterials. As cell culture technology evolves, it is crucial to enhance production efficiency while ensuring the viability and functionality of cultured cells.
Batch vs. Continuous Systems
When scaling up, one of the primary considerations is the choice between batch and continuous systems. In batch culture, cells are grown in a fixed volume of media until they reach a desired density, after which the culture is harvested. This method usually offers simplicity and reduced risk of contamination. However, it may also result in inconsistent yields.
On the other hand, continuous culture systems keep cells in an exponential growth phase by constantly adding fresh media and removing waste and spent media. This setup can enhance productivity and provide a more consistent product output over time. Continuous systems are more complex and may require sophisticated monitoring and control techniques.
Bioreactor Designs
Bioreactor design is another crucial factor when scaling up. Bioreactors come in various configurations, including stirred-tank, airlift, and wave bioreactors. Each design has unique advantages and applications.
- Stirred-tank bioreactors are the most commonly used. They provide uniform mixing and improved oxygen transfer, making them suitable for aerobic cell cultures.
- Airlift bioreactors utilize gas bubbles to circulate the media, reducing shear stress on sensitive cells. This design is typically used for bacterial and certain mammalian cultures.
- Wave bioreactors employ rocking motion to create fluid motion. This type is particularly beneficial for growing cells in suspension, such as stem cells, since it minimizes shear forces.
Selecting the right bioreactor is essential. The design directly influences the cellular environment, nutrient availability, and overall cultivation efficiency.
Automation in Cell Culture
Automation presents significant benefits in scaling up cell culture production. Automated systems can oversee critical processes like media preparation, cell monitoring, and environmental controls. The implementation of automation enhances reproducibility and speed, reducing the potential for human error.
- Robotic platforms are often used for high-throughput screening and cell handling.
- Automated control systems are essential for maintaining optimal conditions during cell growth, such as temperature, pH, and dissolved oxygen.
- Data collection and analysis can also be automated, allowing for real-time adjustments to maintain the best conditions for cell growth.
Automation not only streamlines the process but also makes larger-scale productions feasible, enabling facilities to respond swiftly to market demands without compromising quality.
"Scaling up cell culture production is not merely about increasing volume; it is about ensuring consistency and quality."
"Scaling up cell culture production is not merely about increasing volume; it is about ensuring consistency and quality."
Regulatory Considerations
Regulatory considerations play a crucial role in cell culture production. They ensure products meet standards for safety, efficacy, and quality. In an industry that relies heavily on biological materials, maintaining these standards is essential for public health and trust. Compliance with regulations prevents contamination, ensures the integrity of biological samples, and promotes reproducibility in research and production.
Good Manufacturing Practices (GMP)
Good Manufacturing Practices, or GMP, establish guidelines that manufacturers must follow to ensure products are consistently produced and controlled. These regulations govern all aspects of production from the raw materials to the finished product. By adhering to GMP, organizations help to mitigate risks.
- Quality Control: Ensuring the quality of starting materials and monitoring processes is vital.
- Personnel Training: Staff must be adequately trained to carry out procedures consistently.
- Facility Standards: Production environments must meet specific cleanliness and operational standards.
- Documentation: Proper documentation is essential for tracking processes and ensures accountability.
These measures not only enhance product safety but also improve the reliability of research data.
Ethical Concerns
Ethical concerns in cell culture production are paramount, especially when human tissues or cells are involved. Questions arise about consent, accessibility, and the potential exploitation of biological resources. Researchers and practitioners in cell culture must navigate these ethical dilemmas carefully. Common dilemmas include:
- The necessity of informed consent from donors.
- Considerations surrounding the use of embryonic stem cells.
- The need for fair access to therapies arising from cell culture innovations.
These points emphasize the need for a robust ethical framework guiding cell culture practices, ensuring respect for human dignity and life.
Quality Control Measurements
Quality control measurements are integral to the reliability of cell culture production. They encompass various strategies to ensure that cell cultures are free from contaminants and that they function as intended. Key measurements include:
- Bioburden Testing: This involves assessing the viable microorganism load in a culture.
- Contamination Detection: Regular testing helps identify bacterial, fungal, or viral contamination.
- Cell Viability Assays: These assays measure the health of the cells in culture, ensuring they are growing and functioning correctly.
- Performance Metrics: Monitoring growth rates and differentiation capabilities of cells.
Implementing stringent quality control protocols safeguards the integrity of research findings and product efficacy.
"Regulatory considerations are not just a box to check; they are fundamental to the integrity of the biotechnology field."
"Regulatory considerations are not just a box to check; they are fundamental to the integrity of the biotechnology field."
In summary, regulatory considerations in cell culture production encompass GMP, ethical concerns, and quality control measurements. Together, these elements ensure that cell culture production is performed responsibly and effectively.
Applications of Cell Culture Production
Applications of cell culture production are critical in biotechnology and medicine. This methodology allows scientists to grow and manipulate cells in controlled environments. The significance of understanding how to effectively apply cell culture technologies can’t be understated. It opens avenues for innovations that drive advancements in research and industry.
Pharmaceutical Developments
Cell culture is instrumental in pharmaceutical development. It supports the creation of drug candidates by providing a platform for testing drug efficacy and toxicity. For example, various cell lines, such as HeLa and CHO cells, are used in the production of therapeutic proteins and monoclonal antibodies. The controlled setting of cell cultures minimizes variables. This enhances the reliability of results and aids scientists in understanding drug interactions at the cellular level.
Moreover, in vitro testing often reduces the need for animal testing, aligning with ethical considerations in the development of new drugs.
Vaccine Production
The role of cell culture in vaccine production is profound. Traditionally, vaccines were produced using live attenuated or inactivated pathogens, often requiring the use of live animals. Advances in cell culture techniques have shifted many vaccine production processes to using cultured cells.
For instance, the use of Vero cells has been pivotal in developing vaccines for diseases like polio and rabies. These cells provide a suitable environment for viral replication, which is essential in vaccine formulation. By utilizing cell-based systems, the production process is more efficient and scalable. This has been especially crucial during public health emergencies, such as the COVID-19 pandemic, where rapid vaccine development was necessary.
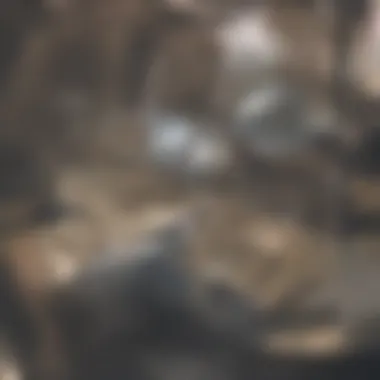
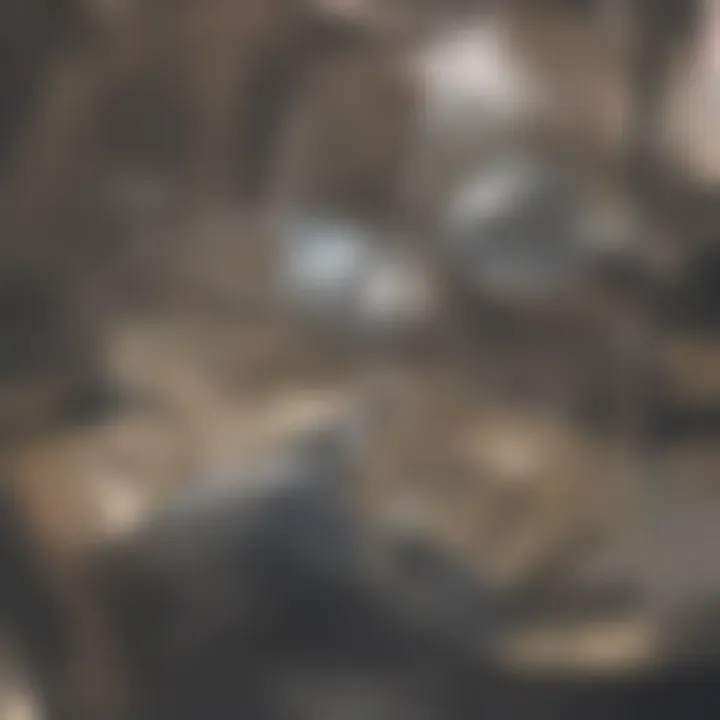
Tissue Engineering
Tissue engineering leverages cell culture to develop biological substitutes that restore, maintain, or improve tissue function. By cultivating cells in three-dimensional matrices, researchers can create functional tissue constructs. These constructs are critical in regenerative medicine and surgical applications.
For instance, stem cells are frequently used to create cardiac tissues for repairing heart injuries. The ability to culture cells under specific conditions allows for the manipulation of cellular behaviors, leading to advancements in personalized medicine. Such tissue engineering applications provide exciting prospects for treating degenerative diseases and injuries.
"Cell culture techniques form the foundation of many modern applications in life sciences, driving forward research in critical areas such as drug development, vaccine production, and tissue engineering."
"Cell culture techniques form the foundation of many modern applications in life sciences, driving forward research in critical areas such as drug development, vaccine production, and tissue engineering."
Challenges and Limitations
Understanding the challenges and limitations in cell culture production is essential for researchers and professionals in biotechnology and pharmaceuticals. These issues can affect the quality of research outcomes and the feasibility of scaling up production. Addressing these challenges ensures that cell culture techniques remain robust, reliable, and continue to advance in efficiency.
Cell Line Stability
Cell line stability refers to the consistency of cell behavior over time. This is crucial because changes in cell characteristics can lead to variations in experimental results. For example, a cell line may change expression of certain genes or lose its ability to proliferate, which can significantly impact studies on drug efficacy or toxicity.
Maintaining stability involves careful selection of cell lines with proven track records. Regular monitoring of cell characteristics during culture is also vital. Techniques such as genetic profiling can be employed to assess any alterations in the cells. Furthermore, proper storage conditions, such as freezing cells in liquid nitrogen, can help preserve their stability long-term.
Contamination and Its Impact
Contamination is a major concern in cell culture as it can compromise the integrity of the results. Sources of contamination include microorganisms like bacteria, fungi, or viruses, which can proliferate under culture conditions. Even a small number of contaminating cells can overwhelm the culture system, leading to inaccurate data and wasted resources.
To mitigate contamination risks, stringent aseptic techniques must be followed. Regular monitoring of cell cultures for signs of contamination is essential. Utilizing solutions like antibiotic supplements can also help, although they should be used cautiously as they may induce resistance. Regular cleaning of work surfaces and thorough sterilization of equipment are basic yet effective strategies in preventing contamination.
Economic Constraints
Economic constraints pose a significant limitation to cell culture production. The costs involved in maintaining cell cultures can be high. This includes expenditures on supplies like growth media, reagents, and equipment. In addition, costs associated with skilled labor to manage and monitor cultures can also add up.
To address economic limitations, organizations may consider optimizing their protocols. This might involve using less expensive media formulations or employing bioreactors that enhance yield without proportionate increases in cost. Furthermore, developing partnerships with suppliers for bulk purchasing could provide significant savings.
"Cost-effectiveness is vital for sustainable cell culture practices, allowing researchers to maximize output while minimizing expenses."
"Cost-effectiveness is vital for sustainable cell culture practices, allowing researchers to maximize output while minimizing expenses."
By navigating these challenges effectively, researchers can enhance the reliability and applicability of cell culture in various fields, including drug development and vaccine production.
Future Directions in Cell Culture Production
Cell culture production has matured over decades, yet its future is pivotal to advancing biotechnology and pharmaceuticals. Innovations drive efficiency, effectiveness, and the quality of cell-based products. The direction in which cell culture evolves will shape critical areas such as drug development, personalized medicine, and sustainability practices in the industry.
Innovative Technologies
The integration of advanced technologies stands to revolutionize cell culture production. Automation is reshaping workflows to ensure precision and scale. Today’s automated systems can handle tasks from media preparation to cell harvesting with minimal human intervention. Moreover, bioreactors equipped with real-time monitoring capabilities enhance the quality of cell growth by adjusting conditions dynamically.
Technologies like CRISPR are enabling targeted modifications of cell lines, offering researchers the ability to develop more specialized cells for therapeutic uses. Advances in microfluidics also hold promise, facilitating the transport and manipulation of cells with increased efficiency, thus allowing more complex experiments on a smaller scale.
Personalized Medicine Approaches
The push toward personalized medicine emphasizes the need for custom solutions in treatment protocols. Cell culture production plays a vital role in tailoring therapies to individual patient needs. Individualized drugs, developed through patient-specific cell lines, allow for a more precise and effective treatment approach.
This methodology can lead to better clinical outcomes and improve the efficiency of drug discovery processes. Furthermore, organ-on-a-chip technologies are gaining traction, allowing researchers to evaluate responses to drugs using cells from specific patients, thereby offering unique insights into how treatments may work in real-world scenarios.
Sustainability in Cell Culture Practices
Sustainability is an increasing concern within cell culture production. The consumption of resources can be significant, necessitating innovative strategies to minimize waste and reduce environmental footprints. Practices such as using plant-based alternatives for serum in culture media are gaining attention. These strategies not only improve sustainability but can also enhance the reproducibility of experiments.
Moreover, recycling and reusing materials in production processes become crucial. The adoption of green chemistry principles assists in reducing harmful impacts on the environment, creating a pathway for a more sustainable biotech future. As regulations tighten over environmental concerns, the industry must align with these expectations.
"The future of cell culture production is not just about innovation in methodologies; it is also about integrating sustainability into every aspect of the process."
"The future of cell culture production is not just about innovation in methodologies; it is also about integrating sustainability into every aspect of the process."
End
First, it reinforces the significance of cell culture in modern biotechnology and its applications in various fields, from pharmaceuticals to regenerative medicine. The production methods discussed help researchers optimize their processes and ensure reliable outcomes. Key factors such as cell type selection, media preparation, and environmental conditions are critical to achieving success in cell culture.
In addition, the conclusion reflects on the challenges and limitations faced in cell culture production. Recognizing these obstacles aids in developing strategic solutions, facilitating progress in research and applications.
Lastly, the discussion of future directions highlights the trends and innovations poised to shape the industry. This emphasizes the evolving nature of cell culture practices, urging stakeholders to keep abreast of advancements in technology and methodology.
Summary of Key Points
- Importance: Cell culture is fundamental for biotechnology and pharmaceutical applications.
- Techniques: Various culturing techniques enhance cell growth and production.
- Media: Proper preparation of culture media ensures optimal conditions for cell health.
- Environmental Control: Maintaining appropriate physical conditions is essential for success.
- Regulatory Aspects: Adhering to regulations is crucial in ensuring quality and safety in cell production.
- Challenges: Recognizing cell line stability issues and contamination risks is vital for improvement.
- Future Directions: Embracing new technologies and sustainability practices will define the next phase of cell culture production.
Final Thoughts on Impact
The influence of cell culture production extends well beyond laboratory settings. It plays a pivotal role in the development of lifesaving medicines, vaccines, and therapeutic strategies. Ultimately, its impact is measured in terms of enhanced human health and the advancement of scientific knowledge.
Investment in research and innovation within this field fosters resilience against emerging health challenges. As the understanding of cell biology expands, so do the possibilities for improved and more efficient production methods.
In summary, an informed viewpoint on the intricate aspects of cell culture production will empower researchers and professionals alike to make strides in their respective domains. Collaborations and knowledge sharing will further catalyze advancements, paving the way for future successes in biotechnology.
"Cell culture is not just a technique; it is the backbone of advancements in biomedicine and technology. Its evolution reflects the progress of science itself."
"Cell culture is not just a technique; it is the backbone of advancements in biomedicine and technology. Its evolution reflects the progress of science itself."
The ability to adapt and innovate in response to challenges will define the future landscape of cell culture production.