Exploring CNT Carbon Nanotubes: An In-Depth Analysis
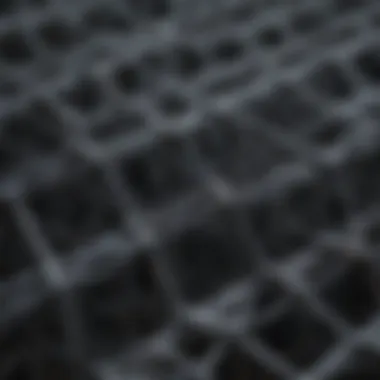
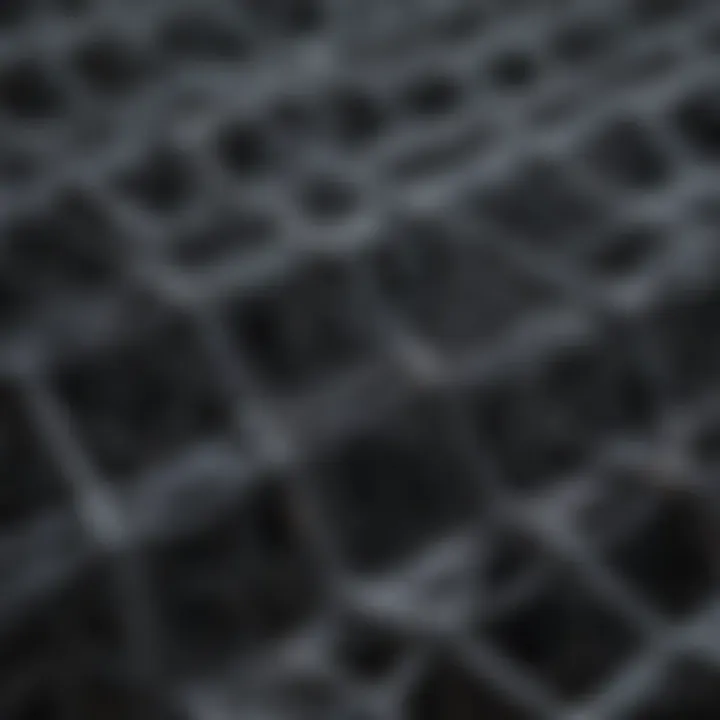
Article Overview
Purpose of the Article
The aim of this article is to provide a thorough examination of carbon nanotubes (CNTs). By offering insights into their structure, properties, and a wide range of applications, this article seeks to enrich the understanding of CNTs for a scientifically literate audience. The discourse will cover their synthesis methods, functionalization techniques, and the critical role they play in diverse industries. In doing so, it will both highlight achievements and address the ongoing challenges in utilizing carbon nanotubes effectively.
Relevance to Multiple Disciplines
CNTs are not only significant in materials science but also have implications across various fields. Their remarkable properties make them valuable in sectors like electronics, energy storage, and biotechnology. As knowledge surrounding CNTs expands, their potential applications continue to evolve, which warrants exploration from a multidisciplinary perspective.
Research Background
Historical Context
The discovery of carbon nanotubes in 1991 by Sumio Iijima marked a pivotal moment in nanotechnology. Initially regarded as mere curiosities, these cylindrical structures soon revealed extraordinary mechanical, thermal, and electrical properties. Research has burgeoned ever since, evolving into a focus on practical applications ranging from lightweight materials to advanced drug delivery systems.
Key Concepts and Definitions
Carbon nanotubes are hollow cylinders composed of carbon atoms arranged in a hexagonal lattice. CNTs can primarily be categorized into two types: single-walled carbon nanotubes (SWCNTs) and multi-walled carbon nanotubes (MWCNTs). The former consists of a single layer of carbon atoms, while the latter contains multiple layers.
- Mechanical Strength: CNTs exhibit a strength-to-weight ratio significantly greater than steel, making them ideal in structural applications.
- Electrical Conductivity: Depending on their chirality, CNTs can behave as conductors or semiconductors, paving the way for advanced electronic components.
- Thermal Conductivity: Their excellent thermal conductivity renders them useful in thermal management systems.
The significance of CNTs is underscored by their capacity to bridge gaps between theoretical potential and practical applications, warranting further investigation into their capabilities and limitations.
Preface to Carbon Nanotubes
Carbon nanotubes (CNTs) have gained attention due to their remarkable properties and potential applications across various fields. Understanding their basic characteristics is essential for grasping why they are crucial in today's scientific discussions. This section provides insight into the definition, structure, and types of carbon nanotubes.
Definition and Structure
A carbon nanotube is a cylindrical nanostructure made entirely of carbon atoms arranged in a hexagonal pattern. They are typically one nanometer in diameter, which is much smaller than human hair. The walls of these tubes can be single-layered (single-walled nanotubes, or SWCNTs) or multi-layered (multi-walled nanotubes, or MWCNTs). The distinct arrangement of carbon atoms contributes to their impressive mechanical, electrical, and thermal characteristics.
The structure is often described as rolled-up sheets of graphene. Graphene itself is a single layer of carbon atoms arranged in a two-dimensional lattice. The unique rolling of the graphene sheets gives carbon nanotubes their unique properties, such as extraordinary strength and high electrical conductivity.
The configuration of carbon atoms also yields two main types of carbon nanotubes based on their chirality, which fundamentally affects their electronic properties. The angle at which the graphene sheet is rolled determines if the nanotube exhibits metallic or semiconducting behavior.
Types of Carbon Nanotubes
There are primarily two types of carbon nanotubes: single-walled carbon nanotubes (SWCNTs) and multi-walled carbon nanotubes (MWCNTs). Each type presents different characteristics and applications.
- Single-Walled Carbon Nanotubes (SWCNTs)
- Multi-Walled Carbon Nanotubes (MWCNTs)
- These consist of a single layer of graphene. Their diameter is approximately one nanometer, making them remarkably small. SWCNTs can exhibit metallic or semiconducting properties, which makes them suitable for a variety of electronic applications.
- MWCNTs are composed of multiple graphene layers, arranged concentrically. The inner tubes can be several nanometers in diameter, while the outer diameter can reach tens of nanometers. MWCNTs generally have greater mechanical strength and thermal stability compared to SWCNTs.
The choice between SWCNTs and MWCNTs depends on the intended application, particularly in electronics, nanomedicine, and materials science. Understanding these differences provides a foundation for exploring how carbon nanotubes can be utilized in advanced technologies.
Physical Properties of CNTs
The physical properties of carbon nanotubes (CNTs) are critical to their functionality and applicability in various fields. Their unique structure allows them to exhibit remarkable characteristics that can be exploited in numerous technological and scientific disciplines. Understanding these properties enables researchers and professionals to tailor CNTs for specific applications, enhancing both performance and efficiency. Key physical properties to consider include mechanical strength, electrical conductivity, and thermal conductivity, each contributing to the overall versatility of CNTs in industrial and research settings.
Mechanical Strength
Carbon nanotubes possess exceptional mechanical strength, surpassing that of steel by many times while being significantly lighter. This property arises from the strong carbon-carbon bonds within their structure. The tensile strength of CNTs can reach up to 100 GPa, making them ideal candidates for reinforcing materials.
Applications such as composite materials benefit from the high strength-to-weight ratio that CNTs offer. For instance, integrating CNTs into plastics can produce materials that maintain structural integrity under stress while reducing overall weight. Furthermore, their flexibility allows them to be used in applications where sheer rigidity is not ideal.
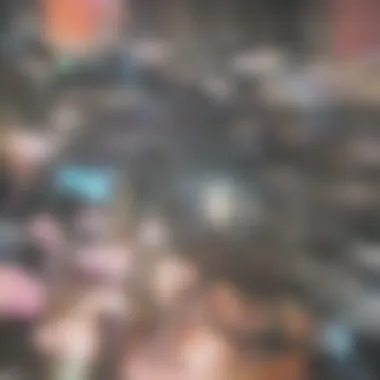
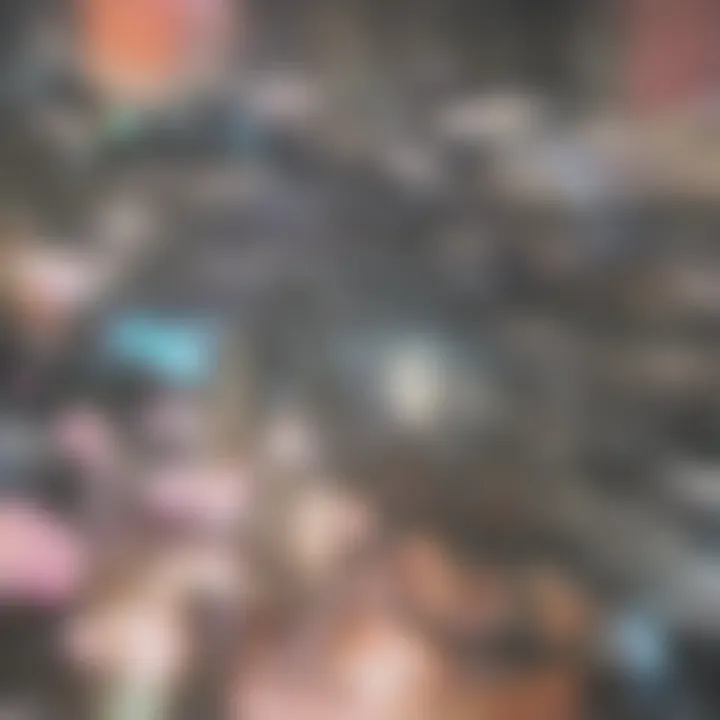
Electrical Conductivity
Another noteworthy property of CNTs is their electrical conductivity. Depending on their structure (single-walled or multi-walled), they can behave as either conductors or semiconductors. This property is pivotal for the advancement of electronic devices.
CNTs exhibit high electrical current carrying capacity, reducing energy loss in electronic circuits. This quality positions them as excellent candidates for use in transistors, sensors, and other semiconductor applications. Furthermore, the field-effect transistors made from CNTs highlight their potential for high-speed electronics, which is increasingly vital in modern communication technologies.
Thermal Conductivity
Thermal conductivity is yet another critical attribute of CNTs. They exhibit remarkable thermal conductivity comparable to that of diamond. This effective heat transfer capability allows CNTs to dissipate heat efficiently, making them particularly useful in thermal management applications.
In electronics, CNTs can help mitigate overheating in devices, enhancing their performance and lifespan. Their thermal properties also make them advantageous in advanced materials that require efficient heat treatment processes.
"The combination of high mechanical strength, excellent electrical conductivity, and superior thermal conductivity makes CNTs a material of great interest across multiple disciplines."
"The combination of high mechanical strength, excellent electrical conductivity, and superior thermal conductivity makes CNTs a material of great interest across multiple disciplines."
Overall, the physical properties of carbon nanotubes are not just intrinsic characteristics; they are fundamental to pushing the boundaries of technology and materials science. As research continues to evolve, further insights into these properties can lead to novel applications and innovations.
Synthesis of Carbon Nanotubes
The synthesis of carbon nanotubes is a critical aspect of their development and application in various fields. It helps determine the quality, structural characteristics, and ultimately the performance of CNTs. Synthesis methods also influence their scalability, cost-effectiveness, and suitability for specific applications. Understanding the synthesis techniques offers insights into how CNTs can be tailored to meet the demands of industries such as electronics, materials science, and nanotechnology.
Chemical Vapor Deposition ()
Chemical Vapor Deposition, often referred to as CVD, is one of the most widely used methods for synthesizing carbon nanotubes. This technique involves the chemical reaction of gaseous precursors, which decompose to deposit carbon on a substrate. The process occurs in a heated environment, allowing for the growth of CNTs on various materials.
CVD offers several benefits:
- Control over Growth: By adjusting temperature and pressure, researchers can control the diameter and length of the nanotubes.
- High Purity: The method allows for the production of high-purity CNTs, which is essential for many applications.
- Scalability: CVD can be scaled up for mass production, making it attractive for commercial purposes.
However, it is essential to manage factors such as reaction time, precursor type, and reactor design to optimize results. In summary, CVD remains a cornerstone technique in the synthesis of CNTs, paving the way for advancements in their applications.
Laser Ablation
Another prominent method for synthesizing carbon nanotubes is laser ablation. In this technique, high-powered lasers vaporize a graphite target in the presence of inert gases. The resulting vapor cools and condenses to form CNTs.
Laser ablation has some notable advantages:
- Quality of CNTs: This method generally produces high-quality and well-structured carbon nanotubes.
- Control over Morphology: Researchers can manipulate the morphology and purity of the nanotubes.
- Potential for Novel Structures: It allows for the synthesis of unique forms and configurations that may not be achievable through other methods.
However, laser ablation also poses challenges, such as higher production costs and the need for sophisticated equipment, which may limit its widespread use in industry.
Arc Discharge
The arc discharge method involves creating an electric arc between two carbon electrodes in an inert gas atmosphere. The intense heat generated by the arc vaporizes the electrodes, producing a carbon vapor that condenses to form carbon nanotubes. This technique is known for its simplicity and relatively low cost.
Key aspects of arc discharge include:
- Rapid Production: It allows for the quick synthesis of large quantities of CNTs, making it suitable for initial research and experimentation.
- Diverse Structures: The process can produce different types of nanotubes, including single-walled and multi-walled structures.
Nevertheless, challenges such as the need for purification and the presence of amorphous carbon remain prominent.
Functionalization of CNTs
Functionalization of carbon nanotubes (CNTs) is a critical area of study that focuses on modifying their surfaces to enhance their chemical properties and expand their applicability in various fields. This process involves attaching different functional groups to the CNTs. The reasons for doing so are manifold, including improving dispersion in solvents, enhancing interaction with other materials, and tailoring electrical and mechanical properties for specific purposes. A better understanding of functionalization is essential, as it bridges the gap between the fundamental properties of CNTs and their practical applications in technology, medicine, and materials science.
Types of Functionalization
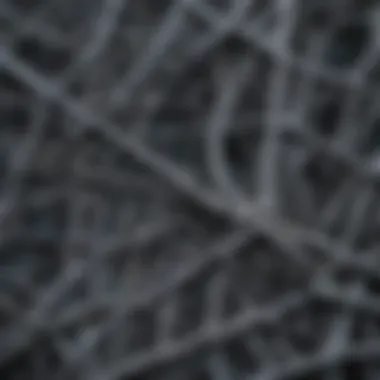
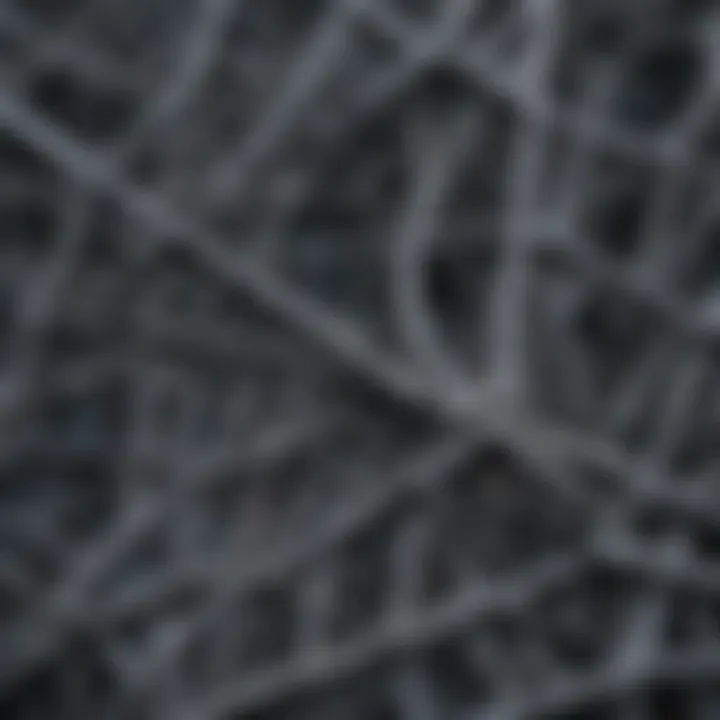
There are several techniques for the functionalization of CNTs, each with its advantages and disadvantages. Some common methods include:
- Covalent functionalization: Involves chemical reactions that create strong bonds with functional groups. This method offers stability but may alter the inherent properties of CNTs.
- Non-covalent functionalization: Utilizes physical interactions, such as van der Waals forces and Ο-Ο interactions. This technique is less invasive, preserving the CNT structure while still enhancing solubility and reactivity.
- Plasma treatment: Exposes CNTs to plasma, leading to the introduction of various functional groups on their surfaces. This method is effective for uniformly modifying the surface.
- Grafting techniques: Involves attaching polymer chains to the surface of the CNTs, which can improve compatibility with polymers and enhance mechanical properties.
Each of these functionalization techniques has unique considerations that affect the performance of CNTs in real-world applications.
Effects on Properties
Functionalization has a profound effect on the properties of carbon nanotubes. Some notable effects include:
- Improved dispersion: Functional groups attached to the CNTs enhance their solubility in various solvents, preventing aggregation and facilitating uniform distribution in composites.
- Altered electrical conductivity: Depending on the type of functional group introduced, the electrical properties can either be enhanced or diminished. For instance, introducing electron-withdrawing groups can reduce conductivity, while adding materials that donate electrons can enhance it.
- Enhanced mechanical strength: Certain functionalization methods increase the load-bearing capacity of CNT composites. This is particularly important in applications where structural integrity is crucial.
- Biocompatibility: For medical applications, functionalization can render CNTs more compatible with biological systems. By modifying their surface to attach biocompatible molecules, CNTs can be utilized in drug delivery systems or tissue engineering.
Functionalization enables the adaptation of carbon nanotubes for specific applications while maintaining their fundamental characteristics.
Functionalization enables the adaptation of carbon nanotubes for specific applications while maintaining their fundamental characteristics.
Applications of Carbon Nanotubes
The applications of carbon nanotubes (CNTs) represent a significant area of interest within materials science, due to their unique properties and versatility. Their ability to exhibit exceptional strength, electrical conductivity, and thermal properties makes them suitable for various industries. Understanding these applications can provide insight into how CNTs can revolutionize technology and improve current systems.
Electronics and Semiconductors
Carbon nanotubes are increasingly utilized in the field of electronics and semiconductors. Their high electrical conductivity and nanoscale size facilitate the development of smaller and more efficient electronic devices. For instance, CNTs are often seen as potential replacements for silicon in transistors. These nanotubes can operate at higher speeds, which is crucial for modern computing requirements. Furthermore, their flexibility can lead to innovative designs in flexible electronics, such as bendable displays and ultra-thin solar cells.
The incorporation of CNTs into materials can enhance the performance of various semiconductor devices, offering benefits like reduced energy consumption and improved thermal management. However, challenges remain in aligning and processing CNTs in a way that optimizes these properties for commercial use.
Energy Storage and Conversion
In the domain of energy storage and conversion, carbon nanotubes are proving to be a game-changer. Their high surface area and conductivity make them ideal materials for enhancing the efficiency of supercapacitors and batteries. CNTs can be used to create electrodes that store more energy and charge faster, addressing two critical limitations of traditional energy storage systems.
In lithium-ion batteries, for instance, CNTs can significantly improve both charge capacity and cycling stability. Moreover, they facilitate the development of lightweight materials for electric vehicles, thus contributing to energy efficiency. Researchers are exploring their potential in hydrogen storage as well, aiming for efficient fuel cells that can foster sustainable energy solutions.
Nanocomposites and Materials Science
In materials science, carbon nanotubes are being integrated into nanocomposites to enhance mechanical and thermal properties. By reinforcing materials like polymers and metals, CNTs contribute to creating materials that are lighter, stronger, and more resilient. These improvements can lead to advancements in industries including aerospace, automotive, and construction.
The use of CNTs in composites can also lead to better thermal conductivity, which is essential in applications requiring heat dissipation. Such innovations indicate a promising future for materials designed to meet specific industry needs while reducing weight and improving performance.
Biomedicine
The biomedicine field has seen a growing interest in the use of carbon nanotubes due to their unique properties. CNTs can be utilized for drug delivery systems, facilitating targeted delivery to specific cells or tissues. This precision can improve the efficacy of treatments while minimizing side effects associated with traditional drug delivery methods.
Moreover, carbon nanotubes are explored for their potential in imaging and diagnostic applications. As contrast agents, they can enhance the signals in imaging techniques like MRI, providing clearer visuals and better diagnostics. However, concerns regarding the toxicity of CNTs need to be addressed to ensure their safe application in healthcare.
In summary, carbon nanotubes hold transformative potential across multiple fields including electronics, energy, materials science, and biomedicine. Proper exploration and understanding of these applications can shape future technological advancements.
In summary, carbon nanotubes hold transformative potential across multiple fields including electronics, energy, materials science, and biomedicine. Proper exploration and understanding of these applications can shape future technological advancements.
Challenges and Limitations
As the field of carbon nanotubes (CNTs) continues to evolve, it is essential to address the various challenges and limitations that accompany their exploitation in diverse applications. Despite their remarkable properties and potential, such hurdles could impede practical implementation. Understanding these challenges not only highlights the areas needing attention but also paves the way for future advancements and innovations. Among the most pressing issues are toxicity and environmental concerns, scalability of production, and the integration of CNTs with existing technologies. Each of these aspects requires thorough investigation and targeted research to fully leverage the advantages of CNTs.
Toxicity and Environmental Concerns
Toxicity is a significant issue in any discussion surrounding new materials, and carbon nanotubes are no exception. Laboratory studies suggest that CNTs can induce various forms of cellular and tissue damage due to their unique structure and properties. While they exhibit high biocompatibility in certain applications, the potential for respiratory harm or adverse biological interactions when ingested poses a serious concern for safety.
Moreover, the environmental impact of CNTs must be considered. The synthesis processes can result in waste products and contaminants which may prove harmful if they enter ecosystems. The accumulation of these materials in the environment could lead to unforeseen consequences, such as bioaccumulation in organisms. Regulatory bodies and researchers must investigate the implications of CNT usage, ensuring that their advantages do not come at an unbearable ecological cost. In addressing these concerns, collaborative work between material scientists, toxicologists, and environmental engineers is vital.
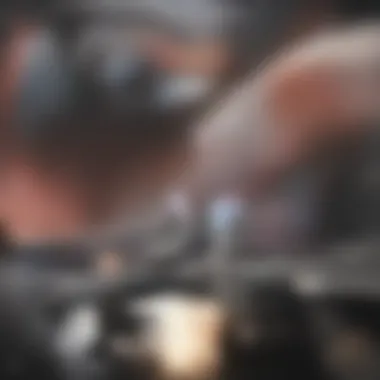
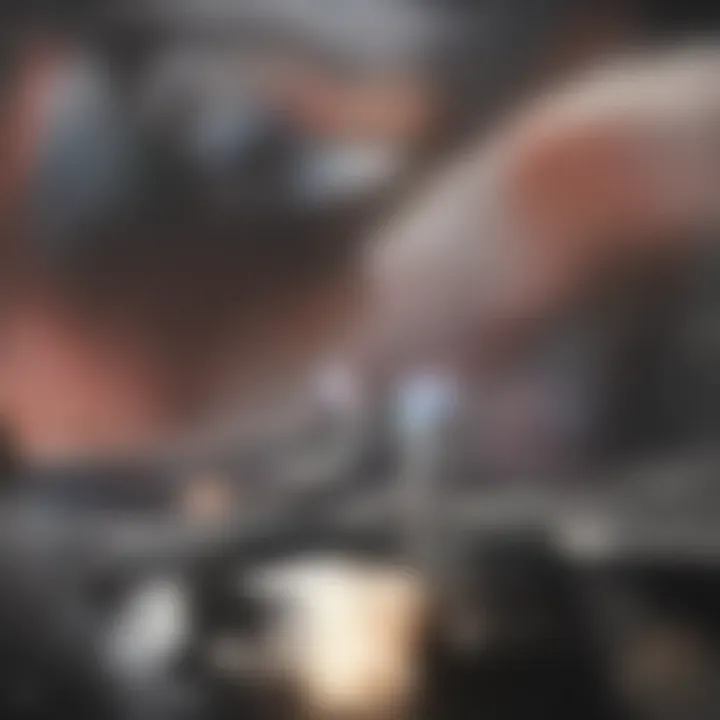
Scalability of Production
While techniques such as Chemical Vapor Deposition and laser ablation are well-established methods for producing CNTs, scaling these processes for large-scale commercial production remains a challenge. Research has shown that achieving high purity and uniformity becomes increasingly difficult as the quantity produced rises. The cost of raw materials, along with the necessity for specialized equipment, further complicates the scalability issue.
Efforts to develop more cost-efficient synthesis methods are ongoing. Innovative approaches include the use of alternative precursors and reaction conditions to enhance yield without compromising quality. As the demand for CNTs grows in industries like electronics and biotechnology, finding a feasible balance between production scale and cost-effectiveness becomes crucial.
Integration with Existing Technologies
Integrating CNTs into current technology frameworks presents its own set of challenges. Existing manufacturing processes in various industries may not be readily adaptable for CNT incorporation. For example, CNTs often require specific handling conditions due to their unique properties, such as their sensitivity to defects or environmental variations.
Furthermore, compatibility with traditional materials and manufacturing practices can hinder the implementation of CNT-enhanced products. Research on tailored composite materials can potentially address these issues, but this requires investment in both time and resources. A successful integration often involves multi-disciplinary approaches, merging insights from materials science, engineering, and process management to create viable pathways for CNT adoption.
"For the technology surrounding CNTs to flourish, a comprehensive understanding of these challenges is essential to developing viable solutions."
"For the technology surrounding CNTs to flourish, a comprehensive understanding of these challenges is essential to developing viable solutions."
By recognizing and addressing these hurdles, researchers and industry professionals can work towards realizing the promise that carbon nanotubes hold for the future.
Future Directions in Research
The realm of carbon nanotubes (CNTs) holds significant promise for advancing numerous fields. As researchers investigate the capabilities, properties, and applications of CNTs, the focus shifts toward future directions that could transform both academic inquiry and practical implementation.
Innovations in Synthesis Techniques
Developing new synthesis methods is crucial for enhancing the properties and functionality of carbon nanotubes. Traditional methods like Chemical Vapor Deposition (CVD) have paved the way in CNT production. However, innovations are ripe for exploration.
More precise control over parameters during synthesis can lead to tailored structures with specific properties. For instance, advancements in laser ablation could yield CNTs with improved purity and fewer defects. Researchers are also looking into pulsed lasers and alternative reactants to widen the scope of synthesis.
Another promising approach is the use of biogenic methods, harnessing microorganisms to produce CNTs. Such methods could offer safer and more eco-friendly alternatives compared to traditional chemical processes.
Interdisciplinary Applications
Carbon nanotubes are not confined to a single domain. Their versatile characteristics allow them to play a role in various interdisciplinary applications. In materials science, CNTs can vastly enhance the strength-to-weight ratio of composites. Applications may extend to aerospace, automotive, and even sports equipment, necessitating collaboration across fields.
Moreover, in the field of biomedicine, CNTs show potential in drug delivery systems, enabling targeted therapy. The collaboration between chemists, engineers, and biologists can yield innovative solutions addressing complex challenges.
"The ability of CNTs to bridge different scientific and engineering disciplines underscores their importance in future research endeavors."
"The ability of CNTs to bridge different scientific and engineering disciplines underscores their importance in future research endeavors."
Sustainability and Green Chemistry Approaches
Sustainability is increasingly becoming a focus in research and industry. As CNT applications expand, there is a pressing need to develop green chemistry principles around CNT production and usage.
Researchers are investigating methods that minimize waste and reduce environmental impact, aligning with global sustainability goals. This includes the development of cleaner synthesis routes and recycling methods for CNTs.
Additionally, utilizing natural resources as feedstocks for CNT synthesis can promote sustainability. The focus on green chemistry necessitates a deeper understanding of the effects of CNTs on ecosystems, leading to safer protocols in their application and disposal.
Closure
The conclusion of this article serves as a crucial touchpoint, drawing together the significant threads discussed throughout the analysis of carbon nanotubes (CNTs). It emphasizes the vital role that CNTs play in advancing various fields such as electronics, materials science, and biomedicine. Readers can appreciate the multifaceted advantages that these nanomaterials offer, particularly their exceptional mechanical and electrical properties. Moreover, it addresses the challenges and limitations that still hinder widespread application. This nuanced understanding is important for scholars, researchers, and industry professionals who are invested in pushing the boundaries of what is technologically feasible and environmentally sustainable.
Summary of Key Points
In summary, the article has covered several important aspects of carbon nanotubes:
- Definition and Structure: CNTs are unique cylindrical nanostructures made of carbon atoms arranged in a hexagonal lattice.
- Physical Properties: Exceptional mechanical strength, high electrical conductivity, and superior thermal conductivity were highlighted.
- Synthesis Methods: Critical techniques such as Chemical Vapor Deposition (CVD), laser ablation, and arc discharge were discussed.
- Functionalization: The modification of CNTs to enhance their properties became evident through various functionalization methods.
- Applications: Significant uses in electronics, energy sectors, and biomedicine, showcasing versatility.
- Challenges: Issues surrounding toxicity, environmental impact, and production scalability were critically examined.
- Future Directions: Innovations in synthesis and interdisciplinary applications indicated pathways for further research and improved outcomes.
Through this summary, the reader should recognize that carbon nanotubes not only have significant potential but also demand responsible development and integration into existing technologies.
The Future of CNT Technology
Looking ahead, the future of CNT technology appears promising yet complex. Research continues to identify new synthesis techniques that can simplify production and reduce costs. For example, utilizing green chemistry approaches to lessen environmental impact is becoming more important. Innovations in functionalization and application methods may yield enhanced performance in composites and electronics. The adaptability of CNTs suggests a revolution in fields like energy storage, where higher capacities and faster charge rates are sought.
Additionally, interdisciplinary collaborations will likely accelerate breakthroughs. Collaboration between material scientists, engineers, and environmentalists can foster more eco-conscious approaches. New regulatory frameworks are also essential to address safety concerns while promoting research.