Exploring Diffraction Grating in Modern Physics
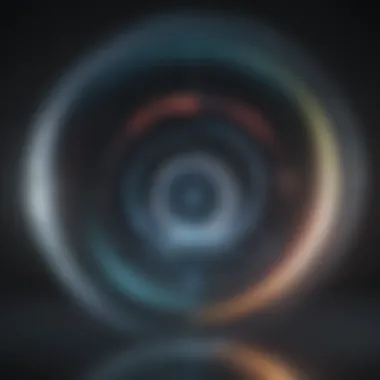
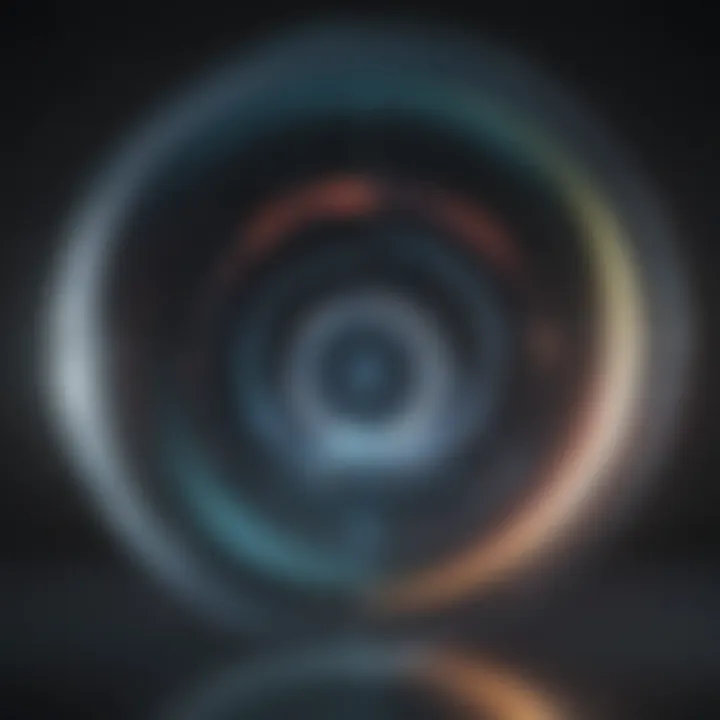
Article Overview
The study of diffraction grating plays an essential role in the modern world of physics and optics. This article aims to peel back the layers on diffraction grating, guiding readers through its fundamental principles and applications. In the following sections, we will explore the type of diffraction gratings, their uses in various fields, and the recent technological advancements that enhance their effectiveness.
Purpose of the Article
The primary goal of this article is to provide a thorough understanding of diffraction grating, catering to a range of audiences from students to seasoned professionals. By merging both theoretical underpinnings with practical insights, this piece aspires to be a comprehensive resource that illustrates how diffraction gratings serve as a bridge between physics and its applications.
Relevance to Multiple Disciplines
Diffraction grating is not just about splitting light. Its implications reach across disciplines:
- Physics: Fundamental experiments elucidate wave properties.
- Materials Science: Gratings are pivotal in characterizing material structures.
- Biology: Techniques like spectroscopy assist in biological research.
- Engineering: Optical devices often depend on gratings for functionality.
Research Background
To truly grasp the significance of diffraction grating, one must first delve into its historical context and foundational concepts.
Historical Context
Diffraction itself is a phenomenon that's been studied for centuries, with early insights laid down by figures like Christiaan Huygens in the 17th century. The modern conception of diffraction grating emerged during the 19th century through the work of pioneers like Joseph von Fraunhofer. His explorations not only identified the principles behind grating but also led to crucial techniques that are still used today.
Key Concepts and Definitions
At its core, diffraction grating harnesses the principles of light wave interference. It typically consists of a series of closely spaced slits or grooves that diffract light into various colors, much like a prism but often with far greater precision. Understanding certain terms is vital:
- Angle of Diffraction: The angle at which light is bent as it passes through the grating.
- Wavelength: The distance between successive peaks of a wave, a key factor in determining how light interacts with the grating.
- Spectroscopy: The study of the interaction between matter and electromagnetic radiation, heavily reliant on techniques involving diffraction gratings.
"Diffraction gratings serve as critical tools for exploring the depths of both the visible and invisible spectrums."
"Diffraction gratings serve as critical tools for exploring the depths of both the visible and invisible spectrums."
As we delve deeper into the article, keep in mind how these concepts weave into applications and advancements that underscore the importance of diffraction grating in today's scientific landscape.
Foundations of Diffraction Grating
Understanding the foundational principles behind diffraction gratings is crucial for those peering into the world of optics and light manipulation. These principles not only lay the groundwork for what follows in the realm of spectroscopy, telecommunications, and sensor technology, but they also illuminate how light behaves when it encounters various surfaces. The importance of these foundations cannot be overstated, as they facilitate more advanced discussions and applications that stem from this core knowledge.
Understanding Diffraction and Interference
To grasp the concept of diffraction, one must first recognize that it’s not just a mere bending of light. It’s a complex interaction that reveals the wave nature of light. When light waves encounter an obstacle or slit that is comparable in size to their wavelength, they spread out instead of simply reflecting off or passing through. This bending or spreading out is called diffraction.
Interference, on the other hand, is the phenomenon that results when two or more light waves meet. It can be constructive, where waves align and amplify each other, or destructive, where they cancel each other out. Both diffraction and interference are vital to understanding how diffraction gratings function. By arraying multiple slits or grooves on a surface, gratings create a structured opportunity for these light waves to interact, resulting in distinct patterns that we can analyze.
"Diffraction teaches us about the underlying behavior of waves, reflecting both their complexities and their beauty."
"Diffraction teaches us about the underlying behavior of waves, reflecting both their complexities and their beauty."
To put this into perspective, consider a classic example: the use of a CD or DVD. When light is shone on the surface of these discs, the microscopic grooves act as a diffraction grating. The resulting spectrum seen when observing the reflected light illustrates the harmony of diffraction and interference in a very tangible way. Understanding this ebb and flow of light is foundational to mastering subsequent topics that will culminate in real-world applications.
Principles of Light Behavior
Delving into the principles of light behavior unveils a tapestry woven from numerous threads of physics. Light travels in straight lines, usually, but that’s just the tip of the iceberg. When it interacts with gratings, it showcases its dual nature: acting both as a particle and a wave.
The core tenets include reflection, refraction, and diffraction itself. Reflection is where light bounces off surfaces, while refraction indicates that light changes direction as it passes through different mediums. However, what truly takes center stage with diffraction grating is how these principles combine. This leads us to consider the equation:
[ d \sin(\theta) = m\lambda ]
where ( d ) is the distance between lines on the grating, ( \theta ) is the angle of diffraction, ( m ) is the order of the spectrum, and ( \lambda ) is the wavelength of light. This relationship highlights how light's behavior is dictated not just by its source, but also by the medium it encounters.
In summary, the foundations of diffraction grating bring together intricate interactions between light waves. Its implications spread across various fields, making it a cornerstone in the understanding of optics. Mastery of these foundational concepts enables researchers and enthusiasts alike to venture confidently into more complex territories of this fascinating subject.
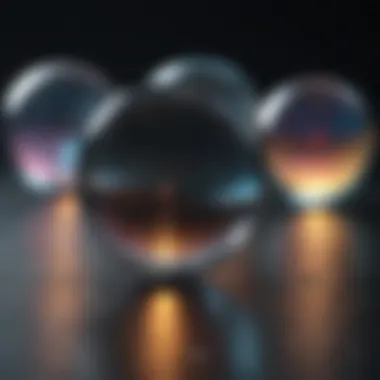
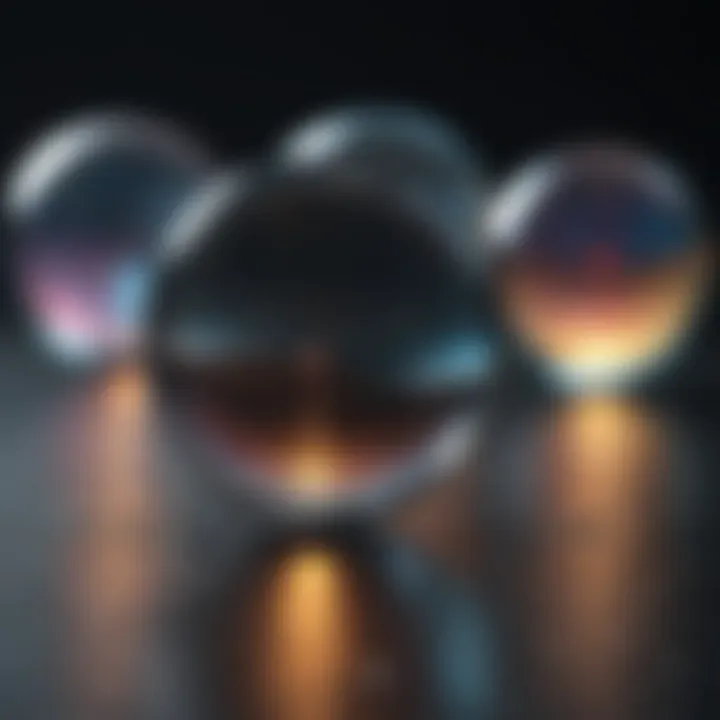
Types of Diffraction Gratings
Diffraction gratings play a pivotal role in optics, serving as essential tools for analyzing light and its properties. Understanding the different types of diffraction gratings is crucial for students, researchers, and professionals alike, as these variations impact light manipulation and application in various fields. Each type of grating offers unique benefits and considerations that make them suitable for specific tasks.
Transmission Gratings
Transmission gratings are designed to allow light to pass through them. These gratings consist of a series of closely spaced lines or grooves, which diffract light into various orders. Their significance lies in their ability to separate light into its constituent wavelengths while maintaining the intensity of the transmitted light. This makes them ideal for applications in spectroscopy, where precise wavelength measurement is necessary.
Some important points about transmission gratings include:
- High Efficiency: They generally exhibit good efficiency, which is crucial when analyzing faint signals.
- Versatility: These gratings can be manufactured in various formats, such as fused silica or glass, which makes them suitable for visible light as well as ultraviolet and near-infrared applications.
- Limitations: However, the resolution may be impacted when high orders are produced, leading to overlapping spectra in some cases.
Reflection Gratings
Reflection gratings operate on a different principle, reflecting incident light rather than transmitting it. The grooves on reflection gratings are oriented to reflect light back, dispersing it into various wavelengths. This type is particularly relevant when high power and brightness are required, such as in laser systems or high-throughput applications.
Reflection gratings have several noteworthy attributes:
- Efficiency at High Orders: They are often more efficient than transmission gratings at higher diffraction orders, making them suitable for applications requiring significant power like laser systems.
- Compactness: These can be more compact since they don’t require space behind them for light to pass through, making them advantageous in limited-space setups.
- Balancing Contrast: While they provide high diffraction efficiency, attention must be paid to the surface quality and groove profile for optimal performance.
Blazed Gratings
Blazed gratings are specially designed with an angled groove structure that enhances the efficiency of light diffracting at specific orders. This uniqueness allows blazed gratings to concentrate the diffracted light more effectively into the desired order, making them a popular choice in precision applications.
Characteristics of blazed gratings include:
- Optimized Performance: By directing more light into a pre-defined order, blazed gratings can maximize signal strength and improve resolution.
- Customizability: They can be tailored for various wavelengths and sensitivity levels, which enhances their applicability.
- Heat Management: Care needs to be taken regarding thermal effects, as high intensity can lead to heating and potential distortion over time.
Understanding these different types of diffraction gratings is paramount for anyone looking to leverage light manipulation effectively in scientific research and real-world applications.
Understanding these different types of diffraction gratings is paramount for anyone looking to leverage light manipulation effectively in scientific research and real-world applications.
Mathematical Description of Diffraction Grating
The mathematical foundation of diffraction grating plays a pivotal role, serving as the backbone for the principles discussed throughout this article. Understanding the mathematical description enables researchers and practitioners alike to navigate the complexities of light manipulation through diffraction. It's not just numbers and formulas; it's a way to predict how light interacts with materials on a microscopic level. This mathematical lens brings clarity to various applications, ranging from spectroscopy to modern telecommunications, significantly enhancing their efficiency and effectiveness.
Grating Equation Derivation
The grating equation is a fundamental tool in understanding diffraction patterns produced by gratings. The equation is expressed as:
[ d \sin(\theta) = m\lambda ]\
where
- d is the distance between adjacent grating lines,
- \theta is the diffraction angle,
- m is the diffraction order (an integer),
- \lambda represents the wavelength of the incident light.
Deriving this equation involves some careful consideration of geometry and principles of wave interference. To begin with, imagine a monochromatic light beam striking a grating at an angle. The light rays diffracted by different slits will interfere with each other, producing a pattern characterized by bright and dark spots known as fringes.
When applying basic trigonometry, you end up establishing the relationship between the grating spacing, the angle of incidence, and the resultant angles of diffraction. Each order of diffraction corresponds to integer values of m, signifying multiple paths the light might take after interacting with the grating.
The ability to accurately derive and manipulate this equation allows for precise control over optical systems used in various fields, ensuring light can be harnessed in remarkable ways. For instance, by adjusting d, one can fine-tune a device for specific wavelengths, aiding in applications like wavelength-selective filters in optical instruments or telecommunications.
Order of Diffraction and Angular Distribution
When discussing the practicality of diffraction gratings, understanding the order of diffraction becomes essential. Each value of m in the grating equation corresponds to a different diffraction order, creating a unique distribution of light.
- First order (m=1): The most intense and typically the brightest order, often utilized in analytical and measurement systems.
- Second order (m=2), and higher orders diffuse the light further and tend to be less intense. They provide additional data points but can complicate the extraction of information if not managed correctly.
The angular distribution of light depends on the wavelength and the grating periodicity, leading to variations in fringe separation and intensity. This spatial arrangement is crucial for determining detection limits in spectroscopy, as wider separations between orders can improve selectivity while narrowing them enhances resolution.
End
In sum, the mathematical description involving the grating equation and the concept of diffraction orders is foundational. It allows for a structured understanding of light behavior as it encounters different types of diffraction gratings. This is critical not only for theoretical insights but for the advancement of practical applications in various scientific fields. As researchers advance in their understanding, the multitude of uses for diffraction gratings continues to expand, underscoring their significance in contemporary physics.
Fabrication Techniques for Diffraction Gratings
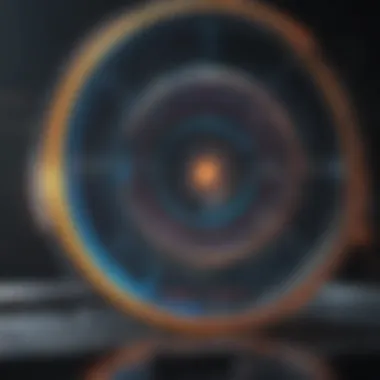
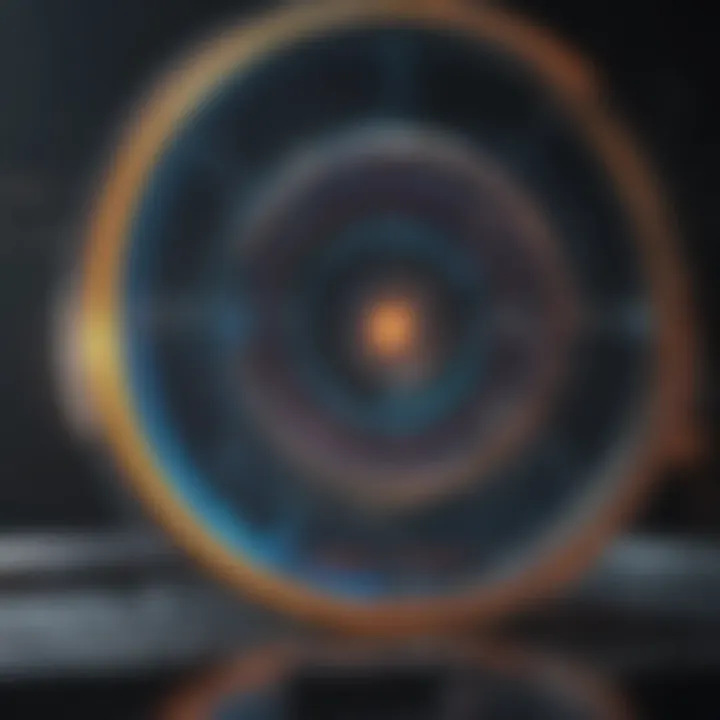
The production of diffraction gratings is not just a technical endeavor; it lies at the very heart of how these optical devices function and contribute to various scientific fields. An advanced understanding of fabrication techniques opens doors to enhanced performance and the development of specialized applications in spectroscopy, telecommunications, and bio-sensing technologies. By diving into these methods, researchers can fine-tune the properties of gratings, ensuring they meet the rigorous demands of contemporary physics.
Photoetching Methods
Photoetching is a widely used technique for creating diffraction gratings that capitalizes on photosensitive materials. In this process, a photoresist layer is applied onto a substrate, typically glass or silicon. After exposure to light through a mask, the photoresist is developed, revealing the patterned surface on which the grating grooves will be etched.
- Precision Control: This method allows for fine control over groove spacing and depth. The resolution achieved can be exceptional, often reaching sub-micrometer accuracy.
- Cost-Effectiveness: Photolithography can be scaled for mass production, making it a cost-effective choice in various applications.
- Versatility: It can be adapted for different materials and geometries, providing flexibility in design.
However, some considerations include material limitations as not all substrates can withstand the etching process. Furthermore, careful handling during the photolithography process is crucial as any contamination can lead to defects in the final product.
Electron Beam Lithography
Electron Beam Lithography (EBL) stands out for its sheer precision and capability to produce ultra-fine features at nanometer scales. Unlike traditional photoetching, which relies on optical masks, EBL utilizes a focused beam of electrons to directly write patterns onto a semiconductor substrate.
- High Resolution: The capability to produce gratings with extraordinarily fine grooves allows researchers to explore uncharted territories in optics. This is particularly beneficial for applications in the realm of photonics.
- Customizability: Because it is maskless, every design can be uniquely tailored without incurring the high costs associated with custom masks.
Yet, challenges include a slower throughput compared to photoetching methods—making it less suitable for large-scale production. The cost of EBL equipment and maintenance can also be prohibitive for smaller labs.
Nanofabrication Approaches
Nanofabrication techniques are becoming increasingly relevant as demand for miniature devices in optics rises. This umbrella term encompasses various methods, including top-down approaches like colloidal lithography, and bottom-up techniques like self-assembly.
- Scalability: Nanofabrication allows large arrays of gratings to be created simultaneously, which is advantageous in enhancing performance metrics in bulk optics.
- Complex Structures: Researchers can engineer complex nanostructures that enhance the efficiency and functionality of diffraction gratings beyond what was previously possible.
Yet, practitioners face hurdles like integration with existing technologies, and sometimes the materials used can have unpredictable optical properties at nano scales.
"Through innovative fabrication techniques, diffraction gratings are continually evolving, playing a fundamental role in revolutionizing optical technologies."
"Through innovative fabrication techniques, diffraction gratings are continually evolving, playing a fundamental role in revolutionizing optical technologies."
In summary, the techniques for fabricating diffraction gratings demonstrate a blend of artistry and science, where careful planning and precision intersect with the ingenuity of modern technology. Each method brings its unique set of advantages and complications. Navigating these aspects equips researchers and professionals to leverage diffraction gratings in ways that push the envelope of current scientific understanding.
Applications of Diffraction Grating
Diffraction gratings play an integral role in numerous technological and experimental realms, particularly within optics. Their versatility and precision in manipulating light make them an essential tool in various applications. The ability to disperse light into its constituent wavelengths allows scientists and engineers to explore a multitude of phenomena, leading to advancements across disciplines. Let’s delve into some specific areas where these gratings shine.
Spectroscopy in Chemical Analysis
At the heart of spectroscopic techniques, diffraction gratings serve a vital function. They enable the separation of light into a spectrum, granting researchers a visual representation of wavelengths. This capability is crucial in chemical analysis, as different substances absorb and emit light at characteristic wavelengths.
- Identification of Compounds: By analyzing the spectrum produced by a sample, scientists can determine its composition. Each chemical compound has a unique spectral fingerprint, making it possible to identify substances accurately.
- Quantitative Measurements: Spectroscopy also allows for quantitative analysis. By measuring the intensity of light at specific wavelengths, researchers can ascertain the concentration of substances in a mixture, which is essential in fields ranging from environmental monitoring to pharmaceuticals.
- Real-World Applications: In practice, this method is utilized in diverse settings, such as monitoring pollutants in air and water, analyzing food safety, and even in forensic science to identify unknown substances. The precision of the technique enhances reliability in results, making it a cornerstone in chemical analysis.
Optical Sensing Technologies
Another noteworthy application of diffraction gratings is in optical sensing technologies. Here, the gratings facilitate the detection of changes in light properties that correspond to specific environmental or biological factors.
- Sensor Development: Gratings can be incorporated into sensor designs that respond to changes in refractive index, temperature, or concentration of chemical species. This adaptability allows for the creation of highly sensitive sensors.
- Real-Time Monitoring: These sensors are instrumental in real-time monitoring applications. For instance, in healthcare, they can detect changes in biomolecules linked to diseases. In industries, they can monitor environmental changes that affect product quality or safety.
"The development of advanced sensors, including those using diffraction gratings, is paving the way for more efficient monitoring solutions across sectors, tackling challenges from pollution to health diagnostics.”
"The development of advanced sensors, including those using diffraction gratings, is paving the way for more efficient monitoring solutions across sectors, tackling challenges from pollution to health diagnostics.”
- Versatility at Play: The flexibility of optical sensing technologies highlights the importance of diffraction gratings in both research and commercial sectors. Their precision ensures that insights gleaned from monitoring applications are reliable, which is critical for informed decision-making.
Laser Systems and Telecommunications
The interaction of diffraction gratings with laser systems and fiber optics is another area that cannot be overlooked. Gratings modulate and manage light in ways that can significantly enhance the performance of communication systems.
- Wavelength Division Multiplexing (WDM): In telecommunications, diffraction gratings are crucial for WDM, a technology that allows multiple signals to be transmitted over a single fiber optic cable at different wavelengths. This process maximizes the efficiency and bandwidth of communication networks.
- Laser Applications: Similarly, in laser technology, gratings are employed to stabilize laser frequencies and improve beam quality. These applications are vital in both scientific research and commercial settings, such as laser cutting or medical procedures.
- Future Prospects: As optical communication continues to expand, the demand for more sophisticated grating technologies will likely increase. Innovations in this field can lead to faster data transmissions and more robust networks, essential in today's fast-paced digital environment.
In summary, diffraction gratings are not mere tools of academic study; they have a tangible impact across various fields, from chemical analysis to sensing technologies and telecommunications. Their adaptability, precision, and efficiency underscore their significance in advancing modern physics and its applications.
Recent Advancements in Diffraction Grating Technologies
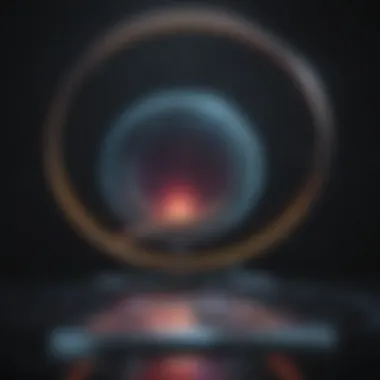
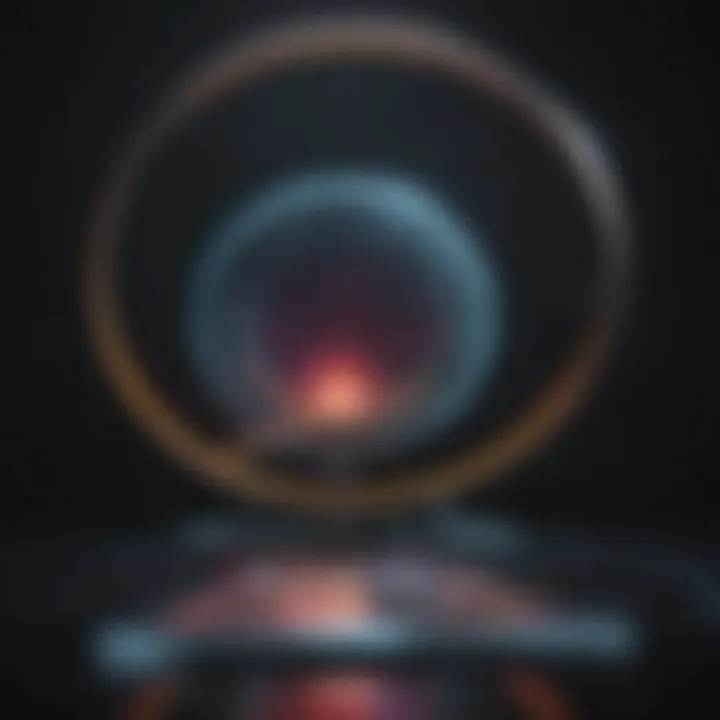
The landscape of optics has seen a transformative shift with the recent advancements in diffraction grating technologies. These developments are not just incremental improvements; they represent significant jumps that enhance functionality and application in various fields. As the demand for precision increases in industries such as telecommunications, spectroscopy, and sensing technologies, understanding the innovations in this area becomes pivotal.
Developments in Plasmonic Gratings
Plasmonic gratings are a cutting-edge technology that leverages the interaction between light and localized surface plasmons in metallic structures. These gratings can manipulate light at dimensions smaller than the wavelength of light, leading to fascinating applications in sensors and imaging systems. The real beauty of plasmonic gratings lies in their ability to amplify optical signals. When light hits these structures, it can produce significantly enhanced signals, allowing for the detection of lower concentrations of substances, which is a game changer for chemical analysis and biological sensing.
One key example is the use of plasmonic gratings in biosensors. These devices can detect biomolecules with high sensitivity, enabling rapid diagnostics in medical fields. The recent advancements in fabricating such gratings have allowed for greater control over their optical properties, making them highly precise. The tailoring of the grating's dimensions affects the wavelength of resonance, giving researchers a tool to fine-tune their applications.
"Plasmonic gratings are not just an enhancement; they're a revolution in how we approach light manipulation at a fundamental level."
"Plasmonic gratings are not just an enhancement; they're a revolution in how we approach light manipulation at a fundamental level."
Incorporating plasmonic mechanisms has also opened avenues for novel devices, including optical switches and photonic circuits. As the integration of optics in electronic systems grows, these advancements establish a crucial synergy between the two fields.
Nanostructured Gratings for Enhanced Performance
The development of nanostructured gratings is another exciting chapter in the evolution of diffraction grating technologies. These gratings boast features that enable them to manage light properties in remarkable ways. Imagine layers of hybrid materials arranged on a nanometer scale, designed to enhance light absorption, reflection, and diffraction.
Nanostructured gratings are instrumental in many applications, including solar energy collection and LED technology. By optimizing the surface features, researchers manage to push the boundaries of efficiency. For instance, in solar cells, these gratings can direct more sunlight into the semiconductor, improving overall energy capture. This capability fosters advancements in sustainable energy solutions, paving the way for greener technologies.
Another impressive application of this technology is in the field of imaging systems. Nanostructured gratings can enhance the resolution of imaging devices. By controlling light at the nanoscale, these gratings enable better detail capture in optical microscopies, leading to breakthroughs in biological research and material science.
In summary, the innovations in plasmonic and nanostructured gratings offer vast possibilities. They hold the potential to revamp existing technologies while inspiring new applications that could solve complex problems in scientific and industrial realms. Understanding these advancements is not just beneficial for students and professionals; it’s essential to grasp the future trajectory of optical technologies.
Challenges in Diffraction Grating Research
In the realm of optics, while diffraction gratings play a central role in numerous applications, they are not without their challenges. Understanding these difficulties is not just important for researchers but also for practitioners who rely on the effectiveness of these optical devices in their work. The complexities involved in the fabrication and usage of diffraction gratings can influence their performance, durability, and overall applicability in various fields. Addressing these challenges is essential for advancing current technologies and optimizing future innovations.
Material Limitations
One of the prominent hurdles in the field of diffraction gratings is related to material limitations. The choice of materials directly affects the grating's efficiency and spectral range. For instance, traditional materials such as glass and certain plastics may exhibit satisfactory performance in visible light applications but struggle when tasked with dealing with ultraviolet or infrared wavelengths. The refractive index, absorption coefficients, and mechanical properties of these materials can restrict the operational scope of grating devices.
Additionally, while creating gratings for applications involving high-energy photons, like X-rays, one encounters materials like silicon or specialized alloys that can withstand such high-energy impacts, but these options can be significantly costlier. Beyond costs, some materials may also present challenges during the fabrication process, including etching precision or thermal stability during exposure to light. A well-thought-out selection of materials that balances performance characteristics with manufacturing feasibility is critical for successful implementation.
Environmental Sensitivities
Another significant challenge lies in the environmental sensitivities of diffraction gratings. These devices can be influenced by factors such as temperature fluctuations, humidity levels, and exposure to various chemicals. For instance, some materials may suffer from distortion or degradation when exposed to high humidity, which can subsequently lead to performance issues in applications that require consistent results.
Furthermore, temperature variations can induce thermal expansion in the grating material, causing displacement of the aligned features. This can shift the diffraction pattern, thus altering the light paths that are essential for precise measurements in spectroscopic methods. Such sensitivity to environmental changes means that special care and measurement conditions must be maintained during experiments or applications, complicating operational logistics.
Understanding and addressing these material and environmental challenges is imperative in order to ensure the optimal performance of diffraction gratings.
Understanding and addressing these material and environmental challenges is imperative in order to ensure the optimal performance of diffraction gratings.
As research progresses and novel materials and fabrication techniques emerge, there's hope for overcoming these issues. Striking a balance between innovation and practical reliability will be the key to unlocking new potential applications for diffraction gratings.
The Future of Diffraction Grating
As the field of optics continues to evolve, the dialogue surrounding the future of diffraction grating cannot be ignored. This essential tool has been a stalwart in both research and practical applications and will be crucial in the ongoing quest for precision in optical technologies. Its ongoing development promises enhanced capabilities across multiple domains, and understanding this trajectory is vital for students, researchers, and professionals alike.
One significant consideration is the persistent demand for more effective spectroscopic methods. With applications ranging from environmental monitoring to telecommunications, diffraction gratings must increasingly meet stringent criteria for sensitivity and accuracy. The future landscape will likely see gratings that can operate effectively across a wider spectrum of light, making it easier to analyze complex chemical compounds, detect pollutants, and facilitate real-time data processing in communication networks.
Interdisciplinary Applications
The future of diffraction grating isn't confined merely to traditional optics; it branches into various interdiciplinary fields, broadening its relevance considerably. Here are some areas where it can make a lasting impact:
- Biomedical Imaging: The integration of diffraction gratings in imaging systems could significantly enhance the resolution and specificity of optical imaging techniques used in medical diagnostics.
- Environmental Science: Advanced diffraction gratings stand to improve the detection of trace pollutants and toxins in natural water sources, contributing to better environmental monitoring.
- Information Technology: With the rise of quantum computing and photonics-based technologies, diffraction gratings are likely to play a critical role in managing quantum states and light fields, which can allow for faster data processing and communication systems.
"The advancements in diffraction grating technology will surely bridge the gap between several scientific disciplines, enabling groundbreaking discoveries and enhancements in everyday applications."
"The advancements in diffraction grating technology will surely bridge the gap between several scientific disciplines, enabling groundbreaking discoveries and enhancements in everyday applications."
Potential Innovations and Trends
Looking ahead, various innovations and trends are emerging that hint at a transformative future for diffraction gratings:
- Integration with Nanotechnology: By incorporating nanostructured materials, new designs could lead to gratings that provide superior performance in light manipulation. Innovations in this area promise increased sensitivity and efficiency, which can revolutionize applications in various fields.
- Smart Gratings: The notion of 'smart materials' is taking root, and diffraction gratings could evolve to incorporate adaptive characteristics, adjusting their properties based on environmental conditions. This could lead to dynamic optical devices that respond in real-time to stimuli.
- Sustainability Considerations: As the global focus shifts toward sustainability, future developments may also address the ecological footprint of manufacturing diffraction gratings. Techniques that utilize more sustainable materials or processes will likely gain traction, ensuring that advancements do not come at the expense of our environment.
The exploration of these applications and innovations heralds an exciting time for diffraction gratings. As interdisciplinary collaboration continues to flourish, we can anticipate a bright future where this technology will not only enhance our understanding of the physical world but also contribute to solving some of society's pressing challenges.