Compressed Tissue: Mechanics and Applications Explained
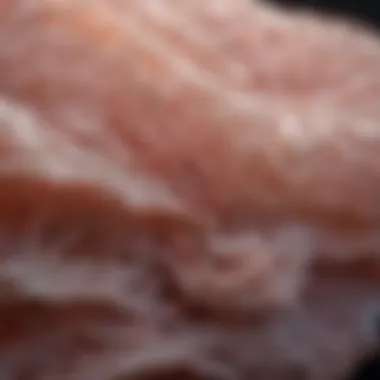
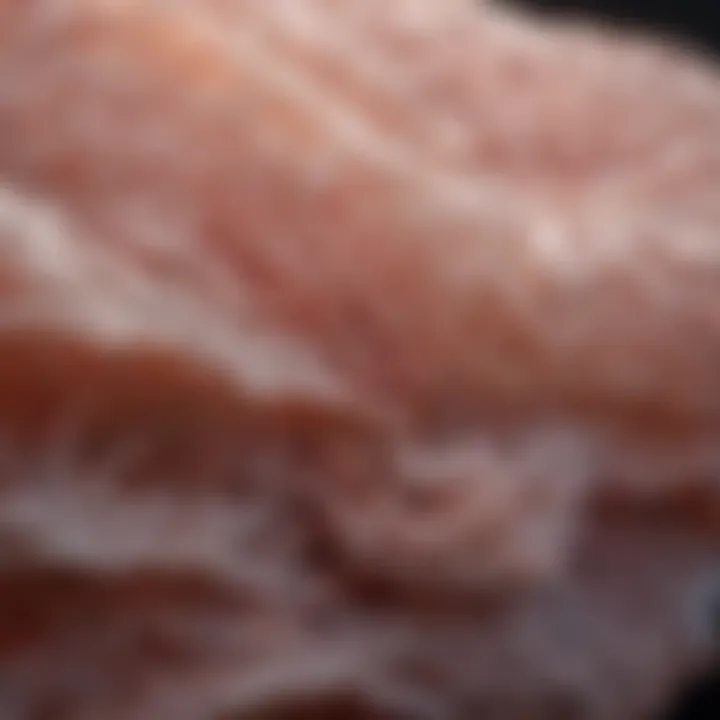
Intro
Compressed tissue plays a pivotal role in various scientific fields, including biology and medicine. By examining its unique mechanics and applications, we gain valuable insights into both fundamental biological processes and innovative medical technologies. Understanding this topic is essential for researchers and professionals alike, as it paves the way for advancements in tissue engineering, regenerative medicine, and other interdisciplinary domains.
Article Overview
Purpose of the Article
The main aim of this article is to explore the intricacies of compressed tissue. We will analyze its formation, mechanisms, and the implications it has across different sectors. This piece is designed to serve as a comprehensive guide that equips the reader with a deep understanding of compressed tissue, its properties, and potential innovations.
Relevance to Multiple Disciplines
Compressed tissue finds its relevance in a mixture of disciplines. In biology, it aids in understanding cellular structures and functionalities. In medicine, the applications range from organ transplantation to wound healing. The intersections of these fields highlight the importance of studying compressed tissue, facilitating collaborations that can lead to groundbreaking discoveries.
Research Background
Historical Context
The exploration of compressed tissue has evolved significantly over time. Early studies focused on basic structural analysis, while contemporary research dives into its functional applications. Historically, figures such as Robert Hooke laid the groundwork for understanding cellular compression in plants, but it was not until the development of advanced imaging techniques that the complexities of compressed tissue in animals became apparent.
Key Concepts and Definitions
To effectively discuss compressed tissue, it is vital to clarify its key concepts:
- Compressed Tissue: A type of biological tissue that has undergone a reduction in volume due to external pressure or forces, often affecting its structural integrity and functionality.
- Mechanics: The branch of physics that deals with the behavior of objects when subjected to forces. In the context of compressed tissue, it focuses on how tissue responds to stress and strain.
- Applications: The practical uses of compressed tissue in real-world scenarios, especially within biomedical engineering and clinical settings.
Understanding these foundational concepts is crucial as we navigate the complexities of compressed tissue, particularly in its application and innovation potential.
"The study of compressed tissue is not merely academic; it informs practical solutions to critical medical challenges and offers hope for future advancements."
"The study of compressed tissue is not merely academic; it informs practical solutions to critical medical challenges and offers hope for future advancements."
In the following sections, we will delve deeper into the properties and applications of compressed tissue, helping to illuminate its significance in both research and practical applications.
Preface to Compressed Tissue
Compressed tissue is critical in both biological and medical science. Understanding its mechanics opens doors to innovative applications and deeper insights into how living organisms function. The study of compressed tissue is not merely an academic endeavor; it has direct implications for areas such as tissue engineering, surgical practices, and material science. This introduction focuses on the fundamental aspects of compressed tissue and sets the stage for more detailed exploration throughout the article.
Definition and Understanding
Compressed tissue refers to biological tissues that have undergone a process of compaction, resulting in altered physical and mechanical properties. This phenomenon can occur naturally or can be induced by external forces. In terms of definition, it encompasses various types of tissues including connective tissue, muscle tissue, and others that can be deformed by stress.
The significance of compressed tissue lies in its mechanical behavior. These tissues exhibit unique features like elasticity and strength, allowing them to withstand compressive forces. Additionally, compressed tissue plays a role in biological functions, such as nutrient transport and waste removal, highlighting its relevance in both health and disease.
Historical Context
The study of compressed tissue is rooted in both biology and engineering. Historically, early observations of tissue properties date back to the 19th century when scientists begain to explore the structural integrity of tissues under different forces. Research advancements over the years have expanded our understanding of how tissues respond to compression.
For instance, researchers like Thomas Young and Robert Hooke contributed foundational concepts in elasticity. Their principles can be applied to compressed tissues, elucidating their behavior under stress. As biomedical technologies evolved, so too did the methodologies for studying compressed tissues, paving the way for modern applications in regenerative medicine and bioengineering.
The future of compressed tissue research is likely to be influenced by ongoing innovations in technology and materials science, further bridging the gap between biological structures and practical applications. This historical context emphasizes the importance of interdisciplinary approaches, essential for advancing our understanding of compressed tissue.
Properties of Compressed Tissue
Understanding the properties of compressed tissue is essential for its applications in diverse fields, notably medicine and material science. The properties help in determining how compressed tissue behaves under different conditions, influencing both design and implementation. Key properties include mechanical aspects such as elasticity, viscosity, and strength, along with biological factors like cellular composition and metabolic activity. Each property plays a pivotal role in the efficacy and usability of compressed tissues, making it imperative to delve into their specific elements.
Mechanical Properties
Elasticity
Elasticity refers to the ability of compressed tissue to return to its original shape after deformation. This characteristic is crucial for applications such as surgical implants where tissue extents and contracts during bodily movements. High elasticity ensures that implants can adapt dynamically, reducing the risk of injury or discomfort. A major benefit of elasticity is its capacity to absorb shocks and distribute stress, which is particularly advantageous in biological settings.
One unique feature of elasticity in compressed tissue is its dependence on the arrangement and composition of the cells. This can result in varying elastic responses when subjected to mechanical stress. However, a disadvantage may arise from low elastic tissues, as they may lead to complications like stiffness or inability to support dynamic movements.
Viscosity
Viscosity describes the resistance of compressed tissue to flow or deformation under stress. It is an important property in understanding how tissue behaves over time and under various loads. A key characteristic of viscosity is its impact on the healing process; more viscous tissues can slow down fluid movement in the body, thereby influencing nutrient delivery and waste removal.
This property is beneficial due to its role in ensuring stability, especially during the initial stages of tissue grafting. However, a drawback of high viscosity may be seen in situations where rapid fluid movement is necessary, potentially leading to inefficiencies in biological functions.
Strength

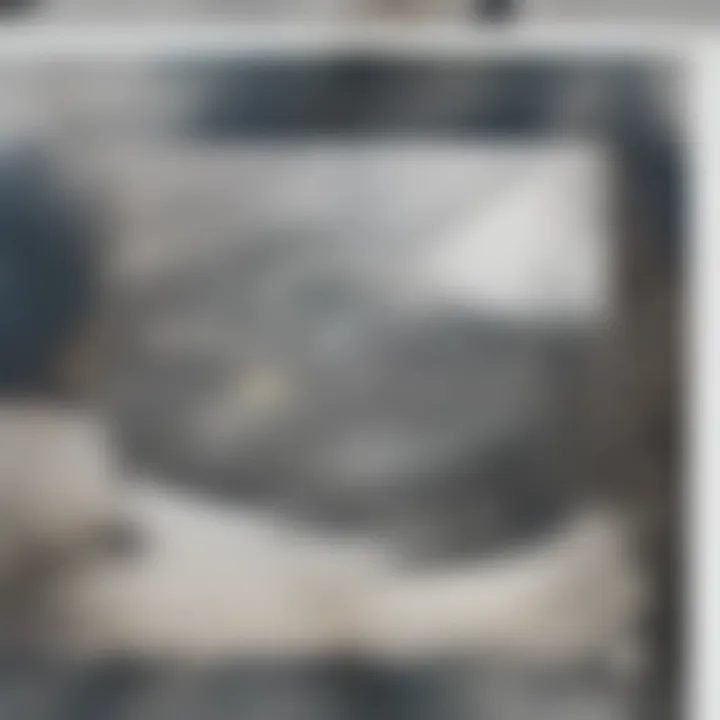
Strength pertains to the maximum stress a material can withstand before failure. In compressed tissue, this property heavily influences its application in load-bearing scenarios like bone implants. A key characteristic is an ability to sustain weight without deformation, crucial in maintaining structural integrity.
The unique feature of strength in compressed tissue attributes to its structural design, perfectly balancing tensile and compressive forces. Its high strength is beneficial, especially for implants subjected to constant loads. However, strong materials might pose challenges in their integration with softer adjacent tissues, risking rejection or inadequate healing.
Biological Properties
Cellular Composition
Cellular composition refers to the types and distributions of cells within compressed tissue. This aspect is vital as it influences both mechanical properties and the tissue's biological functions. A significant characteristic is the variety of cell types present, which can enhance functionality like reparative processes in tissue engineering.
Diverse cellular compositions are advantageous, as they can be designed to mimic natural tissues more closely. This customized design leads to better integration and performance in medical applications. However, this complexity may also present challenges in ensuring the consistency and predictability of tissue behavior, leading to variability in outcomes.
Metabolic Activity
Metabolic activity indicates the level of biochemical processes occurring within the tissue, influencing its overall viability and functionality. Key here is the role of metabolic processes in tissue growth and repair. High metabolic activity can facilitate faster healing, which is a critical aspect of regenerative medicine.
The unique feature of metabolic activity links directly to the health and sustainability of the tissue in question. While higher metabolic rates are generally beneficial, they may lead to exhaustion of necessary resources if not appropriately balanced, impacting overall tissue viability.
Understanding the properties of compressed tissue offers insights into its applications and limitations, guiding advancements in both medical and material disciplines.
Understanding the properties of compressed tissue offers insights into its applications and limitations, guiding advancements in both medical and material disciplines.
Mechanisms of Compression
The understanding of compressed tissue is incomplete without analyzing the mechanisms of compression. This section delves into the essential physical and chemical processes that contribute to the behavior and characteristics of compressed tissue. A thorough comprehension of these mechanisms not only enhances the application of compressed tissue in various fields but also informs future research directions. Exploring these principles offers valuable insights for professionals and researchers alike, leading to innovative approaches and improved outcomes in tissue-related studies.
Physical Forces
Physical forces are fundamental to how compressed tissue behaves under various conditions. These forces include tension, compression, and shear stress, which each play distinct roles in influencing the structure and integrity of tissues. In biological contexts, these forces often govern the way tissues grow, heal, and respond to external stimuli.
- Tension occurs when forces pull apart the tissue. This can stimulate growth and adaptation in living organisms.
- Compression, on the other hand, involves forces pushing together the tissue. It can lead to changes in cellular organization and function.
- Shear stress arises from layers of the tissue sliding past one another, affecting how cells orientate and communicate.
In engineering contexts, understanding these forces allows for the development of better biomechanical devices and artificial tissues that require precise control of mechanical environments. The interactions between different types of forces also highlight how various tissues can be optimized in clinical applications.
Chemical Interactions
The role of chemical interactions in the compression of tissues is equally important. The biochemical components within tissues, including proteins, lipids, and carbohydrates, directly influence the mechanical properties of compressed tissues. When tissues are subjected to compression, various biochemical reactions occur that affect cell signaling, metabolism, and structural integrity.
These chemical factors can be categorized as:
- Covalent bonds that provide structural stability to proteins and contribute to tissue resilience.
- Hydrogen bonds which, although weaker, facilitate interactions between various molecules, impacting the elasticity of tissue.
- Ionic interactions that can alter tissue response to mechanical stress, modulating cellular behavior.
Understanding these chemical processes allows for improved drug delivery systems and tissue engineering strategies. By manipulating the chemical environment, researchers can enhance the efficacy of therapies that utilize compressed tissues.
"The intricate relationship between physical forces and chemical interactions is key to unlocking the full potential of compressed tissue in both biological and material sciences."
"The intricate relationship between physical forces and chemical interactions is key to unlocking the full potential of compressed tissue in both biological and material sciences."
In summary, both physical forces and chemical interactions play crucial roles in the mechanisms of compression. They contribute directly to the functionality and application of compressed tissue in various domains. Their interplay is fundamental for advancements in research and practical applications.
Applications in Medicine
The exploration of compressed tissue in the medical field demonstrates its profound relevance and versatility. There are two primary areas where compressed tissue plays a crucial role: tissue engineering and surgical practices. Both applications exhibit distinct characteristics and offer valuable benefits that address specific medical challenges.
Tissue Engineering
Scaffold Design
Scaffold design is a significant aspect of tissue engineering, ensuring that new tissue mimics the behavior and properties of natural tissue. The main characteristic of scaffold design lies in its ability to provide a supportive structure for cell growth. This characteristic makes it a beneficial choice for promoting tissue regeneration.
The unique feature of scaffolds is their porosity. A well-designed scaffold allows nutrients and oxygen to reach the cells, which is critical for their survival and function. The scaffold can be composed of various materials, including biopolymers like chitosan or gelatin. These materials have distinct advantages, including biocompatibility and the capacity to facilitate cellular adhesion. However, challenges exist, such as ensuring long-term stability and functionality within the body.
Regenerative Medicine
Regenerative medicine depends heavily on compressed tissue to restore and enhance the natural healing processes of the body. A key characteristic of regenerative medicine is its focus on stimulating the body’s own repair mechanisms. This approach is a popular choice as it can lead to more effective and longer-lasting results.
A unique feature of regenerative medicine is its application in stem cell therapy. This method holds promise for replacing or repairing damaged tissues. Some advantages include the ability to target specific issues and the potential for personalized treatments. Nevertheless, concerns surround ethical implications and the complexity of stem cell handling that must be addressed to enhance patient safety and acceptance.
Surgical Practices
Compression Bandaging
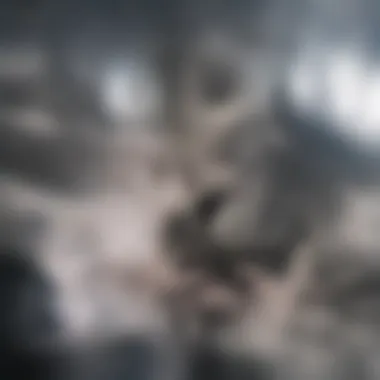
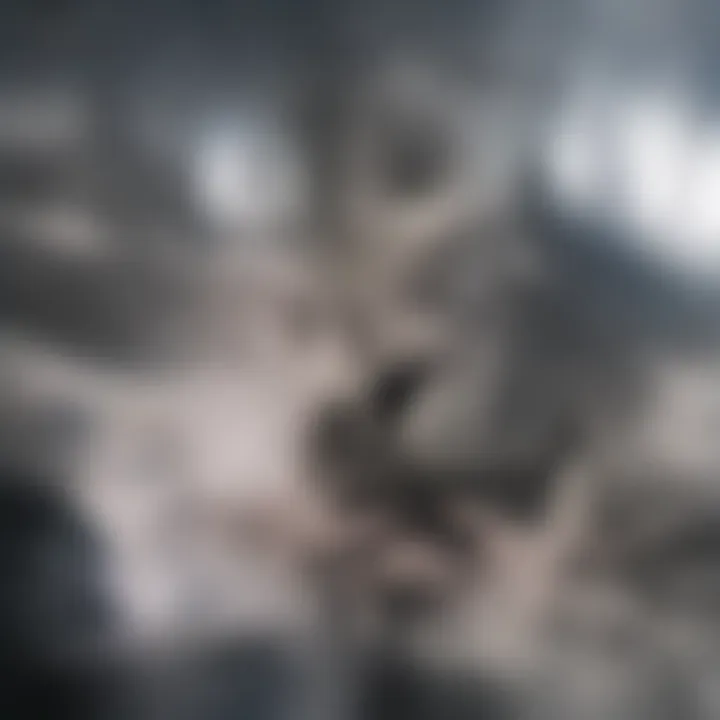
Compression bandaging is another practical application of compressed tissue in the surgical field. This technique applies pressure to the affected area to control swelling and enhance recovery outcomes. A key characteristic of compression bandaging is its capacity to reduce hematoma formation post-surgery. This makes it a beneficial choice for wound management and healing.
The unique feature of compression bandaging is its adjustable nature. Bandages can be modified in terms of tightness and material to suit various patient needs. Key advantages include improved blood circulation and reduced pain. However, it can also lead to issues like discomfort or skin irritation if improperly applied.
Post-Surgical Recovery
Post-surgical recovery efforts increasingly integrate approaches involving compressed tissue. This aspect aims to enhance the overall healing process by utilizing techniques that align with biological responses to surgery. The main characteristic of these efforts is the focus on minimizing complications and promoting faster recovery, making it a beneficial choice within medical practices.
A unique feature of post-surgical recovery strategies includes tailored rehabilitation programs. These programs can adapt exercises and compression therapy to the patient’s specific needs for optimal recovery. Advantages of this method include improved patient satisfaction and shorter hospital stays. However, monitoring patient responses is necessary to avoid adverse effects during recovery.
Applications in Material Science
Compressed tissue plays a critical role in material science, offering insights into the development of advanced materials with unique properties. Its application is particularly important in enhancing the performance of materials used in various industries. The significance of understanding compressed tissue in this context lies in its potential to inform the design and synthesis of composite materials that are tailored to meet specific requirements.
Composite Materials
Composite materials are engineered materials made from two or more constituent materials. The combination leads to improved properties that are unavailable in the individual components.
Strengthening Techniques
Strengthening techniques involve methods used to enhance the mechanical properties of composite materials. Techniques such as fiber reinforcement and layering are common in this field. One key characteristic of these methods is that they increase tensile strength while maintaining lightweight properties. This makes them beneficial for a range of applications, especially in aerospace and automotive industries.
A unique feature of strengthening techniques is the ability to optimize performance by adjusting material properties according to specific loads and stresses.
Advantages of incorporating strengthening techniques include:
- Improved load-bearing capacity.
- Greater resilience to impact.
- Enhanced durability in challenging environments.
However, some disadvantages can include complexity in manufacturing and potential costs associated with advanced materials.
Durability Testing
Durability testing is significant for assessing how well materials withstand wear, pressure, or damage over time. In the context of compressed tissue, understanding durability is essential for predicting the lifespan of materials under various conditions. The primary characteristic of durability testing is its ability to simulate real-world conditions in a controlled setting, offering reliable data on material performance.
This testing is particularly popular because it helps to prevent failures in practical applications, thus protecting investments in material development.
Unique features of durability testing include:
- Long-term performance assessment.
- Insights into material behavior at different temperatures and mechanical loads.
Advantages of durability testing are clear:
- Provides critical data for quality assurance.
- Helps in refining design choices for better performance.
On the downside, the process can be time-consuming and expensive, especially for extensive testing protocols.
Innovative Manufacturing Processes
The continued evolution of manufacturing processes is fundamental in the application of compressed tissue in material science. Innovative techniques such as additive manufacturing and advanced molding processes have revolutionized how composite materials are produced. These methods allow greater control over material properties, enabling the creation of parts with complex geometries and optimized characteristics.
Furthermore, the integration of compressed tissue in material science applications can lead to enhanced sustainability. By employing eco-friendly materials and processes, manufacturers can contribute to reducing environmental impacts. Understanding these processes provides a pathway for future advancements, emphasizing the importance of ongoing research in this field.
Compressed Tissue in Ecology
The study of compressed tissue in ecology reveals its vital role in maintaining and enhancing ecological balance. Understanding this aspect is crucial for assessing the benefits and challenges posed by the interaction of compressed tissue with various ecological factors. This section will explore the effects of compressed tissue on biodiversity, focusing primarily on habitat formation and species interactions, as well as its significance in carbon sequestration.
Impact on Biodiversity
Habitat Formation
Habitat formation is a critical aspect of how compressed tissues influence ecosystems. Compressed tissues often create unique physical structures that serve as shelters or breeding grounds for various species. These structures can significantly modify the environment. An important characteristic of habitat formation is its ability to provide stability in soils, which encourages the growth of vegetation that supports fauna.
The unique feature of habitat formation through compressed tissue lies in its capability to retain moisture. Moisture retention leads to enhanced plant growth, which in turn supports diverse animal communities. The advantage of such formations is apparent in regions where moisture is scarce, making them a beneficial choice for species struggling to survive in harsher climates.
However, habitat formation can also present challenges. If compressed tissues are too dense, they can limit root penetration, impeding some plant species' growth. This limitation may affect the overall diversity of habitats. Hence, the balance between compression strength and plant growth is vital for maintaining biodiversity.
Species Interactions
The interactions between species in environments influenced by compressed tissue are complex and significant. Species interactions reflect the degree to which different organisms rely on each other for survival and growth. A key characteristic here is the interconnectedness of plant and animal species that depend on the habitats formed by compressed tissue.
One unique feature of species interactions in these contexts is mutualism, where different species provide benefits to each other. For example, plants growing in compressed soils can offer shelter and food to various insects, which may, in turn, facilitate pollination. This relationship proves advantageous in promoting ecological resilience.
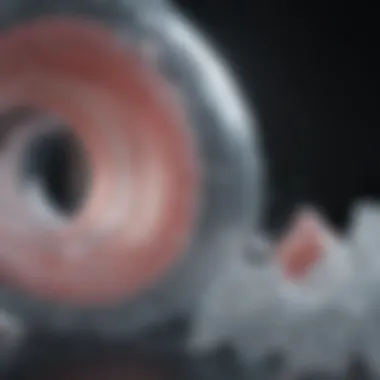
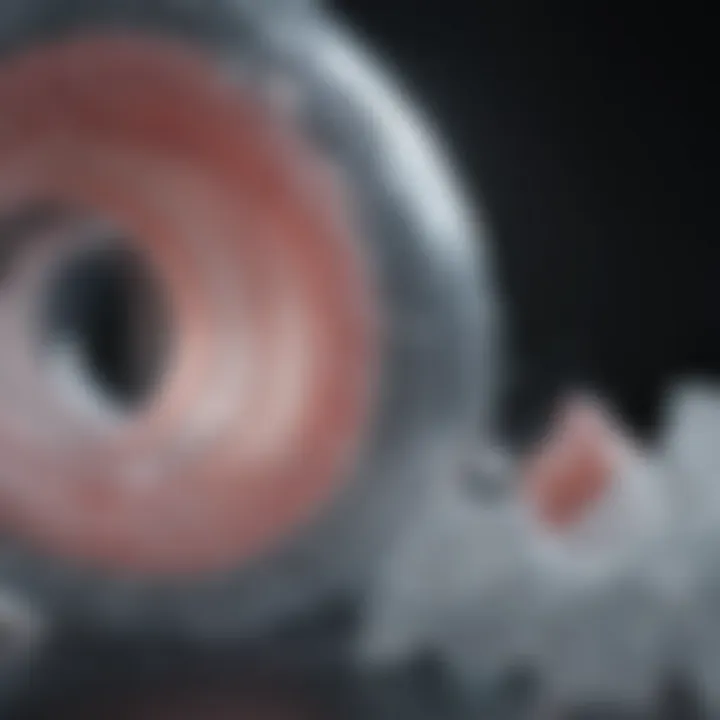
The challenge in studying species interactions stems from the fact that altered habitats may also attract invasive species, potentially disrupting established ecological relationships. This aspect underscores the importance of monitoring ecological impacts of compressed tissues on species diversity.
Role in Carbon Sequestration
The role of compressed tissue in carbon sequestration is particularly noteworthy. Compressed tissues, especially in plant forms like peatlands, play a significant part in absorbing and storing atmospheric carbon dioxide. By doing so, they help mitigate climate change effects.
Through carbon sequestration, compressed tissues contribute to soil fertility and biological productivity. This process makes regions that contain such tissues essential for a sustainable ecological future. They function as long-term carbon sinks, preventing excessive greenhouse gas emissions.
In summary, compressed tissue is not merely a physical construct; it actively shapes ecological dynamics. Its influence on biodiversity, habitat formation, and carbon sequestration highlights the multifaceted role that compressed tissues have in sustaining ecosystems.
Challenges Associated with Compressed Tissue
Compressed tissue presents unique challenges that merit thorough examination within the context of its applications and implications. Understanding these challenges can guide future research and practical implementations. It encompasses multiple disciplines, including biology, medicine, and material sciences. These fields benefit from a clear understanding but are also hindered by various obstacles that can restrict advancements and potential applications.
Limitations in Research
The research surrounding compressed tissue is at times limited by several factors. First, the availability of comprehensive data regarding the mechanical and biological properties of compressed tissue can be insufficient. This lack of data often stems from the complexity of the tissue composition and variability in environmental conditions. Moreover, many traditional methods of studying tissue mechanics do not adequately account for the nuances involved in compression, resulting in gaps in understanding.
Furthermore, funding for comprehensive studies may be sparse. Researchers might find it challenging to secure grants for projects that delve into compressed tissue since this area might still be seen as emerging. Limited financial support can constrain the ability to conduct extensive research, leading to an incomplete picture of the potential innovations in this field.
Also, technical challenges arise during experiments. Instruments required to measure properties like elasticity and viscosity are often sophisticated and not widely available. This creates a barrier for smaller institutions or individual researchers who wish to contribute to the field.
Ethical Considerations
Ethical considerations form another critical aspect of challenges in compressed tissue research. Working with biological tissues involves serious ethical scrutiny, particularly when dealing with human samples. Informed consent is essential, yet obtaining it can be complicated in some research contexts.
There’s also the matter of environmental impact. The sourcing of materials for experiments and applications can lead to ecological ramifications. For instance, if research relies heavily on specific biological resources, it may threaten the species involved. Scientists must balance the pursuit of knowledge with the responsibility of maintaining biodiversity and ecological health.
Another ethical issue lies in the potential misuse of technology developed from compressed tissue research. Innovations in this area could be co-opted for applications that may benefit capital over community wellbeing. Thus, researchers must navigate their corporate partnerships carefully and ensure their work aligns with ethical standards that prioritize societal benefit.
Future Directions in Compressed Tissue Research
Future directions in compressed tissue research hold tremendous potential for various scientific fields. Ongoing advancements in this area can significantly impact biological studies and medical practices. The exploration of compressed tissue can lead to novel discoveries and applications that enhance our understanding of tissue mechanics and functions. This section will outline key technological innovations and interdisciplinary approaches shaping the future of this research.
Technological Innovations
Technological innovations are crucial in advancing the field of compressed tissue research. New technologies allow researchers to manipulate and study compressed tissue structures with greater precision than ever before.
- 3D Bioprinting: This technique offers the ability to create complex tissue structures that closely mimic natural tissues. With this innovation, scientists can better understand how different tissue types respond to compression.
- Advanced Imaging Techniques: Techniques like MRI and CT scans provide detailed insights into the internal structure of compressed tissue. These images help researchers observe mechanical properties in real time, leading to better insights into tissue behavior under different stress conditions.
- Nanotechnology: Utilizing nanomaterials can enhance the mechanical properties of compressed tissues. These materials can be incorporated into scaffolds or other constructs to improve their performance in applications such as tissue engineering.
By integrating these technologies, researchers can refine their methods and expand the scope of compressed tissue applications in both medical and ecological fields.
Interdisciplinary Approaches
To fully harness the knowledge surrounding compressed tissue, interdisciplinary approaches are essential. Collaboration among various scientific disciplines fosters innovation and leads to comprehensive insights that would be difficult to achieve within a single field.
- Biology and Engineering: Combining biological understanding with engineering principles allows for the design of better scaffolds for tissue regeneration. Understanding mechanical properties can lead to improved outcomes in tissue engineering.
- Material Science and Medicine: Innovations in material science, including the study of polymers and composites, can enhance the functionality of compressed tissue in medical applications. This can lead to more effective surgical materials and post-operative care solutions.
- Ecology and Health Sciences: Studying compressed tissue's role in ecological systems can also provide insights into its physiological benefits. Understanding interactions in habitats can inspire new medical applications based on ecological principles.
These interdisciplinary efforts ensure that the future research in compressed tissue not only addresses current challenges but also explores new pathways for development and application.
"Interdisciplinary research is essential for unlocking the potentials of compressed tissue, as it bridges the gaps between various fields of knowledge."
"Interdisciplinary research is essential for unlocking the potentials of compressed tissue, as it bridges the gaps between various fields of knowledge."
By focusing on these two critical aspects—technological innovations and interdisciplinary approaches—researchers can direct their energies toward solutions that can transform our approach to compressed tissue in diverse applications.
Epilogue
The examination of compressed tissue is crucial within the framework of this article as it embodies a convergence of various scientific fields. Its relevance extends from practical applications in medicine to theoretical underpinnings in material science and ecology. Through a thorough understanding of compressed tissue mechanics, researchers and professionals can make informed decisions regarding its use and implications.
Moreover, understanding the mechanics of compressed tissue lays the groundwork for exploring its benefits, such as improved surgical outcomes and enhanced material properties. The challenges associated with compressed tissue, including research limitations and ethical considerations, are also crucial. Addressing these challenges will foster innovations in the field.
Summary of Key Points
- Compressed tissue plays a significant role across different disciplines, notably in biology and medicine.
- Its unique properties, such as elasticity and strength, are essential for understanding its functionality and applications.
- Various mechanisms of compression showcase the interactions between physical forces and chemical processes that influence tissue behavior.
- Applications in medical and material sciences reveal its versatility and importance in a range of practices, from tissue engineering to manufacturing.
- Addressing challenges faced in this domain ensures the continued progression of research and technology related to compressed tissue.
Final Thoughts
In closing, the significance of compressed tissue cannot be overstated. It represents a critical area of study that intersects various scientific domains, providing both challenges and opportunities for innovation. Future research must embrace interdisciplinary approaches, leveraging advancements in technology to overcome existing limitations.
As researchers, educators, and professionals continue to explore this area, the knowledge gained will enhance our understanding and application of compressed tissue, paving the way for further advancements in science and technology.
"Understanding compressed tissue is not only about the material itself; it is about its potential to transform various scientific fields, offering solutions to complex problems.“
"Understanding compressed tissue is not only about the material itself; it is about its potential to transform various scientific fields, offering solutions to complex problems.“
In essence, comprehending the nuances of compressed tissue yields vital insights that transcend traditional boundaries, contributing to a more integrated perspective on biological and material sciences.