Exploring the Chemical Significance of Iron
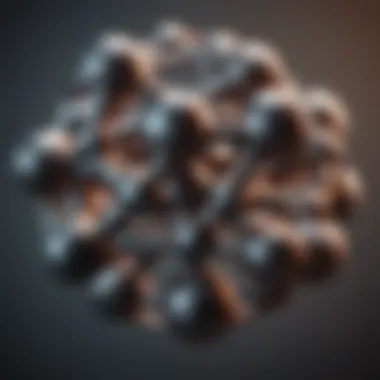
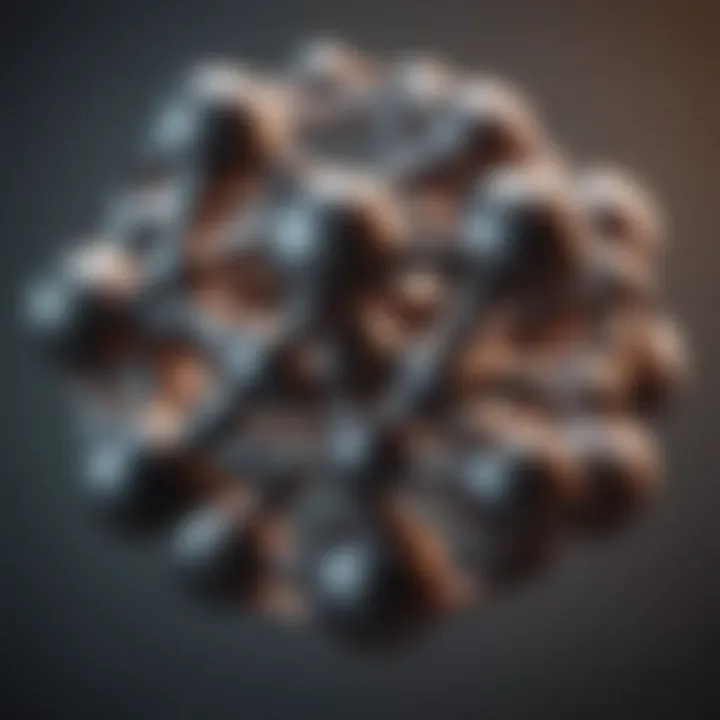
Intro
Iron occupies a unique and pivotal position in both the natural world and numerous scientific domains. Often considered one of the most abundant elements on Earth, its chemical formula may seem simple at a glance, yet it opens a Pandora's box of complexities. This article intends to dissect the chemical formula that represents iron, delve into its significance across various fields, and elucidate its profound role in both industrial applications and biological systems.
As we embark on this exploration, we will highlight critical points such as the derivation of iron's formula, its behavior in chemical reactions, and the broader implications of iron in ecology and human physiology.
Article Overview
Purpose of the Article
This article aims to provide a thorough understanding of iron, emphasizing its formula, relevant implications, and contextual significance. By linking theoretical knowledge with real-world applications, the discussion will guide readers through a spectrum of findings that relate to ironās multifaceted nature.
Relevance to Multiple Disciplines
Iron's versatility makes it applicable in various disciplines:
- Chemistry: Understanding iron's atomic structure and its bonding behavior can reveal much about its chemical properties.
- Biology: Iron is crucial for life; it plays vital roles in oxygen transport and energy production within living organisms.
- Engineering and Technology: Iron and its alloys are foundational in manufacturing and construction, impacting everything from infrastructure to aerospace.
As we journey through these topics, the readers will see how interconnected these fields are and the shared foundation upon which they rest.
Research Background
Historical Context
The journey of iron stretches back to ancient civilizations, where it was often prized for its use in weaponry and tools. Iron's discovery marks a significant chapter in human history, indicating technological advancement. By the late 19th century, the scientific understanding of iron began to blossom with emerging theories about atoms and molecules.
Key Concepts and Definitions
To grasp the significance of iron's formula fully, it's important to define a few key concepts:
- Atomic Structure: Iron is represented as Fe in the periodic table, possessing atomic number 26. The arrangement of protons, neutrons, and electrons gives rise to its distinctive properties.
- Oxidation States: Iron can exhibit multiple oxidation states, most prominently +2 and +3, influencing its behavior in compounds and reactions.
- Chemical Bonding: Understanding how iron interacts with other elements and forms various alloys is crucial for both biological and industrial applications.
Research into iron continues to evolve, pushing the boundaries of our understanding and revealing new applications that shape technology and life itself.
Intro to Iron
The significance of iron in various contexts cannot be overstated, as it plays a crucial role in both scientific inquiry and daily life. This section serves as a gateway to understanding the multifaceted nature of this essential element. From its foundational role in chemistry to its pivotal uses in industries and biology, iron manifests its importance in diverse domains. Without fail, almost every sector incorporates iron in some mannerābe it structural engineering, health sciences, or environmental studies.
The introduction lays the groundwork by touching upon key aspects that will be explored in greater depth. Not only does iron serve as a vital component in manufacturing and construction, but it is also integral to biological systems, particularly in human physiology. Furthermore, understanding iron's unique properties and its various compounds is paramount for any thorough exploration of its applications.
The benefits of grasping the underpinning concepts surrounding iron could be quite substantial:
- Scientific Grounding: A clear understanding of iron contributes to advancements in material science, biochemistry, and various engineering fields.
- Historical Context: Appreciating the historical discovery of iron provides insight into its evolving significance through the ages and how it has shaped human civilization.
- Practical Implications: Knowledge of ironās properties and behavior governs its effective utilization in industry and helps in mitigating environmental impacts related to its processing and usage.
Now, in the following subsections, we will delve into the specific role of iron in scientific disciplines and explore the historical milestones that frame our current understanding of this element.
The Role of Iron in Science
Iron's significance in the scientific realm extends across multiple disciplines. In the field of chemistry, it is often a prime example in discussions about transition metalsāits properties and reactions serve to illustrate a wider array of chemical principles. For instance, in catalysis, iron compounds can facilitate various reactions efficiently, making them integral to organic chemistry and materials science.
Additionally, in physics, iron is pivotal in studies of magnetism, where its unique atomic structure allows it to exhibit ferromagnetism. Its ability to form various oxidation statesāprimarily +2 and +3āimpacts its chemical behavior resulting in diverse applications.
The biological realm, too, cannot be overlooked. Iron is a critical component of hemoglobin, the molecule responsible for oxygen transport in the blood of many living organisms. This connection illustrates how deeply intertwined iron is with life itself.
Overall, iron encompasses a wealth of characteristics that scientists exploit in both theoretical and applied research.
Historical Context of Iron Discovery
The history of iron stretches back thousands of years, revealing a tale of innovation and adaptation. The knowledge of its properties has evolved over numerous erasāfrom the Copper Age, where alloys containing iron were discovered, to the later Iron Age, signaling a major shift in tools and weaponry development.
In terms of notable milestones, the smelting process, where iron ore is heated to separate the metal from its impurities, marks a pivotal development that propelled civilization forward. Ancient cultures, such as the Hittites, were pioneers in iron production, though the exact techniques remain somewhat shrouded in mystery.
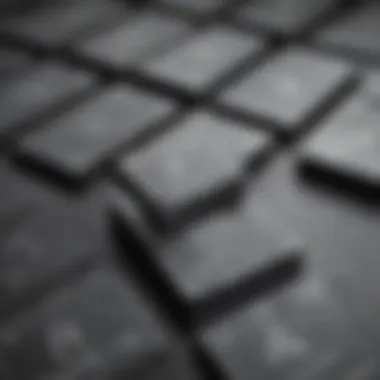
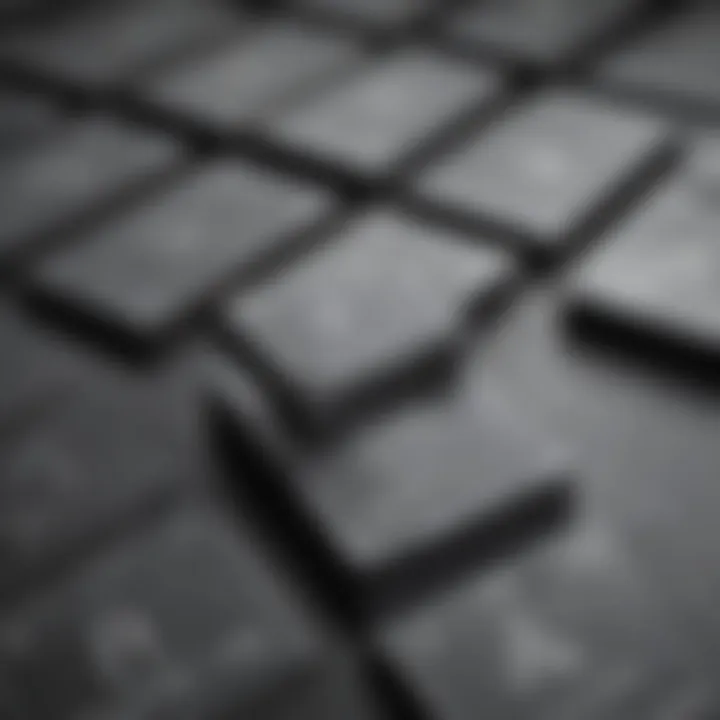
The advent of ironworking ignited an industrial revolution of sorts, enhancing agriculture and increasing construction capabilities. As societies progressed, the manipulation of iron has been a hallmark of innovation, continually evolving with advances in technology and knowledge.
Understanding these historical roots not only provides context but also allows us to appreciate how iron has shaped economies and societies. This backdrop is invaluable when we consider contemporary applications and environmental issues surrounding iron mining and processing.
"Throughout history, iron has been a cornerstone of human progress, demonstrating how material science can transform our way of life."
"Throughout history, iron has been a cornerstone of human progress, demonstrating how material science can transform our way of life."
Connecting these historical insights to present-day implications helps underscore the continuing relevance of iron, forging a path towards deeper exploration throughout the article.
The Chemical Formula of Iron
The chemical formula of iron is fundamental to understanding the properties and applications of this element in both science and industry. It reflects not just the structure of iron itself but also aids in comprehending its interactions with other substances. This section sheds light on the intricate relationship between iron's molecular composition and its vast implications.
Basic Characteristics of Iron
Iron, with the symbol Fe derived from the Latin word ferrum, is a transition metal notable for its strength and magnetic properties. This element typically exhibits a metallic luster and a silvery-grey appearance. It possesses several characteristics that distinguish it:
- Density and Strength: Iron is dense; its atomic weight is about 55.85 g/mol. This trait contributes to its use in construction and manufacturing.
- Malleability and Ductility: It can be hammered or bent without breaking, allowing it to be shaped into various forms, from rebar in construction to intricate components in machinery.
- Conductivity: Iron conducts heat and electricity well, making it valuable in electrical applications, such as wiring and motors.
Fe displays a prevailing stability and a comparatively low melting point, around 1538°C (2800°F). This makes it relatively straightforward to cast into different shapes for various uses.
Understanding Iron's Oxidation States
Iron exhibits multiple oxidation states, which are crucial for its reactivity and its role in various chemical reactions. The common states are +2 and +3, but it can also exist in other forms. The significance of these states lies in their applications and implications:
- Ferrous (Fe²+): In this lower oxidation state, iron is less stable and can easily react with oxygen or other elements. It's prominently observed in iron(II) sulfate, often used as a dietary supplement.
- Ferric (Fe³+): This higher oxidation state is seen in iron(III) oxide, commonly known as rust. Understanding this state is essential, particularly regarding corrosion and material degradation in various environments.
- Complexation: Iron's ability to form complex ions is significant in biology. For example, the iron in hemoglobin operates in the +2 oxidation state, allowing oxygen transport in blood.
"Iron's variability in oxidation states signifies its versatility in both chemical reactions and biological systems."
"Iron's variability in oxidation states signifies its versatility in both chemical reactions and biological systems."
In summary, the comprehension of iron's oxidation states is not merely an academic exercise; it has real-world significance spanning environmental chemistry, metallurgy, and biochemistry. Understanding these characteristics provides essential insights into the element's behavior, applications, and its interactions with other elementsāmaking it a cornerstone of various scientific fields.
Iron in the Periodic Table
Understanding the positioning of iron within the periodic table offers a window into its numerous chemical and physical properties. Iron holds a unique place as atomic number 26, resonating not only with its elemental qualities but also its extensive role in both biological and industrial contexts.
In the grand scheme of the periodic table, iron sits comfortably in the d-block, specifically classified among the transition metals. This classification hints at its varied valance states and propensity to form complex compounds. Iron's significance in various reactions, whether they're biological, geological, or even man-made, makes its placement paramount for deeper exploration.
Positioning of Iron Among Elements
Iron is situated between manganese (atomic number 25) and cobalt (atomic number 27). This arrangement on the periodic table is not merely a numeric one; it speaks volumes about iron's chemical behavior. A defining trait of iron is its potential oxidation states. Iron can exist in oxidation states of +2 and +3, making it exceptionally versatile in bonding scenarios, both with simpler elements like oxygen and more complex organic compounds.
Its location in the periodic table categorizes it alongside essential metals such as copper and nickel, with which it shares similar properties, including conductivity and reactivity. The arrangement also alludes to its historical significance: as humans have progressed, the ability to manipulate iron has allowed us to forge tools and buildings that define civilizations. The unique properties associated with transition metals, like iron, underpin many modern technologies and biological systems.
Comparative Analysis with Transition Metals
When we delve into a comparative analysis of iron and its fellow transition metals, several intriguing facets surface. Iron, like nickel and copper, shows notable malleability and ductility. However, itās the production of alloys, especially with carbon, that sets iron apart from others in its group. Steel, forged from iron, represents a perfect blend of strength and ductility, resulting in applications ranging from skyscrapers to kitchen cutlery.
Moreover, the magnetic properties of iron, particularly in its ferromagnetic state, provide a distinct advantage in electronic applications. Many transition metals do exhibit magnetic characteristics, yet ironās ability to be easily magnetized and demagnetized makes it particularly suitable for a wide range of applications in electronics and electromagnetics.
In summary, viewing iron within the context of the periodic table reveals not just its elemental identity but illustrates its critical role in science, engineering, and biology. By understanding how iron compares to its transition metal neighbors, we can better appreciate its multifaceted applications and significance in our world today.
Biological Importance of Iron
Iron plays a vital role in many biochemical processes. Not only does it serve as an essential micronutrient, but it is also integral to the proper functioning of various biological systems. Far from being just another mineral, iron is like the backbone in a biological orchestra, facilitating the harmonious interplay between various physiological processes. Without sufficient iron, the body struggles, creating a cascade of negative effects that highlight its indisputable importance.
Iron's Role in Human Physiology
Iron is a key player in several physiological functions, most notably in the transportation of oxygen throughout the body. It is primarily found in hemoglobin, the protein in red blood cells, where it binds to oxygen and enables its transport from the lungs to various tissues.
Additionally, iron is a component of myoglobin, another protein that helps supply oxygen to muscles. Without adequate iron levels, our muscles can't perform optimally. For instance, a deficiency in iron can lead to anemia, a condition marked by fatigue and weakness. Individuals often report feeling more tired than usual, dragging themselves through daily tasks.
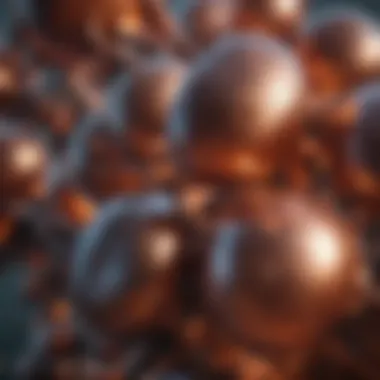
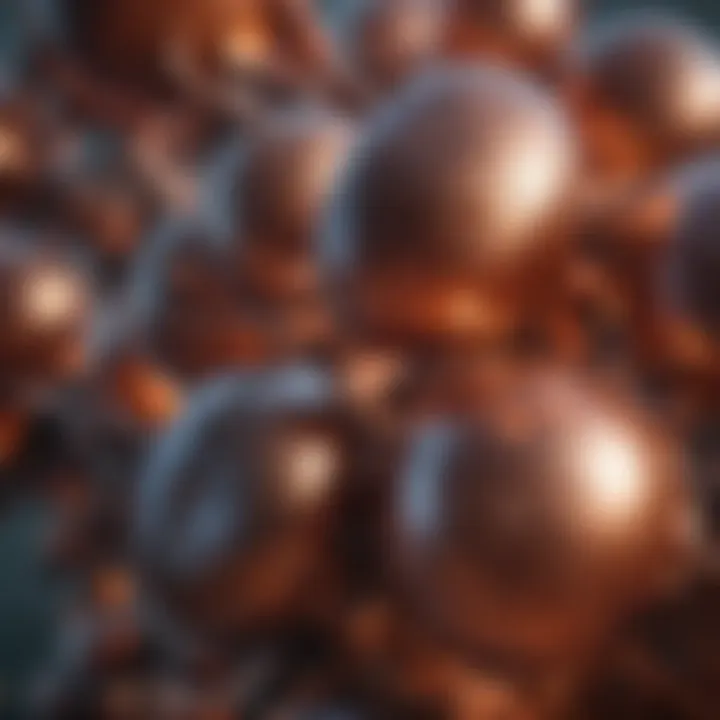
Moreover, iron is involved in several enzymatic reactions. It acts as a catalyst in processes like DNA synthesis, energy production, and cellular respiration. In essence, iron underpins a lot of what we consider basic living functions.
Effects of Iron Deficiency
Iron deficiency has far-reaching implications, often manifesting in various forms of anemia. The symptoms can be quite telling; they may range from fatigue and pale skin to dizziness and shortness of breath during even slight exertion.
When the body lacks iron, its ability to produce hemoglobin falters, which in turn leads to a decreased oxygen supply to tissues. This affects the entire metabolic process, leading to:
- Cognitive issues: There have been studies suggesting that low iron can impact cognitive development in children. It's truly alarming when you think about how something as common as a nutrient deficiency can stunt mental growth.
- Weakened immune response: Iron plays a role in maintaining a healthy immune system. Insufficient levels can lead to a reduced ability to fight off infections, making individuals more susceptible to illnesses.
- Pica: This is a lesser-known but fascinating condition where individuals might crave non-nutritive substances like dirt or chalk. This peculiarity can sometimes be linked to iron deficiency, highlighting the odd ways the body seeks to indicate something is amiss.
Industrial Applications of Iron
Iron is much more than just a common metal; itās the backbone of numerous industries and a pivotal element in the modern world. From towering skyscrapers to everyday appliances, iron's versatility touches almost every aspect of human activity. Its unique combination of properties allows it to be shaped, forged, and employed in myriad ways, making it indispensable in both manufacturing and construction sectors.
Iron in Manufacturing and Construction
In the field of manufacturing, iron finds its applications in creating structural components. Steel, an alloy of iron, is a primary material for building bridges, buildings, and roads due to its strength and durability. Not only does it bear loads effectively, but it also withstands various environmental conditions, making it a long-lasting choice.
Apart from structural uses, iron is also foundational in automotive manufacturing. Automobiles rely heavily on iron-based materials for everything from the chassis to engine components. The automotive industry continues to innovate, leading to modifications like lightweight steel and advanced composite materials to enhance fuel efficiency and performance, highlighting ironās adaptability in a constantly evolving sector. Iron is further employed in appliances; the kitchen ranges from iron skillets to robust refrigerators.
Furthermore, the construction industry utilizes iron in reinforcement bars, known as rebar, to strengthen concrete structures. This combination forms the skeleton of buildings, highways, and bridges, ensuring they can endure the immense pressures they face during their lifespans. Such applications underscore the fact that iron is not merely a building block; it is integral to the very infrastructure of modern society.
Innovations in Iron Processing Techniques
The processing of iron has seen significant advancements over the years. Traditional methods like smelting have modernized, incorporating technologies that enhance efficiency and reduce environmental impact. Techniques such as direct reduced iron (DRI) processing have emerged, allowing for less energy consumption and lower emissions. This method involves extracting iron from ore in an oxygen-free environment, resulting in a purer metal while also minimizing waste.
There's also a push towards using recycled iron, which not only conserves natural resources but also reduces pollution associated with mining and processing raw iron ores. Innovations like electric arc furnaces have drastically transformed how we recycle iron, enabling steel mills to produce steel from scrap in an energy-efficient manner.
Additionally, the rise of 3D printing technology introduces exciting possibilities for iron applications. This method could one day allow for the creation of complex iron structures that are tailored to specific needs, all while significantly reducing material waste and leading to a more sustainable future.
"Iron has evolved from a fundamental element to a critical player in technology and sustainability; the potential for future advancements is boundless."
"Iron has evolved from a fundamental element to a critical player in technology and sustainability; the potential for future advancements is boundless."
Properties of Iron Compounds
Understanding the properties of iron compounds is essential for grasping their role in various chemical applications and biological systems. Iron compounds are ubiquitous and have a wide range of functions, spanning from everyday products to critical components in advanced technologies.
Chemical Properties and Reactions
"Iron's versatility in oxidation states is key to its function in both living organisms and industrial settings."
"Iron's versatility in oxidation states is key to its function in both living organisms and industrial settings."
Moreover, iron compounds readily participate in redox reactions. These reactions are crucial in metabolic processes, such as cellular respiration in humans, where iron-containing proteins like hemoglobin carry oxygen. Additionally, in industries, these reactions are harnessed in processes such as the production of steel from iron ores.
The reactivity of iron compounds also extends to complexation with other ligands. Iron compounds can form coordination complexes with a variety of molecules, allowing for tailored applications in catalysis, where they can either speed up or facilitate chemical reactions. The formation of these complexes often results in distinct color changes, which is exploited in analytical chemistry methods to detect and quantify iron concentrations.
Physical Properties of Iron Alloys
Iron alloys are a cornerstone of modern engineering and manufacturing, offering enhanced physical properties compared to pure iron. The introduction of other elements, such as carbon, chromium, and nickel, results in a wide spectrum of alloys like steel and stainless steel, tailored for specific applications.
Visually, the gleam of stainless steel is a familiar sight, but its resistance to corrosion sets it apart from more traditional iron. This corrosion resistance is due to the formation of a passive layer of chromium oxide that protects the underlying iron from oxidation. Likewise, the addition of carbon to iron creates stainless steel, increasing its tensile strength and flexibility, making it ideal for construction and manufacturing.
The hardness and ductility of iron alloys can be manipulated through heat treatment processes, including annealing and tempering, which alter the microstructure of the metal. This is particularly important in automotive and aerospace industries, where material integrity under stress is critical.
Key physical properties of iron alloys include:
- Strength: Increased tensile strength makes alloys suitable for durable applications.
- Ductility: Allows for shaping and forming into desired structures without breaking.
- Thermal Conductivity: Enables efficient heat transfer in applications like automotive radiators.
- Magnetic Properties: Certain iron alloys retain magnetic properties, which is beneficial in electronics.
In essence, the understanding of both the chemical and physical properties of iron compounds and alloys provides insight into their broad implications in science and industry. Comprehending these aspects leads to better innovation and application of iron-related materials, reinforcing the importance of ongoing research in this area.
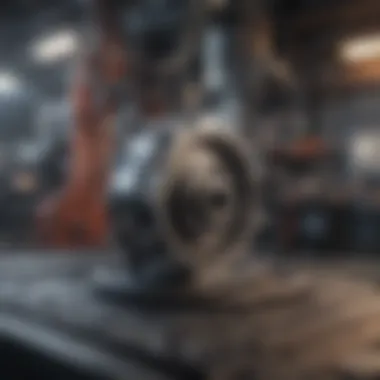
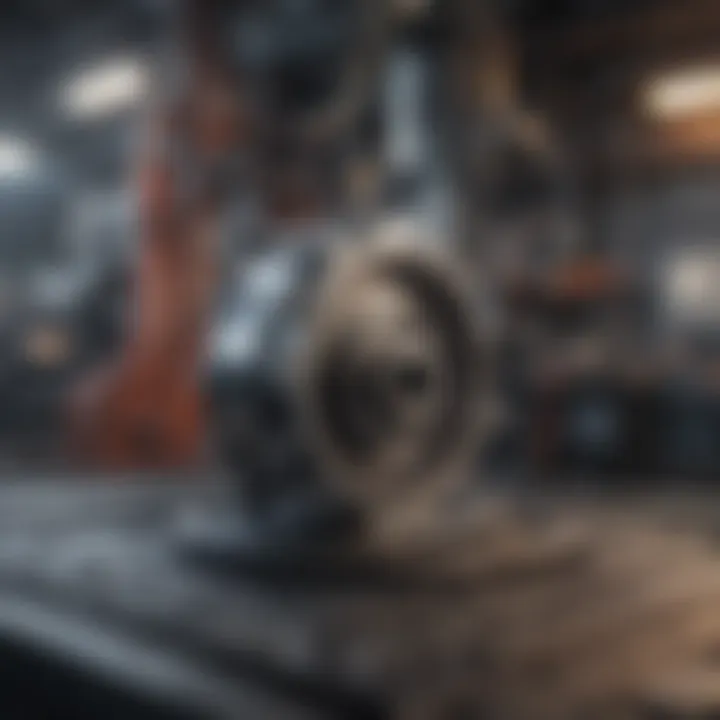
Iron and Environmental Considerations
The significance of iron and its implications on the environment cannot be overstated. As one of the most abundant elements on Earth, iron is not only crucial in various industrial processes but also plays a key role in maintaining ecological balance. This section investigates how iron mining practices can impact sustainability and the surrounding ecosystems. Understanding these dynamics is essential for both industry stakeholders and environmental advocates.
Sustainability and Iron Mining
Iron mining is a necessary component in supplying the metal for numerous applications. However, the environmental cost of extraction can be substantial. The sustainability of iron mining should incorporate practices that minimize ecological disruption. Actions such as:
- Land reclamation: Rehabilitating mined areas can restore habitats for wildlife.
- Efficient resource use: Implementing more resource-efficient practices lowers waste and reduces the environmental footprint.
- Water management: Ensuring that mining operations do not contaminate local water sources is vital for maintaining ecosystem integrity.
Innovative technologies are emerging, aiding in reducing the adverse effects typically associated with iron mining. These include:
- Surface mining techniques that minimize the area disturbed.
- Bioremediation approaches which utilize flora to restore minerals to mined sites.
- Closed-loop systems that recycle water used in processing iron ore.
In many regions, governments are mandating stricter regulations on mining operations to promote sustainability. While profit remains a priority, a shift towards greener mining practices is slowly gaining momentum.
Impact of Iron on Ecosystems
The extraction of iron can lead to significant ecological disturbances. The impact on ecosystems varies depending on several factors including the methods used for mining and the local biodiversity. Here are some of the potential consequences that need serious consideration:
- Habitat destruction: Mining often results in the clearing of forests and vegetation, leading to loss of biodiversity.
- Pollution: Poor mining practices can lead to run-off that contaminates streams and rivers, disrupting aquatic life.
- Soil degradation: The removal of topsoil not only affects flora but can also lead to erosion and loss of arable land.
"The balance of ecosystems can be delicate; reintroducing disturbed areas to their natural state is a complex task that requires thorough planning."
"The balance of ecosystems can be delicate; reintroducing disturbed areas to their natural state is a complex task that requires thorough planning."
On the other hand, iron has vital ecological roles, especially in nutrient cycling. It is an essential micronutrient for many organisms and plays a part in processes like photosynthesis and respiration. In marine environments, for instance, iron acts as a limiting nutrient, controlling primary productivity.
To conclude, while iron mining serves considerable industrial needs, it is imperative that practices evolve towards sustainability. Balancing economic demands with environmental stewardship holds the key to a harmonious relationship between industry and nature.
Recent Advances in Iron Research
Recent strides in iron research have illuminated many facets of this elemental powerhouse, reshaping not just our scientific comprehension but also our practical engagement with iron in various sectors of society. This section will delve into new developments, key breakthroughs, and the far-reaching implications of research surrounding iron, encompassing both academic curiosity and practical applications.
Emerging Trends in Iron Studies
The landscape of iron studies has shifted in recent years due to the integration of interdisciplinary approaches and innovative methodologies. Among these trends, the exploration of nanostructured iron compounds stands out. Researchers are discovering that these materials demonstrate enhanced properties such as increased catalytic activity and greater efficiency in energy applications.
Moreover, the role of iron in biomedicine is gaining traction. Scientists are investigating how iron compounds can be utilized in drug delivery systems, providing targeted therapies for conditions like cancer. The ability to engineer these particles at the nanoscale opens up a range of possibilities for both diagnostic and therapeutic strategies.
Another interesting trend lies in the research around iron-enriched materials for sustainable energy solutions. Iron pyrite, for instance, is being examined for its potential as a photovoltaic material in solar cells. This could pave the way for more economical and sustainable energy sources, reducing dependency on rare materials that are often environmentally detrimental to extract.
"Ironās versatility in applications, from construction to electronics, makes ongoing research indispensable not only for science but also our daily lives."
"Ironās versatility in applications, from construction to electronics, makes ongoing research indispensable not only for science but also our daily lives."
These emerging trends highlight the adaptability of iron compounds and their wide-ranging applications. Researchers are continuously testing new theories, which can result in revolutionary advancements, especially in combating global challenges.
Potential Innovations in Iron Applications
Looking ahead, the innovations stemming from recent iron research are poised to redefine industry standards across a variety of fields. For instance, in the realm of smart materials, iron nanoparticles are expected to play a critical role. These nanoparticles could lead to the development of self-healing materials, which can respond to damage by repairing themselves. The implications here for construction and manufacturing are profound, potentially leading to cost-effective and long-lasting solutions.
In the environmental sector, ironās properties are being further harnessed for pollution remediation. New techniques using iron filings or iron-based catalysts could enhance the efficiency of methods employed to clean contaminants from water sources or to degrade persistent organic pollutants. Such advancements contribute to the sustainability agenda and ensure better compliance with environmental regulations.
Additionally, as cities grow denser, the design of smarter urban environments may see enhanced use of iron-containing materials. Innovations may result in lighter yet stronger constructions, utilizing iron in composite materials to optimize structures while minimizing resource consumption.
The potential of iron in technological applications is also vast, particularly in electronics. Iron-based materials could lead to improvements in magnetic storage, enabling faster data processing and retrieval systems. As we rely more on technology, the need for more efficient materials becomes critical.
In summary, the recent advances in iron research are not just academic but practical, fostering a bridging of gaps between theory and application that could shape our future. As these trends continue to evolve, they hold considerable promise for improving our daily lives and addressing some of Societyās toughest challenges.
Culmination
The final section is where all threads of the discussion converge into a coherent understanding of iron in its myriad forms and functions. The essence of iron goes far beyond simple formulas; it encapsulates a vital part of diverse scientific fields and everyday applications. As we've explored throughout this article, iron demonstrates itself as not only a building block of biological processes but also a cornerstone in industrial advancements.
The summary of key insights is crucial because it distills the vast information into digestible, actionable knowledge. It's where the complex relationships forged in earlier sections become clearer, allowing readers to grasp how iron contributes to everything from anemia treatment to the durability of skyscrapers.
Equally important, the discourse on future directions for iron research illuminates uncharted territories worth exploring. As technology leaps forward and environmental concerns intensify, the quest for sustainable practices related to iron usage will take center stage. This keeps the conversation not just relevant but persistent.
In short, recognizing the significance of iron's various roles encourages us to rethink its applications. Adaptability and innovation within iron research could very well mark the next frontier in both scientific inquiry and practical solutions for society.