Energy Storage Innovations: Trends and Future Directions
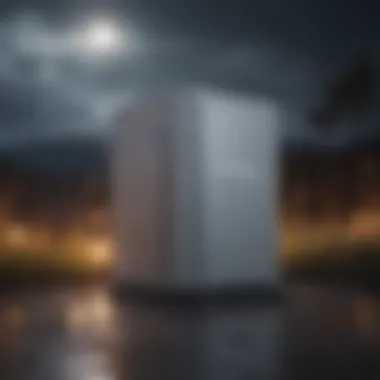
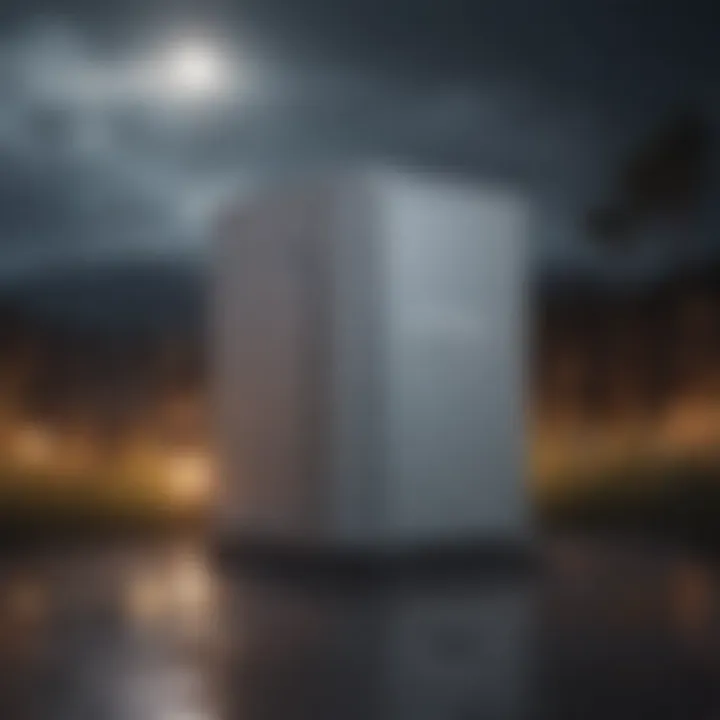
Intro
The landscape of energy storage has seen significant transformations in recent years. With the urgency to adopt sustainable practices, the quest for innovative storage solutions becomes more critical than ever. While renewable energy sources such as solar and wind are pivotal in combating climate change, their intermittency poses a challenge. Energy storage technologies emerge as the bridge to a more resilient energy future, allowing us to harness energy when it’s abundant and dispatch it when needed. As we plunge into this exploration of energy storage innovations, we'll unravel the threads connecting breakthroughs in technology to our essential need for a stable and sustainable energy supply.
Article Overview
Purpose of the Article
This article delves into the various advancements in energy storage technologies, aiming to inform readers about the pivotal developments that drive the efficiency and reliability of energy systems today. With a focus on innovations within different storage methods, we highlight those that show promise for enhancing environmental sustainability. Furthermore, our goal is to provide clear insights into how these technologies impact not only the energy sector but also society at large.
Relevance to Multiple Disciplines
Energy storage touches upon various fields, from science and engineering to economics and environmental studies. Its significance can be observed in diverse areas:
- Engineering: Development of efficient energy storage systems is essential for creating durable and cost-effective solutions.
- Economics: The adoption of these technologies can reshape market dynamics, influencing energy prices and investment strategies.
- Environmental Science: Innovative storage solutions play a role in reducing carbon footprints and promoting sustainability practices.
Research Background
Historical Context
Understanding the evolution of energy storage is key to appreciating its current status. Historically, methods like pumped hydro storage have been relied upon, a practice tracing back to the early 20th century. However, as the world pivots to renewable energy, the limitations of traditional storage become evident. Increased demand for fast-response systems has catalyzed the development of advanced battery technologies and thermal storage methods, which have diversified the energy storage space substantially.
Key Concepts and Definitions
To make sense of the discussions that will unfold, it's crucial to grasp some foundational concepts:
- Energy Storage Systems (ESS): Technologies designed to capture energy output from generation sites and store it for later use.
- Batteries: Electrochemical devices that store energy chemically and release it as electricity.
- Thermal Storage: Systems that store energy in the form of heat for later use, typically through materials that can absorb and retain heat.
- Sustainability: Practices and technologies that aim to preserve environmental quality and reduce resource depletion.
In sum, as we progress through the examination of energy storage innovations, the interplay of technology and sustainability will remain at the forefront. Expect to encounter a variety of storage solutions that hold potential to reshape our approach to energy utilization and resilience.
The Importance of Energy Storage
Energy storage is pivotal in our transition to more sustainable and resilient energy systems. It allows for the efficient management of energy produced from various sources, especially renewable ones like solar and wind. These sources often generate power inconsistently, which creates a mismatch between energy supply and demand. Energy storage systems, therefore, serve as a buffer. They store excess energy generated during peak production times and release it when demand is high, ensuring a steady and reliable energy supply.
Defining Energy Storage
At its core, energy storage refers to the saving of energy for future use. This can take myriad forms, from mechanical systems like pumped hydro storage to chemical systems such as batteries. Each type of storage technology offers unique advantages, catering to distinct applications and requirements.
In simple terms, when energy isn't needed, it gets stored, and when the balance tips, it can be drawn upon. Take, for example, how many homeowners now utilize batteries that charge during the day when solar panels are most productive. When the sun sets, they can use this stored energy, reducing their reliance on the grid.
This ability to shift consumption away from peak periods can lead to both savings and a reduced carbon footprint.
Role in Renewable Energy Integration
The integration of renewable energy sources into the main energy supply grid is fraught with challenges—primarily due to their intermittent nature. Energy storage plays a crucial role in overcoming these challenges. By acting as a balancing agent, it helps smooth out the variability associated with renewables, enhancing grid stability.
For instance, when strong winds cause wind turbines to produce more energy than needed, storage systems can save this excess energy. Later, when demand peaks, that stored energy is readily available. This flexibility not only helps in optimizing the use of renewable resources but also reduces the need for fossil fuel-based peaker plants, which are often employed to meet sudden spikes in demand.
Economic Implications
Investing in energy storage technologies not only enhances energy resilience but also brings substantial economic benefits. As energy prices fluctuate due to various factors including fuel costs and demand uncertainty, energy storage systems can provide price arbitrage opportunities. Users can buy energy when it’s cheap and store it, selling it back or using it when prices are higher.
Moreover, as more industries and residential areas adopt energy storage, economies of scale kick in. The costs associated with manufacturing and deploying these systems can decrease, making them more accessible for wider adoption.
In summary, energy storage is not just a technical solution; it's an economic strategy that boosts efficiency, promotes sustainability, and also supports the development of renewable energy industries.
In essence, energy storage underpins not only the functionality of our future energy systems but also their sustainability and economic viability.
In essence, energy storage underpins not only the functionality of our future energy systems but also their sustainability and economic viability.
Current Innovations in Battery Technology
Current innovations in battery technology are pivotal, especially in the context of energy storage. They represent a significant leap towards achieving efficiency and sustainability in energy usage. With the global push for greener alternatives, the advancements in this field not only promise to improve energy density and performance but also aim to lower costs, thereby making renewable energy resources more accessible and practical. From lithium-ion to newer chemistries, these innovations are reshaping the way we approach energy.
Lithium-Ion Batteries
Advancements in Energy Density
The advances in energy density for lithium-ion batteries are truly remarkable. This term refers to the amount of energy a battery can store in a given volume or weight. As performance parameters improve, manufacturers are able to pack more energy into smaller and lighter packages. One key characteristic of these advancements is the development of high-capacity cathode materials, which enhances storage without increasing size. This makes lithium-ion batteries a preferred choice for portable devices and electric vehicles. With enhanced energy density, users experience longer runtimes, reduced weight, and better overall efficiency. However, the increased energy density also raises concerns about thermal stability, necessitating ongoing research into battery safety and management systems.
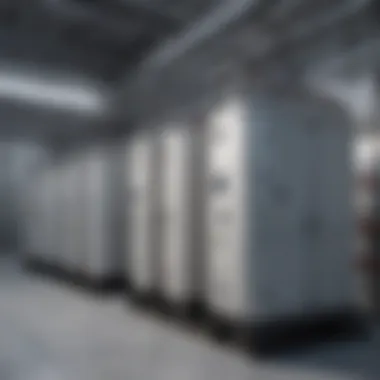
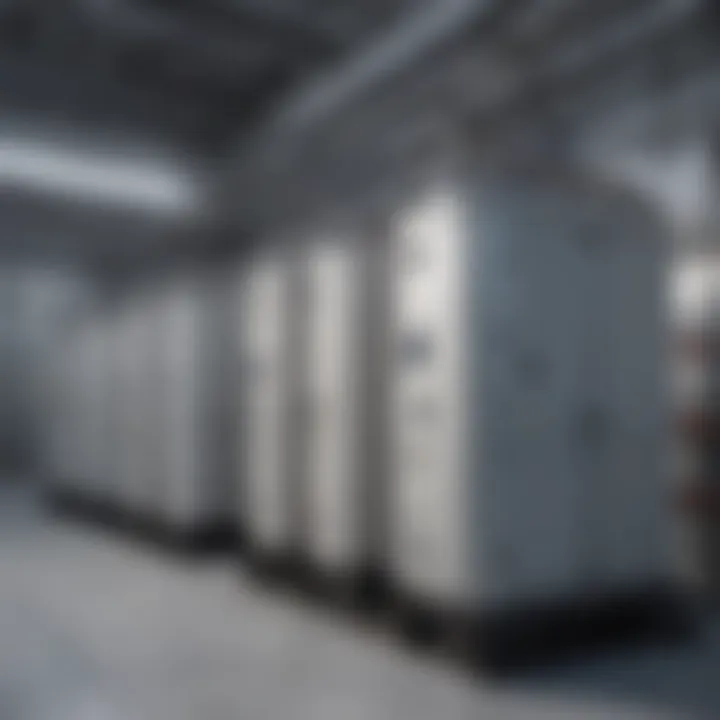
Cost Reduction Strategies
Cost reduction strategies are a crucial aspect of making lithium-ion batteries more accessible. Factors such as economies of scale and improvements in manufacturing processes are lowering the costs. A notable characteristic of these strategies is the use of alternative materials and recycling processes, ensuring that production becomes more sustainable. As the cost of lithium-ion batteries declines, they become more attractive for widespread adoption in electric vehicles and renewable energy storage systems. However, some unique challenges persist, including fluctuations in raw material prices, which can offset gains made in production efficiency.
Solid-State Batteries
Safety Enhancements
Safety enhancements are often cited as the hallmark of solid-state batteries. Unlike traditional lithium-ion cells, solid-state batteries utilize solid electrolytes, which eliminate the risk of leaks and flammability. A key feature is the intrinsic stability of solid-state materials, which makes them less prone to thermal runaway. This enhanced safety profile promotes consumer confidence, which is essential as many people are reluctant to adopt new technologies without assurance of safety. While these batteries do offer significant advantages, their production processes can be more complex and costly compared to conventional battery types.
Performance Improvements
Performance improvements in solid-state batteries can’t be overlooked. They typically exhibit higher energy density and faster charging times. With solid electrolytes, the ionic conductivity can significantly increase, thus enabling quicker energy transfer during charging and discharging. This performance characteristic positions solid-state batteries as a promising option for applications demanding high efficiency, like electric vehicles and grid storage. The downside here lies in the current limitations of scalability and manufacturing techniques, which pose hurdles to their widespread implementation.
Beyond Lithium: Alternative Battery Chemistries
Sodium-Ion Batteries
Sodium-ion batteries present a compelling alternative to their lithium counterparts. A primary advantage is the abundance and low cost of sodium compared to lithium, making it a more sustainable choice. Sodium-ion batteries typically demonstrate a solid performance in terms of cycle stability and environmental impact. However, these batteries still face challenges in terms of energy density, which currently lags behind lithium-ion technology. As research progresses, there’s hope that sodium-based cells will reach competitive performance benchmarks.
Flow Batteries
Flow batteries are another innovative option in the battery landscape. A key characteristic of flow batteries is their unique design, which allows them to store energy in liquid electrolytes contained in external tanks. This provides them with an advantage in scalability and extended discharge times. They are particularly beneficial for grid storage due to their ability to deliver large amounts of energy over long durations. Although their initial costs can be higher and their energy density lower than traditional batteries, their longevity and effectiveness for stationary applications make them a significant part of future energy storage solutions.
Current developments in battery technology—both lithium-based and alternative options—are vital for the future of energy storage. They underscore a transition towards more sustainable practices while meeting the growing demand for efficient energy solutions.
Current developments in battery technology—both lithium-based and alternative options—are vital for the future of energy storage. They underscore a transition towards more sustainable practices while meeting the growing demand for efficient energy solutions.
Thermal Energy Storage Solutions
Thermal energy storage (TES) offers an intriguing approach to storing and utilizing energy, especially within the context of integrating renewable sources like solar or wind power. The core advantage of TES lies in its ability to store excess heat for later use, tapping into a resource that is abundant yet often overlooked. In today's energy landscape, where demand fluctuates and supply intermittently ebbs and flows, the role of thermal storage cannot be understated. Such systems can act as a buffer, supplying energy when and where it's needed most, thus significantly improving grid stability.
One significant consideration regarding thermal storage solutions is their adaptability. Regardless of the source—be it concentrated solar power or waste heat from industrial processes—these solutions can help decouple energy generation and consumption. Moreover, the integration of TES can promote a streamlined approach to energy management, enabling facilities and communities to respond dynamically to real-time energy demands.
Phase Change Materials
Phase Change Materials (PCMs) have emerged as a promising avenue for thermal energy storage. These materials have the unique ability to absorb or release latent heat as they transition between solid and liquid states. With their high energy storage capacity and relatively simple integration into existing infrastructure, PCMs are gaining traction in both residential and commercial applications.
- They can significantly improve the efficiency of heating and cooling systems.
- Reducing peak energy usage not only helps balance the electric grid but also lowers utility costs for end-users.
For instance, using PCMs in building materials like higher-performance wallboards allows structures to maintain more stable indoor temperatures. During warmer periods, the PCM absorbs heat, effectively storing it. Later, when temperatures drop, it releases this stored heat, minimizing the need for active heating systems.
Additionally, the deployment of PCMs in industrial settings can contribute to thermal load shifting, enabling companies to make better use of energy in relation to price fluctuations throughout the day. This presents both an economic and environmental win that aligns with broader objectives for sustainable energy usage and greenhouse gas reduction.
Molten Salt Technology
Molten salt technology is another fascinating facet of thermal energy storage that has gained considerable interest due to its robustness and efficiency. Typically utilized in concentrated solar power (CSP) plants, molten salt can retain heat at significantly high temperatures, which can then be employed to generate steam and produce electricity on-demand.
- An advantage of molten salt storage is its scalability, allowing it to be tailored for small installations or extensive solar farms.
- Operating under high temperatures also boosts thermal efficiency compared to other conventional storage methods, leading to increased overall system performance.
Moreover, molten salt systems provide flexibility that enhances grid stability. They can store energy generated at midday—when solar output is at its peak—and discharge it during evenings, effectively flattening daily energy consumption peaks.
"The transition towards thermal energy storage technologies unveils a pathway towards more resilient and adaptive energy systems, capable of addressing the challenges faced by renewable integration."
"The transition towards thermal energy storage technologies unveils a pathway towards more resilient and adaptive energy systems, capable of addressing the challenges faced by renewable integration."
In summary, with thermal energy storage solutions like Phase Change Materials and molten salt technology, the possibilities appear plentiful. These systems not only bolster sustainability efforts by enabling better use of renewables, but they also contribute to the boilerplate strategies toward future energy demands.
Innovative Concepts in Energy Storage
The landscape of energy storage is evolving rapidly, driven by the demands of a world seeking sustainable and efficient energy solutions. Innovative concepts play a crucial role in redefining how we store and use energy. These approaches not only enhance existing technologies but also pave the way for new methods that could change the energy storage game. By delving into these innovative concepts, we can uncover their potential benefits and the considerations they bring along.
Hydrogen Storage Technologies
Hydrogen storage technologies represent a significant step forward in energy storage capabilities. This segment includes two primary components: electrolyzers and fuel cells. Each of these serves a distinct purpose yet collectively contributes to a more resilient energy framework.
Electrolyzers and Fuel Cells
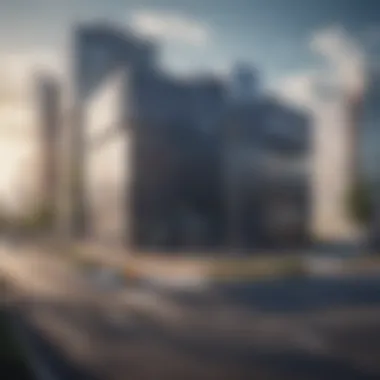
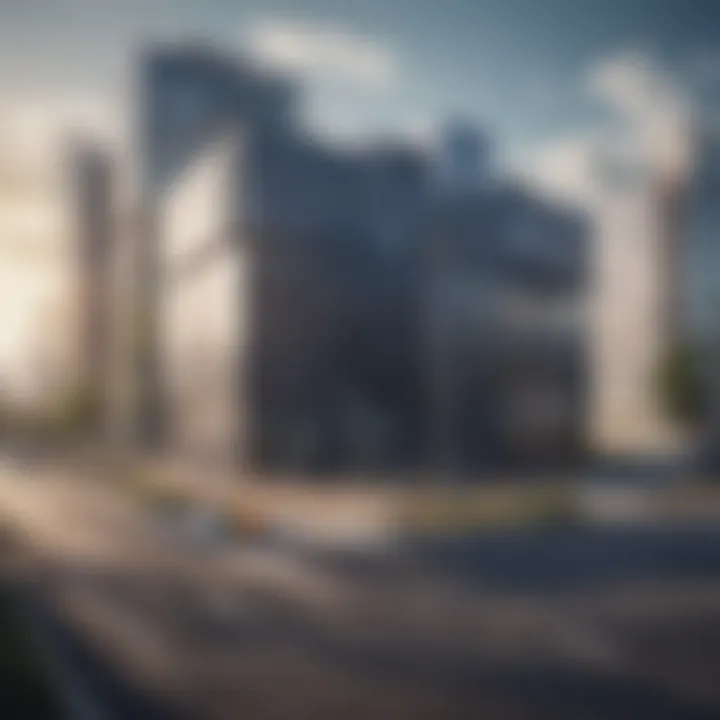
Electrolyzers are systems that employ electricity to split water into hydrogen and oxygen. This process, known as electrolysis, is pivotal for generating hydrogen in a clean manner. The key characteristic of electrolyzers is their ability to produce hydrogen using renewable energy sources, making them a popular choice in today's quest for greener solutions.
The unique feature of electrolyzers lies in their versatility. They can operate on intermittent energy sources, such as solar or wind, effectively capturing surplus energy and converting it into hydrogen. However, a downside to consider is the efficiency loss during this conversion, which can be a hindrance in some applications.
Fuel cells, on the other hand, use stored hydrogen to generate electricity through a chemical reaction. This provides an efficient way to utilize the hydrogen produced. The advantage here is that fuel cells emit only water vapor, adding to their appeal as a clean energy solution. But, the reliance on hydrogen production and the infrastructure required to support it can create challenges.
Applications in Transportation
When looking at applications in transportation, it's clear that hydrogen storage brings a transformative effect. The use of hydrogen fuel cells in vehicles not only allows for rapid refueling but also extends driving range compared to traditional electric batteries. This characteristic makes hydrogen a viable and attractive alternative in sectors where logistics and operational efficiency are paramount.
The unique feature of hydrogen applications in transportation is their ability to support heavy-duty vehicles, including buses and trucks, which require longer ranges and faster refueling times. This offers tangible advantages over battery electric vehicles in certain contexts. However, the relatively high cost of hydrogen production and the current lack of widespread fueling infrastructure can pose significant challenges to broader adoption.
Gravity-Based Energy Storage
Gravity-based energy storage systems leverage potential energy by utilizing heavy materials and elevated positions. When energy needs to be stored, these systems elevate massive weights, using electrical energy to do so. When energy is required, the weights are lowered, and their gravitational potential energy is converted back to electricity. This gives gravity storage the advantage of a simple and robust design with minimal environmental impact. It's particularly appealing for locations where space for traditional batteries might be limited.
Flywheel Energy Storage Systems
Flywheel energy storage systems utilize rotational kinetic energy to store and release energy. These systems are particularly adept at managing short-term energy fluctuations. A key characteristic of flywheels is their ability to charge and discharge rapidly, which makes them beneficial for applications requiring immediate power. For instance, they can quickly respond to sudden changes in energy demand or supply, stabilizing the grid effectively.
The unique aspect of flywheel systems is their durability and longevity compared to traditional batteries. They tend to last longer, with fewer performance degradation issues over time. However, the initial costs and the need for a vacuum-sealed environment to minimize friction pose some challenges.
"Innovative concepts in energy storage are not merely adaptations of existing technologies; they are game changers that hold the potential to redefine our energy landscape."
"Innovative concepts in energy storage are not merely adaptations of existing technologies; they are game changers that hold the potential to redefine our energy landscape."
With these innovative concepts on the table, the future of energy storage appears more promising than ever. As we move forward, the ongoing advancements in these technologies could very well lead us to a sustainable and efficient energy paradigm.
Integration of Artificial Intelligence in Energy Storage
The integration of artificial intelligence in energy storage represents a frontier that could potentially revolutionize how we manage and utilize energy more efficiently. The importance of this topic within the context of energy storage innovations cannot be overstated. As our reliance on renewable sources grows, the demand for intelligent systems that can optimize energy usage, predict storage needs and minimize waste becomes increasingly critical. Existing storage technologies need a layer of sophistication that only AI can provide, enhancing their performance and sustainability.
AI offers a multitude of benefits over traditional methods of energy management. For one, it can analyze vast amounts of data in real-time. This capability allows stakeholders to make informed decisions about energy consumption and storage based on demand forecasts and weather patterns. Moreover, AI systems can adaptively learn, improving their efficiency over time, which is something static models simply can't achieve. With smart grid applications on the rise, ensuring seamless integration of energy storage devices plays a vital role in maintaining energy reliability and meeting consumer needs.
However, while the advantages are clear, deploying AI in energy storage isn't without its challenges. Ensuring data privacy, addressing algorithmic bias, and integrating these systems with existing infrastructure require careful planning and execution. Furthermore, the initial investment in AI technologies can be significant, potentially leading to hesitancy from stakeholders who must weigh immediate costs against long-term gains.
"For the advancement of smart energy storage technologies, companies must invest not only in hardware but also in the intelligence that drives these systems."
"For the advancement of smart energy storage technologies, companies must invest not only in hardware but also in the intelligence that drives these systems."
Predictive Analytics and Management
Predictive analytics acts as the backbone to leveraging artificial intelligence in energy storage. By employing statistical algorithms and machine learning techniques, predictive models can forecast consumption patterns and energy requirements. These insights help in determining when to store energy and when to release it, making systems responsive to fluctuating demands.
Several factors contribute to effective predictive analytics:
- Historical Data Utilization: By analyzing past consumption data, predictive models can recognize trends, seasonality, and anomalies in energy usage.
- Weather Integration: Weather patterns significantly influence energy generation, especially with renewables like solar or wind. Accurate predictions consider meteorological data to enhance grid reliability.
- Consumer Behavior: Understanding customer energy consumption habits allows for tailored energy solutions that align with user needs.
This predictive power not only allows for enhanced efficiency but also enables cost savings since energy is utilized optimally.
Optimization of Storage Systems
Beyond just prediction, AI plays a crucial role in optimizing storage systems themselves. This optimization can take various forms, focusing on maximizing energy retention while minimizing costs and environmental impact. For instance, intelligent algorithms can determine the best charging and discharging cycles based on various inputs — like price signals and availability of renewable energy sources.
Key aspects of optimization include:
- Load Balancing: AI can help balance loads across different storage devices, preventing overloads and ensuring systems operate at peak efficiency.
- Resource Allocation: By optimizing how resources are allocated among various storage technologies, AI maximizes their effectiveness while reducing wear and tear.
- Long-term Projections: Optimization models can simulate long-term energy storage scenarios, providing foresight into capacity needs and helping to plan for future energy infrastructure.
Investing in artificial intelligence solutions for energy storage is a forward-looking approach that not only addresses present challenges but also embraces the possibilities of future innovations. By unlocking the potential of AI in this sector, we can ensure that energy storage evolves alongside the ever-changing landscape of energy production and consumption.
Energy Storage Policy and Regulation
The landscape of energy storage is complex and dynamic, influenced by a myriad of factors, including technological advancements, market dynamics, and crucially, the policy and regulatory environment. Energy storage policy and regulation play a fundamental role in shaping the future of energy storage innovations. They provide the backbone for investment and development, ensuring that innovations not only flourish but also align with broader sustainability goals. Without supportive policies, many cutting-edge technologies might struggle to find a foothold in the market, prolonging our reliance on less efficient means of energy storage.
Effective policies influence several aspects:
- Encouragement of new technologies: With the right incentives, companies are more likely to invest in research and development of novel storage solutions.
- Market creation: Policies that support energy storage ensure a robust market where these solutions can thrive. Importantly, incentives lead to increased adoption and reduced costs.
- Regulatory clarity: In an industry that is constantly evolving, clear regulations help companies navigate compliance and focus on innovation rather than bureaucracy.
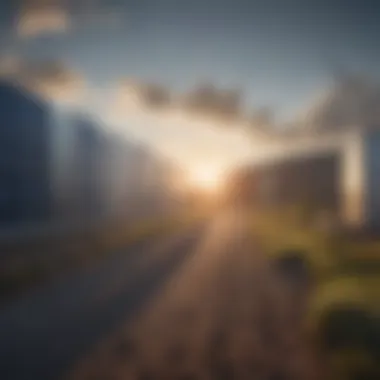
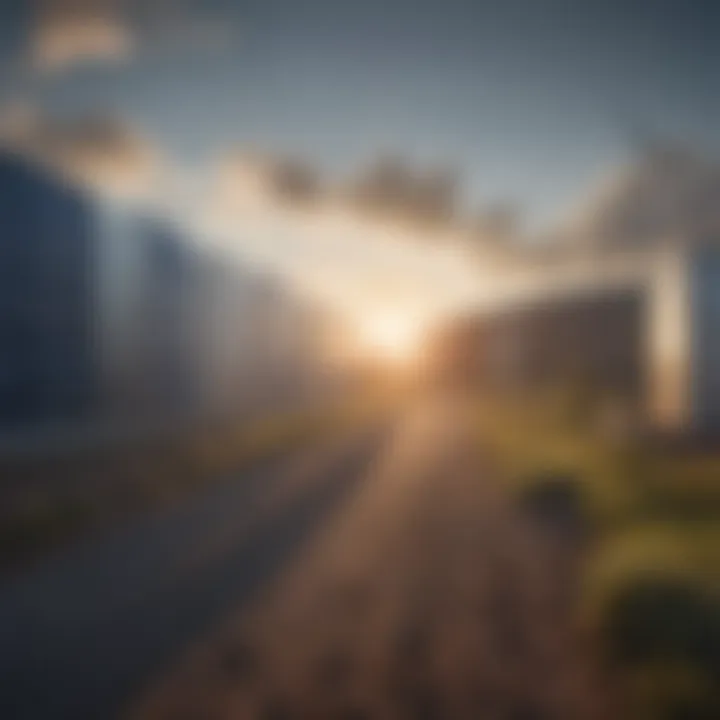
Ultimately, the aim is to foster a balanced environment where energy storage can contribute to a sustainable energy future.
Incentives for Innovation
Incentives for innovation within energy storage are more than just financial; they encompass a broad range of supports that can encourage research, encourage development, and facilitate deployment of cutting-edge technologies. One of the most effective means is through subsidies and grants offered by governments. These financial supports help startups and established companies mitigate risks associated with developing new technologies. Additionally, tax credits for research expenditures can essentially lower the entry barriers for innovators willing to explore cutting-edge energy storage methods.
Another layer of support comes from feed-in tariffs or similar mechanisms that guarantee a premium price for energy generated and stored from renewable sources. This not only provides revenue assurance for energy storage projects but also incentivizes the development of energy storage systems tailored toward renewables.
Other potential incentives might include:
- Public-private partnerships to foster collaboration between government and industry.
- Research tax credits that encourage more investment in basic and applied research efforts in the energy storage sector.
Such incentives create an environment conducive to innovation, promoting competition that can yield better performance and more reliable energy storage solutions.
Regulatory Frameworks
Regulatory frameworks surrounding energy storage must be thoughtfully structured to encourage the growth of innovative technologies while ensuring safety and reliability. With the rapid developments in energy storage technologies, existing regulatory frameworks often lag behind, potentially stifling innovation.
Some key components of effective regulatory frameworks include:
- Interconnection standards: Clear and straightforward standards are necessary for energy storage systems to connect with the grid without undue delays or complexities.
- Safety regulations: Ensuring the safety of new technologies is paramount, and this necessitates the establishment of guidelines that all energy storage solutions must comply with.
- Market participation rules: Energy storage should be allowed to compete on an equal footing with other technologies in energy markets, having the ability to provide services like frequency regulation or demand response.
Certain regions have already started to embrace innovative frameworks, where energy storage is treated similarly to generation assets. This evolving treatment helps integrate energy storage more readily into the energy market dynamics.
Challenges Facing Energy Storage Innovations
In the pursuit of advanced energy storage solutions, various challenges loom large, significantly influencing the direction and success of innovations in this sector. Recognizing these hurdles is crucial for researchers, manufacturers, and policymakers alike. Addressing these challenges could pave the way for more sustainable and efficient energy systems. Let’s delve into two major issues: resource scarcity and waste management concerns.
Resource Scarcity
One pressing issue facing energy storage innovations is resource scarcity. Many of the materials required for building batteries—such as lithium, cobalt, and nickel—are not just expensive, but their supply can be unpredictable. Mining these materials often leads to environmental degradation and geopolitical tensions, as resources are not evenly distributed across the globe. For instance, the Democratic Republic of the Congo accounts for a significant portion of the world's cobalt supply. This concentration gives rise to vulnerability in the supply chain, making the electric vehicle and energy storage industries susceptible to fluctuations in availability and price.
Moreover, as demand for these technologies grows, the competition for resources intensifies. If we keep tapping into these materials at current rates, we may find ourselves in a tight spot in a couple of decades. Researchers are actively seeking alternative materials or innovations that can help mitigate this challenge. Some are exploring the potential of abundant elements such as sodium, which can be a game changer if it proves to be a viable alternative to lithium-ion batteries. Sodium-ion batteries could establish a more stable supply chain, reducing reliance on scarce materials and leading to a more sustainable cycle of energy storage.
Waste Management Concerns
The disposal and recycling of energy storage systems, particularly batteries, raise significant environmental questions. As outdated battery technology is phased out, the proliferation of electronic waste is a considerable concern. Batteries contain hazardous materials that, if not properly managed, can lead to soil and water contamination. In addition, with the rapid rise of consumer electronics and electric vehicles, the number of batteries reaching the end of their life will continue to increase dramatically.
Effective strategies for recycling are still in their infancy. While advancements have been made in reclaiming valuable materials from spent batteries, the current processes can be inefficient and expensive. Companies like Redwood Materials and Li-Cycle are pioneering methods to recover lithium and other materials in a way that minimizes environmental impact. However, widespread adoption and more efficient recycling technologies are essential to reduce the amount of lithium-ion battery waste that ends up in landfills.
"Sustainable management of waste from energy storage systems is not just about preventing pollution; it’s about creating circular economies that harness the resources we have already extracted."
"Sustainable management of waste from energy storage systems is not just about preventing pollution; it’s about creating circular economies that harness the resources we have already extracted."
The challenge of waste management is multifaceted. It necessitates a collaborative effort among manufacturers, consumers, and policymakers to create regulations, incentives, and education around recycling practices and the importance of responsibly managing battery life cycles. Drawing from materials science innovations and consumer engagement will be key in transforming these challenges into opportunities for progress.
Overall, understanding these fundamental challenges informs future directions in energy storage technology and encourages the development of sustainable practices that can lead to both economic and environmental benefits.
Future Directions in Energy Storage
The field of energy storage is poised for significant transformations that hold the potential to reshape the entire energy landscape. As reliance on renewables increases, the need for robust and versatile storage solutions becomes paramount. The exploration of future directions in energy storage highlights several key innovations and methodologies that can enhance efficiency, sustainability, and cost-effectiveness.
Emerging technologies not only facilitate better energy management but also offer fresh approaches to integrate renewable resources with the grid. Deep diving into novel concepts like nanotechnology and biological methods illustrates the breadth of innovation. Each of these areas contributes to advancing energy storage applications, providing practical solutions to some of the pressing challenges faced in energy systems today.
Thus, examining the future directions not only provides insight into technological advancements but also underscores the broader implications these innovations have on energy resilience and sustainability. With that in mind, let’s explore the realms of emerging technologies and their contributions to the future of energy storage.
Emerging Technologies
Nanotechnology Applications
Nanotechnology is making waves in energy storage systems, particularly in battery technology. One of the standout characteristics of nanotechnology is its ability to enhance the performance of materials at a molecular level. For instance, nanoscale materials, such as carbon nanotubes or graphene, improve conductivity and increase the surface area of electrodes in batteries. This leads to higher energy densities and faster charge rates—an irresistible characteristic for both consumer electronics and electric vehicles alike.
The primary advantage of employing nanotechnology in energy storage lies in its capacity for miniaturization without sacrificing performance. Compared to traditional materials, these nanostructures often result in lighter and more efficient batteries. However, it’s essential to consider the trade-offs involved. For example, the complexity and cost of producing nanomaterials may offset some of the efficiency gains, raising questions about scalability in commercial applications.
Biological Methods
Shifting gears, biological methods present another captivating avenue towards innovation in energy storage. Microorganisms, such as algae and bacteria, can be harnessed to store energy in more sustainable and environmentally friendly ways. One notable aspect is the ability of certain bacteria to produce biofuels through natural processes, converting organic materials into usable energy seamlessly.
The inherent advantage of biological methods is their ability to leverage existing biological processes, making it an appealing option for increased sustainability. Furthermore, in terms of compatibility with the environment, these methods tend to have a lower ecological footprint compared to traditional chemical storage techniques. However, challenges remain. These methods are often slower and less energy-dense compared to their chemical counterparts, which may limit their viability on a larger scale.
Role in Grid Modernization
The modernization of the grid is vital for accommodating diverse energy sources and ensuring stable delivery of electricity. Energy storage plays a crucial role in this transition, offering solutions for managing peak demand, stabilizing supply, and integrating intermittent renewable energy. By deploying advanced storage technologies, utilities can enhance grid reliability, reduce energy waste, and lower costs for end-users.