Environmental Impacts of Solar Panel Production and Disposal
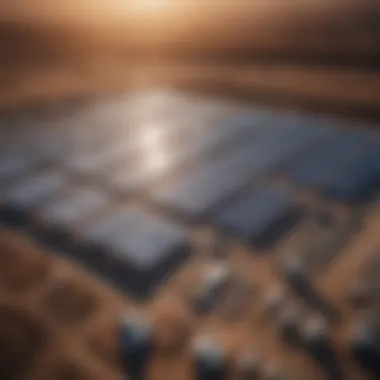
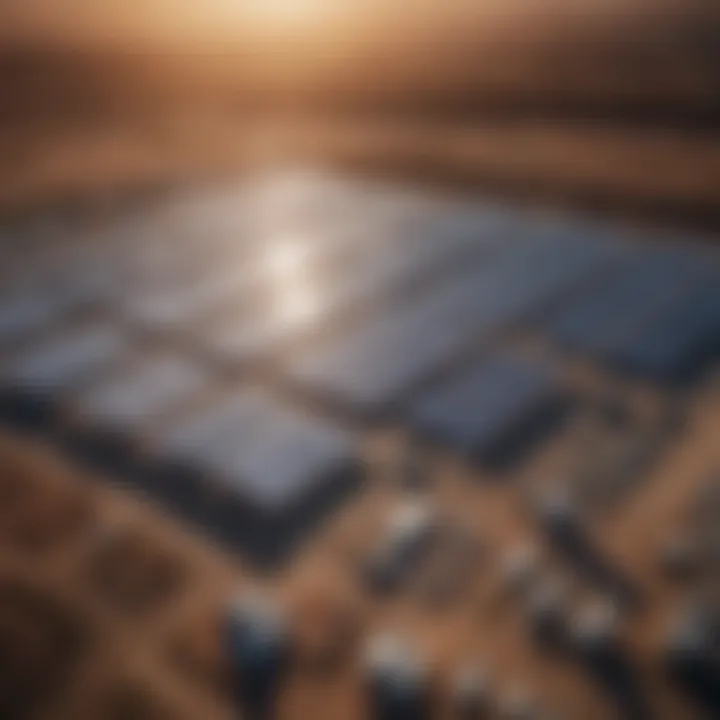
Article Overview
Purpose of the Article
This article aims to shed light on the often-overlooked environmental implications tied to the production and disposal of solar panels. As solar energy gains traction as a prominent alternative to fossil fuels, understanding the ecological footprint of this technology becomes increasingly crucial. By dissecting the intricacies of solar panel manufacturing, the hazardous materials involved, land use changes, and the challenges faced at the end of the panel's life cycle, the intention is to provide a balanced perspective on this renewable energy source. We seek to inform readers, helping them grasp not just the benefits but also the potential pitfalls associated with solar energy.
Relevance to Multiple Disciplines
Solar panels stand at the intersection of technology, ecology, and policy, rendering this discussion relevant to a broad spectrum of fields including environmental science, engineering, economics, and public policy. Students, researchers, educators, and professionals alike can benefit from a nuanced understanding of the environmental consequences tied to solar technology. This article will explore how decisions made in one field can reverberate across others, making the topic particularly rich for interdisciplinary study.
Research Background
Historical Context
The rise of solar technology dates back several decades, gaining momentum as concerns over climate change and energy security escalated. Initially, solar panels were viewed through a lens of innovation and promise, often hailed as a miracle solution to long-standing environmental issues. However, as production processes have ramped up, the detrimental effects of manufacturing practices have come into sharper focus. By tracing the evolution of solar technology, we can identify key milestones that have shaped not only the product itself but also the surrounding environmental landscape.
Key Concepts and Definitions
Understanding the intricate relationship between solar panels and the environment necessitates defining several core concepts. For instance, "life cycle assessment" (LCA) is a crucial framework enabling researchers to evaluate the environmental impacts of solar panels from raw material extraction through production to disposal. Similarly, "hazardous materials" refers to toxic substances, such as cadmium and lead, often used in the photovoltaic cells of solar panels. These definitions serve as foundational pillars as we explore how various stages of solar panel life intersect with environmental health.
"A full understanding of solar technology must include a look not just at energy generation but also at its entire life cycle and its environmental footprint."
"A full understanding of solar technology must include a look not just at energy generation but also at its entire life cycle and its environmental footprint."
In a world increasingly geared towards renewable energy, examining these complexities is essential for informed decision-making about energy solutions.
Preamble
In the quest for sustainable energy, solar panels have emerged as a prominent solution. Their growing popularity has sparked a debate about the hidden environmental costs underlying their production and disposal. This article aims to peel back layers of this topic, revealing the broader ecological footprint of solar technology. By delving into processes such as resource extraction, hazardous materials involved, land use changes, and end-of-life issues, we strive to provide a clear picture of the dilemmas facing renewable energy solutions.
Understanding the environmental consequences associated with solar panels isn't just an academic exercise. As the push for greener technologies accelerates, the implications are becoming increasingly significant—not only for policymakers but also for consumers and industry stakeholders. When we consider the myriad benefits presented by solar energy, it’s crucial to not overlook the complexities involved.
From a pragmatic angle, one might think that solar energy represents a purely positive contribution to mitigating climate change. However, one must consider the full cycle of solar products—from manufacturing to disposal.
"Every technology has a shadow; understanding it is vital for true sustainability."
"Every technology has a shadow; understanding it is vital for true sustainability."
Key elements to cover in this dialogue include:
- Manufacturing Processes: What’s actually involved in creating solar panels, and what resources are consumed?
- Hazardous Materials: Are there toxic components within these panels that pose environmental risks?
- Impact on Land: How does the land conversion for solar farms disrupt existing ecosystems?
- Disposal Challenges: What happens when solar panels reach the end of their lives?
By addressing each of these areas, we hope to arm our readers with a well-rounded perspective on the environmental challenges posed by solar technology. This is not merely about assessing the benefits of renewable energy; it’s about recognizing the inherent trade-offs that come with greener choices.
Manufacturing Processes and Resource Extraction
The manufacturing processes and resource extraction stages are critical as they directly influence the ecological footprint of solar panel production. Understanding the materials used, the energy consumed, and the emissions produced during these steps offers insight into the overall sustainability of solar power technology. Effectively, every choice made in this phase can either mitigate or exacerbate environmental harm, indicating that thorough examination is paramount for anyone analyzing the renewable energy landscape.
Materials Required for Solar Panel Production
Silicon
Silicon is the backbone of most solar panels. It is a semiconductor, which has a great capacity for absorbing sunlight. The key characteristic of silicon that makes it such a popular choice is its abundance. It’s literally everywhere – making up about 28% of the Earth's crust. Using something so fundamentally available does help in driving down costs, enabling more accessible solar technologies.
However, the production process of silicon entails heavy energy use. While silicon is a material that boasts great efficiency when converted into solar energy, the manufacturing procedures can be not-so-green at times. The energy-intensive approach not only undermines some of the environmental benefits that might be associated with solar panels but also raises questions about where the energy comes from. One unique feature that stands out is the detailed purification process that silicon undergoes, which often generates waste products harmful to the environment.
Metals and Minerals
A range of metals and minerals are essential for solar panel functionality. Aluminum and silver, for instance, are widely used. Aluminum constructs the frame of panels, providing durability and strength. Its recyclability is a significant point, as it can have multiple life cycles without losing quality. Silver, despite its skyrocketing price, is needed for its superior conductivity attributes.
Yet, the extraction of these metals carries environmental risks. Mining operations can lead to significant land degradation and biodiversity loss. Areas where these minerals are harvested often see long-lasting impacts on the ecosystem due to habitat destruction. By the same token, the reliance on such materials leads to economic disparities in regions where they are sourced, raising ethical considerations.
Chemical Components
Various chemicals are utilized in the production of solar cells, adding complexity to the environmental narrative. When we talk about chemical components, we usually refer to phosphorous and boron, which are dopants that enhance the efficiency of silicon solar cells. Their capacity to facilitate electron movement is vital for achieving the necessary electrical performance in panels.
However, the use of these chemicals carries implications. The specific processes involving these substances can lead to harmful byproducts if not managed correctly. One notable aspect is that the production facilities must have stringent waste management measures to prevent these chemicals from leaking into soil and waterways. Therefore, while these chemicals play a vital role in augmenting efficiency, hacks in their handling can cause greater environmental detriment.
Carbon Footprint of Manufacturing
Energy Consumption
When calculating the carbon footprint of solar panels, energy consumption forms the core metric. From the extraction of raw materials to the actual manufacturing of the panels, the entire process is energy-hungry. It’s critical to assess whether this energy is derived from renewable sources or fossil fuels. The latter seriously diminishes the green promise that solar panels carry. The trend towards employing renewable energy for manufacturing would drastically reduce the accumulation of greenhouse gases associated with these processes.
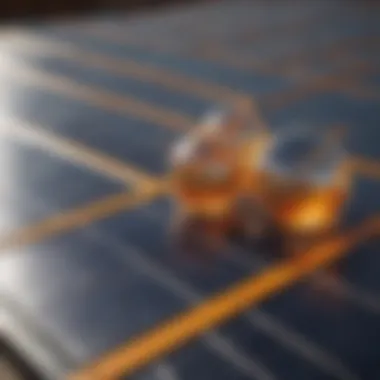
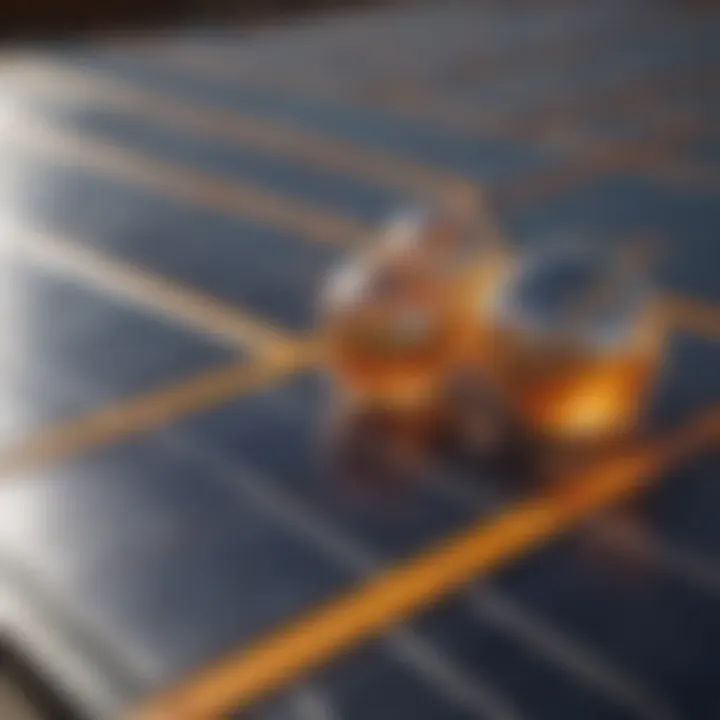
One of the unique features of examining energy consumption is that it also spotlights the efficiency of technology over time. As newer approaches are integrated into manufacturing, potential reductions in energy needs may arise, ultimately influencing the solar power economics and environmental footprint positively.
Greenhouse Gas Emissions
Solar panel production isn’t without its emissions. The carbon dioxide released all through various manufacturing stages prompts a deeper examination. These emissions primarily arise from energy consumption as fossil fuels are frequently employed. When looking specifically at greenhouse gas emissions, one striking realization is that they can be relatively high compared to the expected benefits of solar energy consumption later.
The unique feature here is the break-even point in emissions. Essentially, it takes years of solar energy production for the benefits of reduced emissions to outweigh the initial output during production. This delay calls into question whether the solar future can be as rosy as we might hope unless strategies are put in place to reduce those initial emissions dramatically.
"Understanding the energy inputs and emissions in solar production is crucial. This reflection shapes the narratives around solar as both a renewable solution and its unforeseen drawbacks."
"Understanding the energy inputs and emissions in solar production is crucial. This reflection shapes the narratives around solar as both a renewable solution and its unforeseen drawbacks."
Hazardous Materials in Solar Panels
Understanding the role of hazardous materials in solar panels is a crucial piece of the puzzle when looking at their environmental impact. These materials are integral to the operation of solar technology, but they come with significant concerns. For one, the presence of toxic elements during production and their consequent effects not only on the environment but also on human health cannot be overstated. In the light of increasing renewable energy demands, it becomes important to critically examine these factors.
Toxic Chemicals Used in Production
Cadmium
Cadmium, a heavy metal with a silvery-white appearance, plays a pivotal role in certain types of thin-film solar cells, particularly those made from cadmium telluride. This aspect ties into the overall issue at hand, as cadmium's efficiency in converting sunlight to energy makes it a desirable component. However, its key characteristic lies in its notorious toxicity, prompting serious health risks associated with long-term exposure. The unique feature of cadmium, contributing to the challenges addressed in this article, is its propensity for bioaccumulation in the food chain. When improperly handled, cadmium can seep into soils—posing risks not only to agricultural lands but also to surrounding ecosystems.
Lead
Lead has been used widely in various products, including the production of solar panels, due mainly to its favorable conductive properties. Its popularity stems from its efficiency in energy conversion, making panels more viable for energy generation. Yet, lead's unique property as a neurotoxin raises serious alarm bells. This material can leach into the environment if proper disposal procedures are not followed. Although lead may bolster panel performance, the disadvantages are stark; its potential to contaminate soil and water presents an urgent problem that must be tackled through stringent regulations and practices.
Antimony
Antimony is another heavy metal used in some solar cell applications, contributing to the technology’s overall performance. Its key feature is a unique ability to improve the efficacy of certain photovoltaic materials. Administrations note that while antimony compounds are helpful, they also pose risks particularly if they find their way into the environment unchecked. The challenge here is balancing the advancements in solar technology with the environmental ramifications that antimony can bring. Thus, while antimony might hold promise, the concerns regarding its usage must prompt caution, making it a dual-edged sword in the solar panel production landscape.
Environmental Risks of Chemical Exposure
Soil Contamination
Soil contamination emerges as a serious concern linked to the hazardous materials present in solar panels, especially when these panels reach the end of their lifecycle. This situation often arises during the disposal or recycling phase, where toxic elements can leach into the earth. The significance of soil contamination cannot be overlooked; it leads to degraded land quality and adversely affects agricultural productivity.
The unique aspect of contaminated soil is the long-term harm it can do, not only affecting crops but also disrupting local ecosystems. Replacing contaminated topsoil is often a daunting task, making prevention and management of hazardous materials in solar technology a paramount concern.
Water Pollution
Water pollution acts as another consequential layer of the environmental impacts discussed here. Heavy metals, including cadmium, lead, and others, can leach from solar panels into nearby water bodies during their disposal or mishandling. This key characteristic of water pollution is its ability to spread rapidly, potentially affecting human populations reliant on these water sources. The unique feature of this issue is that contaminants can accumulate in aquatic organisms, entering the food chain and posing risks to fish populations and their predators, including humans. Thus, it is imperative that both the solar industry and regulatory bodies address these challenges.
"The challenge of managing hazardous materials properly can determine the ultimate sustainability of solar technologies."
"The challenge of managing hazardous materials properly can determine the ultimate sustainability of solar technologies."
Land Use and Ecological Impact
The discussion around solar energy often overlooks the nuanced interplay between land use and its ecological effects. This topic is vital to the broader discourse of renewable energy as implementing solar panels requires significant land transformation, which can have far-reaching consequences for the environment. Understanding these impacts helps in assessing the sustainability of solar energy as a green alternative to fossil fuels, prompting a critical examination of how we can balance energy needs with ecological preservation.
Impacts of Land Conversion
Habitat Destruction
One cannot discuss land conversion without bringing attention to habitat destruction. When land is cleared for solar farms, ecosystems that have flourished for ages are often lost completely. This is mainly because natural habitats, such as forests and wetlands, are crucial for providing shelter and food to myriad species.
The key characteristic of habitat destruction is its immediate and profound effect on local wildlife. When their living spaces are carved out for solar installations, it often leads to the displacement of species, creating fragmented habitats that are unsuitable for their survival. This point is particularly salient in regions where biodiversity is rich, as it can tip delicate ecosystems into a state of collapse.
The unique feature of habitat destruction related to solar energy production highlights its role as a guilty bystander in the push for renewable resources. The advantages might be the generation of clean energy, but the disadvantages could be incalculable ecological costs as entire species face extinction.
Biodiversity Loss
The link between solar panel installations and biodiversity loss is profound. As land is repurposed for photovoltaic systems, the delicate balance of ecosystems is often disrupted. Biodiversity loss can be characterized by the decline or extinction of various plant and animal species that once thrived in those environments.
This phenomenon is critical because biodiversity contributes to ecological resilience, enabling ecosystems to withstand and recover from environmental changes. Notably, the key characteristic of biodiversity loss is that it doesn’t just affect individual species—it can lead to a domino effect, disrupting food chains and ecological interactions.
When assessing the implications of solar farms on biodiversity, it becomes clear that while solar energy represents a step towards reducing carbon footprints, it can also lead to unintended consequences. The unique aspect here is the challenge of finding a balance: how can society benefit from renewable energy without simultaneously harming the intricate web of life that sustains it? The advantages of adopting solar technology are undeniable, but they come at the potential cost of losing biodiversity, which is essential for healthy ecosystems.
Solar Farms and Land Efficiency
Agrivoltaics
Agrivoltaics emerge as a promising approach within the landscape of solar energy. This concept involves placing solar panels on agricultural land, allowing the land to be utilized for both farming and energy generation. The benefit of this technique is considerable, as it enhances land efficiency; farmers can produce their crops while generating renewable energy, maximizing the utility of each plot of land.
A noteworthy characteristic of agrivoltaics is its double-edged nature. By harnessing solar power while still permitting agriculture, this method offers an innovative solution to the concerns surrounding land use. It showcases that solar energy does not have to come at the expense of food production. Moreover, this symbiotic relationship allows for better land management, mitigating some of the ecological impacts associated with conventional solar farming.
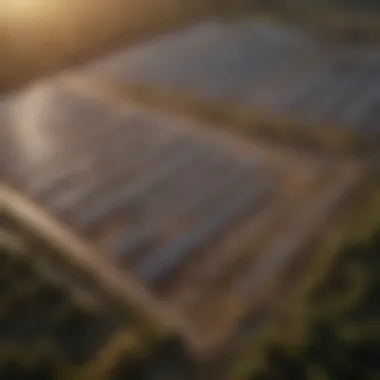
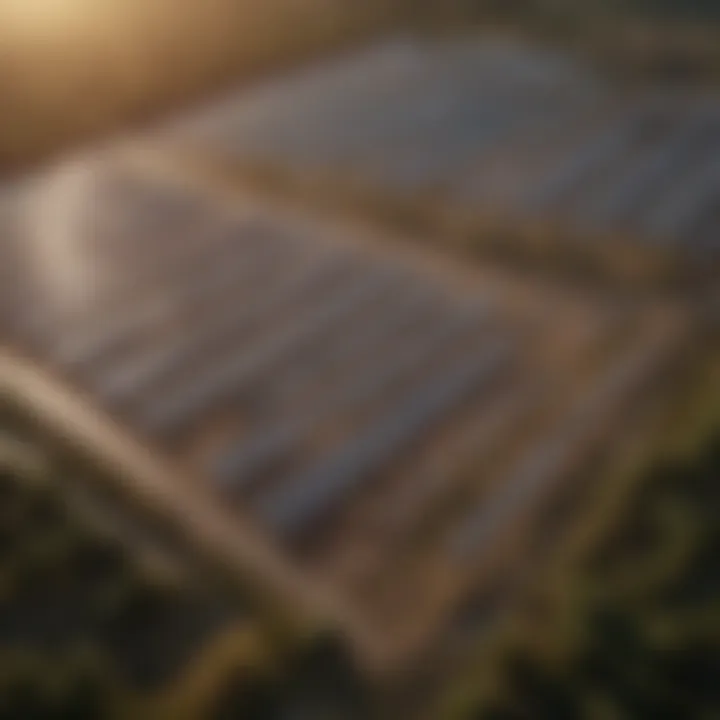
However, there are disadvantages to consider as well. Transitioning to agrivoltaics may require upfront investment and adaptation in farming practices, which could be a barrier for many. Still, experimentation and technological advancements can pave the way for more applicable solutions in the near future.
Multi-use Land Strategies
Multi-use land strategies represent a broader vision for integrating renewable energy installations into existing landscapes without overwhelming them. By employing techniques such as land-sharing, solar panels can coexist with recreational spaces, grazing pastures, or even conservation areas.
What stands out about these strategies is their flexibility. They allow energy production to flourish while maintaining the integrity of the land. This becomes a beneficial choice because it alleviates the pressure on untouched natural areas and conserves essential habitats.
A unique feature of multi-use land strategies is the promotion of ecological coexistence. For instance, solar farms designed to run alongside existing ecosystems not only reduce land use conflicts but can enhance local biodiversity by providing additional habitats or food sources for wildlife.
Nevertheless, one must critically assess the disadvantages of this approach, such as the potential for conflicting land uses that could arise, leading to tensions between different stakeholders. Thus, fostering dialogue and collaboration among land users is vital for the success of these innovative practices.
"Balancing the need for renewable energy with ecological preservation is not just an environmental issue, but a moral imperative for future generations."
"Balancing the need for renewable energy with ecological preservation is not just an environmental issue, but a moral imperative for future generations."
End-of-Life Challenges
The end-of-life phase of solar panels poses several significant challenges that cannot be overlooked. As the global push for renewable energy garners momentum, understanding how solar panels are managed at the end of their operational lifespan becomes crucial. With millions of panels set to be decommissioned in the coming years, addressing these challenges is not merely an environmental concern but a pressing need for sustainable resource management. Proper handling of obsolete solar panels has ramifications that affect landfill overcrowding, recycling efficiency, and environmental pollution. It's vital to highlight specific elements related to the end-of-life challenges and their broader implications in order to grasp the full ecological footprint of solar technology.
Recycling Issues
Current Recycling Practices
Current recycling practices for solar panels are evolving, but still lag behind other recycling industries. Primarily, the methods used today involve breaking down panels into their constituent parts, which includes glass, metals, and silicon. This process can recover about 90% of the materials in the panels, which is a noteworthy figure. However, the effectiveness varies significantly depending on the technology used. Many facilities specialize in recycling traditional crystalline silicon panels but do not adequately handle newer thin-film technologies.
A key aspect of these practices is that they bring benefits, like reducing the accumulation of waste in landfills and conserving resources. But they also present unique challenges. For instance, the recycling process can be costly and technically demanding, limiting widespread adoption. Additionally, the quality of recycled materials may not always meet the industry standards required for producing new panels, potentially leading to a cycle of inefficiency.
- Benefits:
- Challenges:
- Resource Recovery: The ability to reclaim valuable materials helps reduce the need for new raw materials.
- Environmental Mitigation: Decreases landfill waste and reduces the harmful impact of discarded panels.
- Costly Methods: The complexity and overhead of recycling processes restrict access.
- Material Quality: Recycled materials might not fulfill the required purity for new products.
Recycling Technologies
Innovations in recycling technologies are starting to emerge, shifting the landscape and offering potentials for better management of end-of-life solar panels. Some companies are developing closed-loop systems that not only recycle the materials but also reintegrate them into the production of new panels. This circular economy approach is significant because it ensures that materials remain in use and do not go back into the environment.
The characteristic feature of these technologies lies in efficiency and scalability. As they advance, they promise to lesser the cost and increase the reliability of recycling processes. Unique methods, such as laser ablation and proprietary chemical solutions, show promise in yielding high-quality silicon and metals without significant quality degradation.
- Advantages:
- Considerations:
- Higher Efficiency: New technologies can process panels faster and with better material recovery rates.
- Scalability: As facilities adopt these methods, the practices can become more widespread and accessible to smaller companies.
- Investment Costs: Initial research and development costs may be high for emerging technologies.
- Technical Knowledge: May require specialized staff and insight into advanced recycling methods.
Landfill Concerns
Decomposition of Materials
The decomposition of materials in landfills is a crucial concern in the context of solar panel disposal. When panels reach the landfill, the degradation process can take decades, if not longer. Components like glass and metals do not break down easily and can contribute to long-term land and groundwater contamination.
A distinguishing feature of landfill deposits is the significant volume that needs to be managed. Current disposal practices often lead to ineffective resource use and ecological degradation. With solar panels typically sealed and non-biodegradable, their presence in landfills becomes a long-term issue that municipalities must grapple with.
- Concerns:
- Land Pollution: More panels in landfills result in reduced land availability for other uses.
- Leachate Risk: Chemicals from the panels can leach into soil and water supplies.
Long-term Environmental Effects
Long-term environmental effects of solar panel disposal are a crucial aspect of the discussion on sustainability. The accumulation of solar panels in landfills creates a twofold problem—first, they occupy space that could be used for other purposes, and second, they pose potential risks to ecosystems. Heavy metals and chemical compounds found in panels, such as cadmium and lead, can leach into the surrounding environment over time, posing risks to wildlife and human health.
A notable characteristic of these environmental effects is their often invisible nature. For many people, the consequences might not be apparent at first glance, but over years, these contaminants can accumulate and lead to significant ecological damage. Awareness of this slow degradation process emphasizes the need for proactive measures in solar panel lifecycle management.
- Implications:
- Ecosystem Damage: Contaminants can disrupt local ecosystems and wildlife populations.
- Human Health Risks: Possible leaching into drinking water may pose long-term health risks to communities.
Addressing these end-of-life challenges is not just an operational or regulatory issue but a vital element in the broader context of adopting solar energy. While the energy generated is largely renewable, the complete lifecycle from production to disposal must be meticulously managed to ensure that the transition to solar energy does not come with its own set of environmental problems.
Regulation and Policy Implications
Regulation and policy implications are crucial in addressing the environmental challenges posed by solar panel production and disposal. As the push for renewable energy continues to intensify, it becomes essential to establish clear guidelines that govern not just the manufacturing processes, but also the end-of-life strategies for solar panels. Without appropriate regulations in place, inefficiencies and potential harms can snowball, leading to significant environmental repercussions. This section will delve into the current regulations in place, international agreements impacting solar energy, and propose future policy changes that can enhance sustainability in the industry.
Current Regulations Governing Production
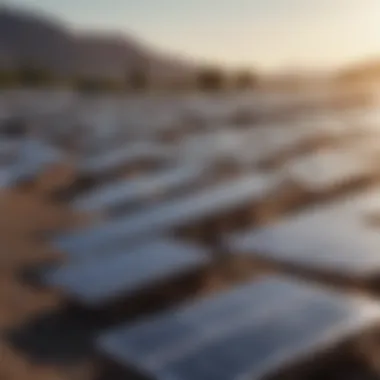
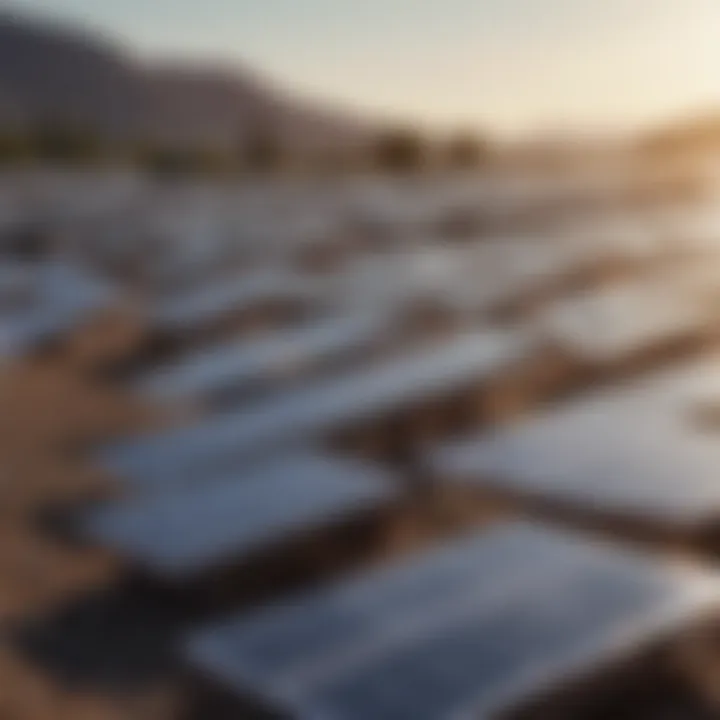
Government Standards
Government standards offer a framework aimed at minimizing the ecological impacts of solar panel production. These regulations frequently focus on emission controls, waste management, and the safety of materials used in manufacturing. One key characteristic of government standards is their local applicability; each country develops its own set of rules based on unique environmental needs and industrial capabilities. An example is the Clean Air Act in the United States, designed to regulate air emissions from various sectors, including solar manufacturers. The main benefit of these standards is their adaptability; they can evolve in response to technological advances and public health considerations.
However, there's a flip side. Strict standards can hinder innovation by limiting the materials and processes companies can utilize. Startups, in particular, may find it hard to navigate compliance with these constraints, which might discourage investment in new technologies.
International Agreements
International agreements play a pivotal role in aligning global efforts toward sustainable solar production. These agreements, such as the Paris Agreement, urge nations to commit to reducing greenhouse gas emissions comprehensively. A notable characteristic here is the cooperative framework; countries are encouraged to share technological advancements and best practices. This collaboration can spur innovation and lead to more effective sourcing of renewable technologies.
The uniqueness of these agreements lies in their emphasis on accountability. Countries reporting their progress on sustainability can set benchmarks for others to follow. However, the downside is evident when nations with less stringent regulations can essentially act as havens for less eco-friendly manufacturing practices, which could undermine global efforts.
Proposed Policy Changes
Innovations in Regulations
Innovations in regulations can significantly enhance the effectiveness of existing policies governing solar panel production and disposal. The trend is moving toward a more adaptive regulatory environment, where flexibility is prized. The rise of technologies like blockchain can introduce transparency in supply chains, which in turn strengthens compliance with environmental guidelines. Such innovations enable regulators to track materials and processes in real time, making it easier to enforce standards.
One key aspect of these innovations is the incorporation of stakeholder feedback, which means regulations do not merely conform to bureaucratic timelines; they grow organically based on industry needs and ecological outcomes. Therefore, it's quite a beneficial choice, as it aligns both economic and environmental goals, promoting shared responsibility.
Yet, the challenge remains in ensuring that there are sufficient incentives for companies to adopt these innovative practices. Without clear benefits or support, organizations may hesitate to commit to necessary changes.
Incentives for Sustainable Practices
Incentives for sustainable practices can drive the solar industry towards more ecologically friendly production and end-of-life management. These incentives may come in the form of tax breaks, grants, or subsidies for companies adopting green technologies and practices. A key characteristic is their proactive approach; instead of merely enforcing compliance, they encourage behavioral changes beneficial to the environment.
The uniqueness of these incentives is that they create a win-win scenario. Companies are motivated to innovate and upgrade their processes, leading to better environmental outcomes while enhancing their market competitiveness. The challenge is ensuring these incentives are enough to motivate widespread change. If not suitably funded or well-structured, they might end up benefiting only a small number of companies, leaving the majority to stick with less sustainable practices.
End
In wrapping up this exploration of the environmental consequences linked to solar panel production and disposal, it becomes evident that while solar energy is often lauded as a beacon of sustainability, significant challenges lurk beneath the surface. One must appreciate the nuanced balance between harnessing solar energy and mitigating its ecological footprint. The discussions here zero in on vital aspects ranging from the toxic materials used in manufacturing to the grave implications of improper disposal practices.
The information elucidated throughout this article serves several purposes. First, it raises awareness about the hidden costs of solar technology, drawing attention to the harmful substances such as cadmium and lead that pose risks not just to the environment but also to human health. These elements frequently get swept under the rug in conversations about renewable energy, yet understanding their impact is crucial for informed decision-making.
Second, the examination of land utilization underscores an essential consideration. Transitioning to solar energy frequently necessitates significant land conversion, which can lead to habitat destruction and loss of biodiversity. By understanding these trade-offs, stakeholders can pursue more equitable and judicious land use strategies, integrating solar systems within existing landscapes more sensitively.
Lastly, addressing sustainability in the end-of-life phase of solar panels can’t be overstated. A lack of robust recycling processes leads to accumulating panel waste that threatens groundwater and soil health. By advocating for innovations in recycling technology and promoting a circular economy, the industry can move towards a future where solar energy generation does not translate to ecological compromise.
"Understanding these aspects better equips us to tackle the environmental ramifications of solar technology while striving for cleaner energy solutions."
"Understanding these aspects better equips us to tackle the environmental ramifications of solar technology while striving for cleaner energy solutions."
Ultimately, the call to action for researchers, legislators, and manufacturers is clear: to engage critically with the multifaceted ramifications of solar panels. By prioritizing sustainable practices across all stages—from production to disposal—we can harness solar energy effectively without sacrificing the integrity of our environment.
Future Directions in Sustainable Solar Energy
The conversation around solar energy is shifting beyond its traditional frameworks, focusing on how advancements can minimize environmental trade-offs associated with solar panel production. Future directions include innovative materials and circular economy approaches, each playing a critical role in enhancing sustainability. This ongoing evolution is imperative, not just to improve efficiency but also to mitigate the ecological footprint that solar technology currently carries. The relevance of these themes is paramount, especially as societies strive for cleaner energy solutions in a world already grappling with the consequences of climate change.
Innovative Materials and Technologies
Organic Photovoltaics
Organic photovoltaics (OPVs) stand out as an interesting alternative to conventional silicon-based panels. One key characteristic of OPVs is their ability to be manufactured using low-cost materials, which can be processed at lower temperatures. This characteristic makes their production less energy-intensive, aligning well with sustainability goals. However, one unique feature of OPVs is their flexibility, allowing them to be integrated into various surfaces, from building facades to textiles.
Despite these advantages, OPVs are still catching up in terms of efficiency. Their energy conversion rates typically lag behind traditional solar cells, and their longevity remains a point of ongoing research. Nevertheless, the potential for lower manufacturing costs and wider application makes them a compelling option in the solar energy landscape.
Perovskite Solar Cells
Perovskite solar cells (PSCs) are also gaining traction, owing to their remarkable efficiency and the variety of materials used in their production. The primary allure of PSCs lies in their rapid advancement in energy conversion efficiencies, frequently achieving performance levels approaching those of silicon panels. The unique feature of perovskites is their tunable bandgap, which allows for customization in energy absorption for a range of light conditions, making them adaptable for different environments.
However, the benefits come hand in hand with challenges. The long-term stability of PSCs remains an issue, as environmental factors can degrade their materials quickly. Additionally, some formulations involve materials that might pose health risks, furthering the dialogue on sustainable practices in solar technology. Despite these hurdles, PSCs' advantages make them essential players in the future of sustainable solar energy.
Circular Economy Approaches
Design for Disassembly
Design for disassembly is a key aspect in promoting sustainability within solar technology. This approach advocates for solar panels to be made with future disassembly in mind. A significant advantage of this method is that it allows for easier repair and recycling of components, which contributes to minimizing waste. Creating solar products that can be taken apart without damaging parts ensures that valuable materials are not lost.
However, implementing such designs can increase production costs upfront. Finding the right balance between cost and sustainability is crucial. Still, as more manufacturers adopt this philosophy, the industry can expect a shift toward more responsible production cycles.
Lifecycle Assessment
Lifecycle assessment (LCA) is another vital tool to understand the environmental impacts of solar panels from production through disposal. This method evaluates the entire lifecycle of a product, providing insights into energy use, emissions, and resource consumption throughout its existence. The key characteristic of LCA is that it offers a comprehensive perspective on how solar panels affect the environment at each stage, revealing opportunities for improvement.
By incorporating LCA into production practices, companies can identify areas where they can decrease their environmental footprint, leading to more sustainable choices. Nonetheless, the complexity of conducting an effective LCA can be daunting for many organizations, which often lack the resources or expertise. As awareness of LCA grows, however, it could pave the way for significant enhancements in how solar panels are manufactured and disposed of, driving the industry toward greater accountability and ethical practices.
The pursuit of sustainability in solar energy technology is no longer optional; it is imperative for ecological integrity and energy evolution.
The pursuit of sustainability in solar energy technology is no longer optional; it is imperative for ecological integrity and energy evolution.
As these new directions unfold, they not only enhance the appeal of solar energy but also contribute to a wider movement toward genuinely sustainable solutions. More innovative materials and introspection into the lifecycle of energy technologies highlight the fact that the journey towards sustainable solar energy is intricate but vital for future generations.