Exploring Genetic Engineering and Recombinant DNA
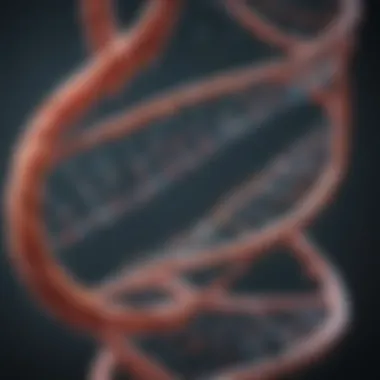
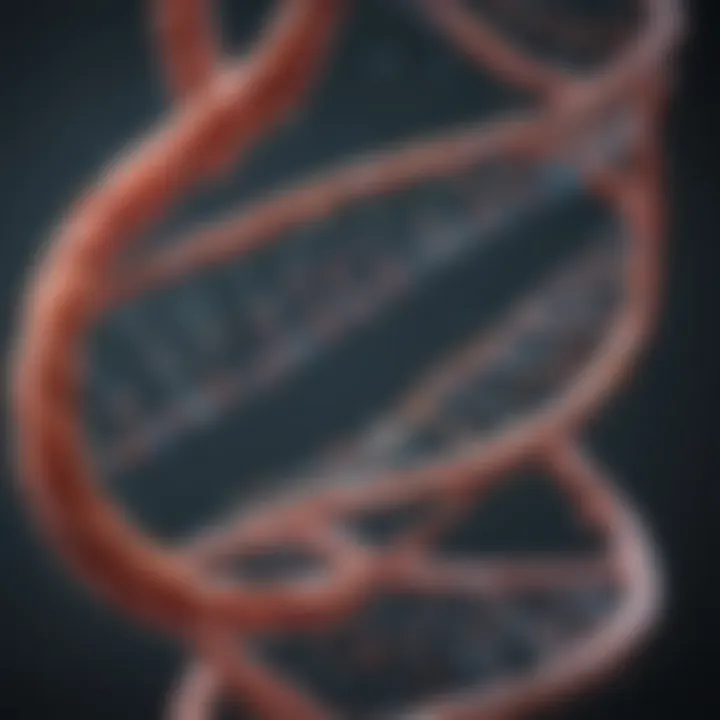
Article Overview
Purpose of the Article
The purpose of this article is to provide a thorough examination of genetic engineering and recombinant DNA technology. These technologies have revolutionized not only the field of biology but also numerous sectors such as medicine, agriculture, and environmental science. By analyzing the methodologies, applications, and the ethical implications involved, this article seeks to enhance understanding of the complex landscape influenced by genetic manipulation. It is essential for students, researchers, educators, and professionals to grasp both the potential and the challenges associated with these advancements.
Relevance to Multiple Disciplines
Genetic engineering and recombinant DNA technology are pertinent to various disciplines. In medicine, these technologies enable groundbreaking therapies, including gene therapy for genetic disorders. Agriculture benefits from these advancements through the development of genetically modified organisms (GMOs), which can enhance crop yield and resilience against disease. Environmental science employs genetic tools to manage ecosystems and address conservation challenges. The interdisciplinary nature of this field highlights its significance across sectors.
Research Background
Historical Context
The history of genetic engineering traces back to the early 20th century with the discovery of DNA's structure by James Watson and Francis Crick in 1953. However, the practical application of genetic modification began in earnest in the 1970s when scientists developed recombinant DNA technology. This technology allows for the insertion of DNA from one organism into another, fundamentally altering biological functions. The first successful transformation of a bacterium using recombinant DNA occurred in 1973, marking the inception of a new era in biotechnology.
Key Concepts and Definitions
Understanding the terminology is crucial for delving into genetic engineering. Here are some key concepts:
- DNA (Deoxyribonucleic Acid): The hereditary material in humans and most organisms, encoded with genetic information.
- Recombinant DNA (rDNA): DNA that has been artificially formed by combining constituents from different organisms.
- Genetic Engineering: The direct manipulation of an organism's genes using biotechnology.
- CRISPR-Cas9: A powerful tool for editing genomes, allowing researchers to easily alter DNA sequences and modify gene function.
Genetic engineering is not merely a scientific endeavor; it raises profound ethical and social questions.
Genetic engineering is not merely a scientific endeavor; it raises profound ethical and social questions.
In summary, the integration of genetic engineering in various fields illustrates its far-reaching implications. An in-depth understanding of its principles and applications is essential for engaging with contemporary scientific debates.
Prolusion to Genetic Engineering
Genetic engineering stands as a cornerstone in the realm of biotechnology, enabling the manipulation of an organism's genetic material to achieve desirable traits. This ability has transformative implications for various fields, such as medicine, agriculture, and environmental science. Understanding genetic engineering is essential, as it opens up discussions on innovation, ethical considerations, and future impacts.
Definition and Scope
Genetic engineering refers to the direct manipulation of an organism's genes using biotechnology. It encompasses a wide range of techniques, such as DNA cloning, genetic modification, and genome editing. The purpose of these techniques varies; it can be aimed at improving crop yield, developing gene therapies for diseases, or even creating organisms with entirely new functions.
The scope of genetic engineering stretches across multiple disciplines. In agriculture, it allows the creation of genetically modified organisms (GMOs), which can withstand pests and environmental stressors. In medicine, genetic engineering contributes to groundbreaking therapies that target diseases at the molecular level. The potential applications seem limitless, suggesting that the tools and techniques of genetic engineering will grow increasingly important in our society.
Historical Background
The journey of genetic engineering began in the early 20th century with the discovery of DNA as the carrier of genetic information. The foundational work by scientists such as Gregor Mendel laid the groundwork for genetics. However, it wasn’t until the 1970s that the necessary techniques came into focus.
In 1973, a significant breakthrough occurred with the advent of recombinant DNA technology. The pioneering work of Herbert Boyer and Stanley Cohen enabled the introduction of foreign DNA into bacterial cells. This was the first step towards a new era of genetic manipulation, allowing for advancements in numerous biotechnological applications.
By the 1980s, the first genetically modified organisms were created, leading to public discussions and eventual regulatory frameworks surrounding the ethical and safety implications of this technology. The development of tools like CRISPR-Cas9 in recent years symbolizes the rapid evolution of genetic engineering, making it easier, faster, and more precise than ever before.
Understanding the evolution of genetic engineering helps to contextualize its current implications and the pressing need for dialogue about its ethical constraints and societal impact.
Principles of Recombinant DNA Technology
Recombinant DNA technology is a cornerstone of genetic engineering, enabling scientists to manipulate genetic material in precise ways. This section explores the essential principles that underpin this technology, shedding light on its methodologies and implications. The integration of DNA from different sources allows researchers to create organisms with desirable traits, which can be applied across various sectors such as medicine, agriculture, and environmental science.
DNA Cloning
DNA cloning involves creating copies of specific DNA fragments. This process is crucial for studying genes and their functions. It starts with the isolation of the desired DNA segment, usually through digestion with restriction enzymes. The fragment is then inserted into a vector, which carries the DNA into host cells. Common vectors include plasmids and bacteriophages. This step is essential for amplifying the DNA of interest, making large quantities available for further research or application.
Some notable benefits of DNA cloning include:
- Targeted Research: Scientists can focus on particular genes to understand their roles and interactions.
- Production of Recombinant Products: It enables the production of proteins, hormones, and vaccines.
- Gene Therapy: Cloning is fundamental to developing therapies that can correct genetic disorders.
Gene Editing Techniques
Gene editing techniques represent a revolutionary advancement in biotechnology. They allow for precise alterations to DNA, altering the genetic makeup of organisms effectively. Three prominent methods in this field are CRISPR-Cas9, TALENs, and Zinc Finger Nucleases. Each technique offers unique features, advantages, and limitations, shaping the landscape of genetic research and application.
CRISPR-Cas9
CRISPR-Cas9 is perhaps the most notable technique due to its simplicity and efficiency. It utilizes a guide RNA to direct the Cas9 enzyme to the target DNA sequence, making a double-strand break. The cell's natural repair mechanisms then take over, allowing for the insertion or deletion of specific genes. This mechanism makes CRISPR-Cas9 a popular choice in genetic studies.
- Key Characteristic: Its ease of use and programmability.
- Advantages: The ability to edit genomes in a variety of organisms, including plants and animals, with high precision and minimal side effects.
- Disadvantages: Potential off-target effects, where unintended parts of the genome may be altered.
TALENs
TALENs, or Transcription Activator-Like Effector Nucleases, are another powerful gene-editing tool. They work by fusing a DNA-binding domain with a nuclease to create targeted breaks in DNA. Unlike CRISPR, TALENs require the design of protein domains for each target sequence, making them more complex but equally effective.
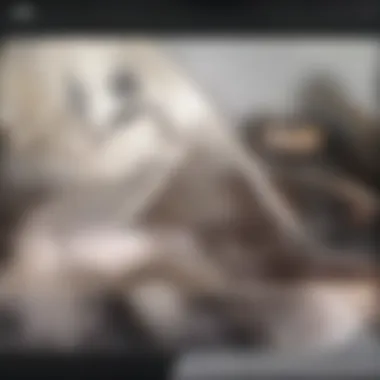
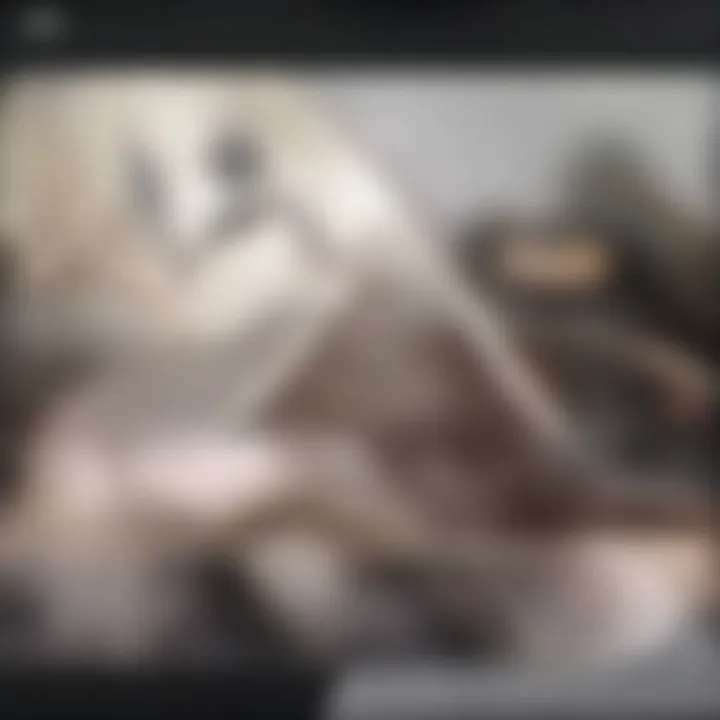
- Key Characteristic: Highly customizable for specific target sequences.
- Advantages: Lower off-target effects compared to other techniques and can target a wider variety of sequences.
- Disadvantages: Complexity in design and production can be a limitation for broader application.
Zinc Finger Nucleases
Zinc Finger Nucleases (ZFNs) are one of the earliest gene-editing technologies. ZFNs combine DNA-binding domains and nucleases to target specific DNA sequences. Each ZFN is engineered to bind to a specific sequence, which allows for precise editing.
- Key Characteristic: Ability to target multiple sequences simultaneously.
- Advantages: High specificity and effectiveness for genomic modifications.
- Disadvantages: Development of ZFNs can be labor-intensive and may result in some off-target effects.
Core Methodologies in Genetic Engineering
Core methodologies in genetic engineering are essential in understanding how genes are manipulated for various applications. These methods provide the foundational tools needed for researchers to conduct experiments and develop innovations. They not only enhance our understanding of genetic material but also enable the practical application of this knowledge in fields like medicine, agriculture, and environmental science. Key methodologies include restriction enzymes, ligases, and vector development systems, each contributing uniquely to the manipulation of genetic material.
Restriction Enzymes and Ligases
Restriction enzymes play a crucial role in genetic engineering. These enzymes act as molecular scissors, cutting DNA at specific sequences. This property allows researchers to isolate particular genes of interest. Once a gene is cut out, ligases come into play. Ligases are enzymes that facilitate the joining of DNA fragments together. This process is vital for creating recombinant DNA, where a gene from one organism is inserted into the DNA of another. The combination of restriction enzymes and ligases allows scientists to manipulate genetic information with precision.
Vector Development
Vector development is another core methodology in genetic engineering. Vectors are tools that transport foreign genetic material into a host cell. They are vital for the successful delivery of genes in various applications. There are several types of vectors, and each has unique characteristics that make it suitable for different scenarios.
Plasmids
Plasmids are small, circular DNA molecules that can replicate independently of chromosomal DNA. Their importance lies in their ability to carry and express foreign genes in host organisms. Plasmids are popular due to their ease of manipulation and the ability to introduce multiple genes simultaneously. A key characteristic of plasmids is their origin of replication, which enables them to be maintained in the host cell.
One significant advantage of plasmids is their versatility. They can be used in various organisms, from bacteria to plants. However, they may have limitations when it comes to carrying very large genes or multiple genes that are too complex, potentially leading to decreased efficiency in expressing those genes.
Bacteriophages
Bacteriophages, or phages, are viruses that infect bacteria. They are increasingly being explored as vectors in genetic engineering. Their ability to deliver genetic material specifically into bacterial cells makes them a powerful tool. A key characteristic of bacteriophages is their specificity to host cells, allowing for targeted delivery.
Bacteriophages show promise in gene therapy approaches, as they can potentially introduce genes into bacterial strains used in pharmaceuticals. However, their use can be limited by the host range and potential immune responses from the host organism.
Viral Vectors
Viral vectors are derived from viruses that have been modified to carry therapeutic genes. These vectors are particularly beneficial in gene therapy for their efficiency in transferring genetic material into host cells. A notable feature of viral vectors is their ability to integrate the delivered genes into the host genome, allowing for long-term expression.
The advantages of viral vectors include their high transduction efficiency and the capacity to infect various cell types. However, concerns around potential immune responses and the stability of the inserted material must be addressed. Safety profiles are crucial to ensure that these vectors do not interfere with normal cellular functions or elicit adverse reactions.
Proper selection of methodology is essential for achieving successful genetic engineering outcomes. Each method carries its own set of advantages and limitations that must be considered based on the application at hand.
Proper selection of methodology is essential for achieving successful genetic engineering outcomes. Each method carries its own set of advantages and limitations that must be considered based on the application at hand.
In summary, understanding the core methodologies in genetic engineering provides the groundwork for advancing this field. From restriction enzymes to various vector systems, each methodology plays a critical role in the manipulation, isolation, and application of genetic material.
Applications in Medicine
The intersection of genetic engineering and medicine signifies a monumental leap in biotechnology. Such advancements foster innovative solutions addressing previously insurmountable medical challenges. The focus on gene therapy and vaccine development illustrates the breadth of possibilities arising from recombinant DNA technology. Their impact enhances patient care and offers hope for conditions previously deemed untreatable.
Gene Therapy
Gene therapy represents a transformative approach in treating genetic disorders. The basic principle is to correct defective genes responsible for disease development. By delivering a healthy copy of the gene or editing the defective one, this technique offers a potential remedy.
Gene therapy has successfully treated conditions such as spinal muscular atrophy and certain inherited retinal diseases. The approach is versatile; it can target a range of genetic mutations. However, challenges exist. Immune responses to therapy and the long-term effectiveness require careful scrutiny. Regulatory guidelines also dictate the pace of clinical adoption. The ongoing research into vector systems, such as viral vectors, progresses in improving delivery efficiency and minimizing adverse effects.
Vaccine Development
Vaccine development has also entered a new era thanks to advancements in genetic engineering. Modern vaccines, especially those leveraging recombinant techniques, demonstrate a nuanced understanding of immune response activation. This subsection delves into two prominent types: recombinant vaccines and mRNA vaccines.
Recombinant Vaccines
Recombinant vaccines utilize genetically engineered organisms to produce antigens. These antigens stimulate an immune response without causing actual disease. A notable example is the hepatitis B vaccine, which uses recombinant DNA technology to produce the Hepatitis B surface antigen.
The key characteristic of recombinant vaccines is their safety profile. They are well tolerated and reduce the risk of infection associated with live-attenuated vaccines. Their adaptability in production is a significant advantage, especially during pandemics. However, one distinct feature of recombinant vaccines is their lower immunogenicity, requiring adjuvants to enhance the immune response. This aspect can increase costs and complicate dosing schedules.
mRNA Vaccines
mRNA vaccines mark another paradigm shift in vaccine technology. By encoding the viral protein, these vaccines instruct cells to produce a harmless piece of the virus, prompting an immune response. The swift development and deployment of COVID-19 mRNA vaccines, such as those from Pfizer-BioNTech and Moderna, highlight their potential.
The main advantage of mRNA vaccines lies in their rapid design and production capabilities. They can be adapted quickly, making them a suitable choice in outbreak situations. However, storage and handling require ultra-cold conditions, raising logistical challenges. Moreover, while effective, questions remain about their long-term immunity and possible side effects.
The integration of genetic engineering into medicine offers revolutionary solutions but demands ethical considerations and rigorous regulatory oversight.
The integration of genetic engineering into medicine offers revolutionary solutions but demands ethical considerations and rigorous regulatory oversight.
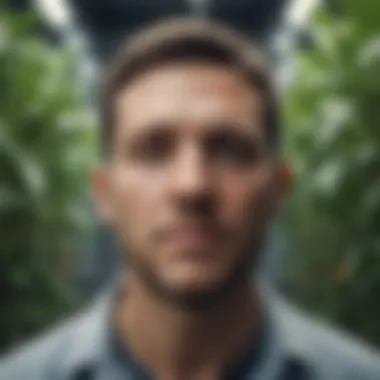
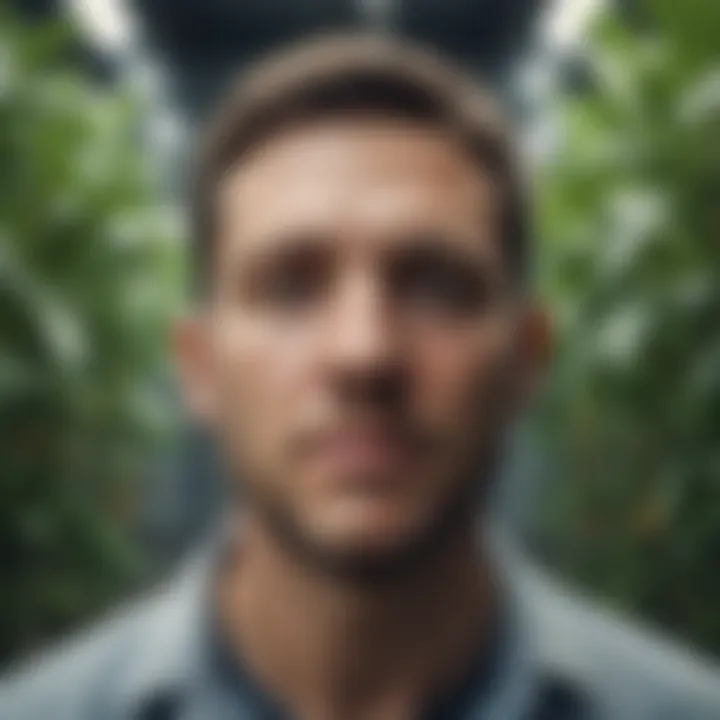
Genetic Engineering in Agriculture
Genetic engineering plays a significant role in agriculture, aiming to improve crop yield, enhance resistance to pests, and promote sustainability. The ability to manipulate the genetic material of plants and animals enables scientists to create organisms with desirable traits. This approach has the potential to address some of the most pressing global challenges, including food insecurity and climate change. The use of genetically modified organisms (GMOs) is at the forefront of this movement, offering a variety of benefits while also presenting certain ethical and environmental considerations.
Genetically Modified Organisms (GMOs)
Crops
Genetically modified crops have transformed agricultural practices. One of the primary advantages of these crops is their enhanced resistance to pests and diseases. For example, Bt cotton and Bt corn incorporate genes from the bacterium Bacillus thuringiensis, which produces a toxin harmful to specific insects. This leads to reduced reliance on chemical pesticides, which is beneficial for both the environment and farmers’ economies.
The key characteristic of GM crops is their ability to withstand herbicides and extreme environmental conditions. Crops like Roundup Ready soybeans allow farmers to control weeds more effectively while minimizing crop damage. These traits contribute to higher yields and more efficient land use.
However, the widespread adoption of these crops has raised concerns regarding biodiversity and long-term sustainability. Some studies indicate that GM crops may lead to monoculture practices, which can diminish the resilience of ecosystems. Its important for farmers and consumers to weigh these pros and cons carefully.
Livestock
In the realm of livestock, genetic engineering has led to advancements that enhance growth rates and disease resistance. For instance, the AquaBounty salmon is engineered to grow faster than its non-modified counterparts, reaching market size in less time. This efficiency can help meet increasing protein demands as the global population grows.
The key characteristic of genetically modified livestock is their potential for reduced resource consumption. By improving growth efficiency, such animals require less feed and water. This can lead to a more sustainable agriculture system, particularly in areas facing resource constraints.
Nonetheless, the use of GM livestock is not without challenges. Concerns regarding animal welfare, genetic diversity, and potential long-term effects on ecosystems are significant. The public discourse surrounding these issues is critical for shaping the future path of genetic engineering in animal husbandry.
Biotechnology for Sustainability
Biotechnology plays a pivotal role in promoting sustainable agricultural practices. By using genetic engineering, scientists can develop crops that require fewer inputs such as water and fertilizers. This minimizes the environmental impact while maximizing productivity.
For example, drought-resistant crops can maintain yields during periods of insufficient rainfall. This not only helps in combating food insecurity but also preserves water resources. Furthermore, nutrient-enhanced crops can improve food quality and health benefits for consumers, promoting better nutrition worldwide.
Environmental Applications
The exploration of environmental applications in genetic engineering and recombinant DNA technology presents unique opportunities to address ecological challenges. These applications leverage innovative techniques to promote sustainability, enhance biodiversity, and restore ecosystems. Understanding the implications of these methods is critical as they work towards mitigating environmental damage caused by human activity.
Bioremediation
Bioremediation is a process that uses living organisms, often microorganisms, to remove or neutralize pollutants from the environment. This technique is becoming increasingly relevant in the context of soil and water pollution. Microbes that have been genetically modified can degrade hazardous substances more efficiently than their natural counterparts. For instance, certain bacteria can be engineered to digest oil spills, breaking down hydrocarbons into less harmful components.
The benefits of bioremediation are numerous:
- Cost-effective: Compared to traditional methods of waste cleanup, bioremediation often requires fewer resources and less time.
- Environmentally friendly: This method typically uses natural processes, making it less disruptive to the ecosystem.
- Versatile: It can be applied to various contaminants, including heavy metals, pesticides, and petroleum products.
However, there are some considerations. The engineering of organisms must be carefully managed to prevent unintended consequences, such as the introduction of new pathogens. Thus, regulatory frameworks must be in place to monitor and assess the use of genetically modified organisms in bioremediation efforts.
Conservation Efforts
The application of genetic engineering in conservation efforts highlights the potential of these techniques to preserve endangered species and restore habitats. One significant initiative involves identifying and modifying genes related to resilience against diseases in wildlife populations. For example, scientists might alter the genetic makeup of amphibians to enhance their resistance to chytridiomycosis, a fungal disease that has devastated global frog populations.
Using genetic tools also allows for the preservation of genetic diversity. Enhancing the genetic variability within a population is critical for its long-term survival, particularly in changing environmental conditions. Moreover, genetic technology can aid in the resurrection of species that are functionally extinct by using available genetic material.
Important considerations for conservation efforts include:
- Ethics: The manipulation of genetic material raises ethical questions about human intervention in nature.
- Ecological balance: Introducing genetically modified organisms into the wild must be done with an understanding of ecological dynamics and potential impacts.
- Public opinion: Gaining acceptance from local communities and stakeholders is crucial for the success of conservation projects that employ genetic engineering.
In summary, environmental applications of genetic engineering not only offer practical solutions to pressing ecological problems but also demand careful consideration of ethical and ecological implications. By engaging in discussions and research about these applications, stakeholders can work towards a more sustainable future.
Ethical Considerations
Ethics play a critical role in the realm of genetic engineering and recombinant DNA technology. As advancements in these fields continue to emerge, understanding the ethical implications associated with them is paramount. This section discusses the moral and social implications inherent to these technologies, as well as the regulatory framework that governs them. Key considerations include potential benefits, risks, and the responsibilities of scientists and policymakers in navigating this complex landscape.
Moral and Social Implications
The moral implications of genetic engineering are wide-ranging and complex. On one hand, the potential for curing genetic diseases, enhancing agricultural productivity, and even combating climate change presents significant societal benefits. Innovations such as gene therapy or genetically modified crops may improve public health and food security. Yet, these technologies also raise profound ethical questions.
Considerations about who benefits from such advancements often emerge. Access to biotechnological innovations can become stratified, leading to inequalities. In societies where wealth dictates access to health care and food sources, the advancements in genetic engineering could exacerbate existing disparities.
Moreover, genetic engineering poses questions of identity and individual rights. Parents may feel pressure to modify embryos for desirable traits, leading to ethical dilemmas around genetic selection. These concerns often extend to the social environment, where stigmatization of non-modified individuals can potentially occur. It is critical to discuss these moral concerns openly and engage diverse viewpoints to ensure responsible usage of genetic technologies.
Regulatory Framework
The regulatory landscape surrounding genetic engineering is intricate and varies across nations. Regulatory bodies are tasked with ensuring that scientific progress aligns with ethical principles, public safety, and environmental sustainability. In many countries, agencies like the U.S. Food and Drug Administration (FDA) and the European Food Safety Authority (EFSA) oversee the approval and monitoring of genetically engineered products.
The regulations typically address several key areas:
- Safety assessments: Comprehensive evaluations ensure that genetically modified organisms (GMOs) do not pose risks to human health and the environment.
- Transparency: Regulatory frameworks often require that consumers are informed about the presence of genetically modified ingredients.
- Long-term monitoring: Continuous observation is essential to gauge the effects on biodiversity and ecosystem dynamics.
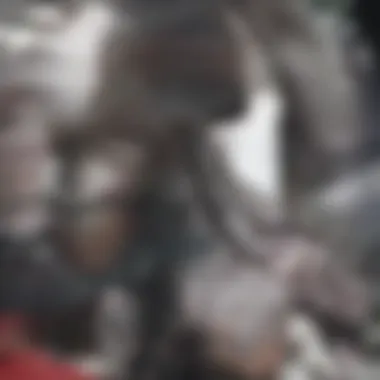
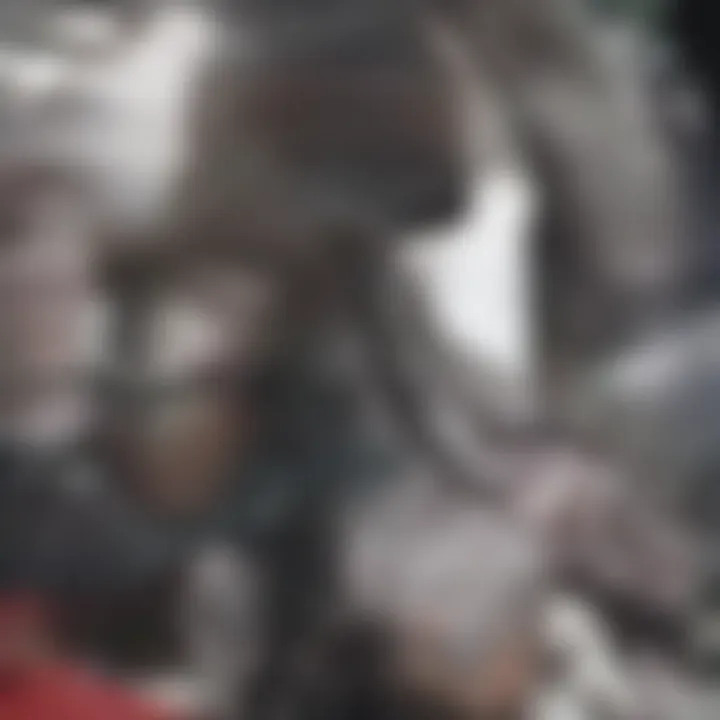
Although international agreements exist, such as the Cartagena Protocol on Biosafety, compliance and implementation can vary greatly. This complexity makes it crucial for policymakers and scientists to work collaboratively, fostering a transparent dialogue that considers ethical implications while promoting innovative advancements.
Ethical considerations in genetic engineering are not mere academic exercises; they shape future norms and practices that influence society.
Ethical considerations in genetic engineering are not mere academic exercises; they shape future norms and practices that influence society.
Legal Landscape of Genetic Engineering
The legal landscape of genetic engineering is vital for understanding how this field functions within society and in the scientific community. This area addresses the rights and responsibilities that come with manipulating genetic material. It also considers how laws influence research and commercial applications of genetic engineering. As this technology progresses rapidly, regulations must adapt to ensure safety, ethical integrity, and innovation. The legal framework impacts everything from research funding to public policy, making it a crucial component of the discussion surrounding genetic engineering.
Patenting DNA and Organisms
One of the primary legal issues in genetic engineering is the patenting of DNA and living organisms. Patents allow inventors to protect their innovations and recoup their exploratory investments. In the context of genetic engineering, companies often seek patents on genetic sequences, modified organisms, and processes. However, there are significant debates regarding the morality and implications of patenting life forms. Some argue that genes, being natural products, should not be patented, while others believe that patents are essential for encouraging innovation.
The U.S. Supreme Court case Association for Molecular Pathology v. Myriad Genetics, Inc. determined that naturally occurring genes cannot be patented. This ruling has profound implications for biotechnological research and development. It emphasized the distinction between natural phenomena and human-made inventions, setting a precedent for how genetic materials are viewed legally.
"While patents incentivize innovation, they can also create monopolistic practices that hinder access to crucial genetic materials."
"While patents incentivize innovation, they can also create monopolistic practices that hinder access to crucial genetic materials."
Investors and researchers must navigate a complex landscape of intellectual property laws to protect their innovations while also considering the ethical implications of their work.
International Agreements
The global nature of genetic engineering necessitates international agreements to regulate practices and intellectual property rights. Different countries have varying degrees of protection for genetic innovation, which can complicate collaborative research efforts. Agreements like the Convention on Biological Diversity and the Nagoya Protocol attempt to establish a framework for the equitable sharing of genetic resources and benefits derived from them.
Such agreements aim to address the needs of biodiversity conservation while supporting scientific research. Compliance with these international regulations is not merely a legal requirement; it also shapes the ethical landscape in which genetic engineering operates.
The alignment of national laws with international standards is critical for fostering trust among nations. Therefore, understanding these agreements is more than an academic exercise. It informs how biotechnological advancements are pursued and how benefits are shared globally.
Future Trends in Genetic Engineering
The landscape of genetic engineering is ever-evolving, characterized by rapid technological advancements and new methodologies that promise innovative solutions to longstanding challenges. This section discusses significant future trends that will shape the field and their relevance for society. With the advent of increasingly sophisticated tools, scientists and researchers will be better equipped to manipulate genetic material in precise ways. The benefits of these trends extend beyond basic research, influencing medical therapies, agricultural practices, and environmental restoration efforts.
Next-Generation Sequencing
Next-generation sequencing (NGS) represents a monumental leap in genetic research. This technology allows for high-throughput sequencing, meaning researchers can decode large segments of DNA far more efficiently than before. One of the most prominent advantages of NGS is its ability to analyze vast genomic sequences quickly and at a reduced cost.
The implications of NGS are profound in several fields:
- Medical diagnosis: NGS can unveil genetic disorders by identifying mutations linked to specific diseases.
- Personalized medicine: This approach enables the tailoring of treatments based on an individual’s genetic makeup, improving efficacy.
- Evolutionary biology: Researchers can explore the genetic variations that underpin diversity within and across species.
However, there are considerations that come with NGS: the challenges of data management and the ethical implications of genomic information. With the power of this technology comes the responsibility to handle genetic data vigilantly to protect individual privacy and prevent misuse.
Synthetic Biology
Synthetic biology merges biology and engineering to design and construct new biological entities. This branch of genetic engineering allows for the creation of custom organisms to perform specific functions. For example, researchers can engineer bacteria to produce biofuels or synthesize pharmaceuticals.
The potential applications include:
- Bio-manufacturing: Enabling more sustainable production methods for chemicals and goods.
- Environmental applications: Developing organisms that can break down pollutants or capture carbon dioxide.
- Healthcare innovations: Crafting living systems that can deliver drugs in a controlled manner within the body.
Despite its promise, synthetic biology raises pertinent questions regarding safety and bioethics. The capability to engineer life forms necessitates rigorous oversight to mitigate the risks of unintended consequences in ecosystems or human health.
"The future of genetic engineering relies not just on scientific discovery, but also on ethical and regulatory frameworks that guide its application in society."
"The future of genetic engineering relies not just on scientific discovery, but also on ethical and regulatory frameworks that guide its application in society."
In a world facing significant challenges ranging from disease outbreaks to environmental degradation, advancements in genetic engineering and recombinant DNA technology hold the potential to provide solutions. Through research, collaboration, and careful consideration of ethical implications, the future trends outlined here could transform various aspects of life as we know it.
The End and Implications for Society
Understanding the implications of genetic engineering and recombinant DNA technology is crucial in today’s world. These advancements offer transformative benefits across multiple fields. However, they also raise significant ethical and social issues. The power to modify genetic material means we have to consider the moral responsibilities that come along with it. The ability for scientists to create genetically modified organisms can lead to breakthroughs in medicine and agriculture, but such technologies must be employed with caution.
Summarizing Key Takeaways
- Relevance Across Fields: Genetic engineering and recombinant DNA technology hold immense potential in medicine, agriculture, and environmental science. These advancements allow for targeted treatment of diseases, enhanced crop resilience, and even bioremediation strategies to combat pollution.
- Ethical Considerations: The manipulation of life at a genetic level poses ethical challenges. Questions arise about the extent to which humans should intervene in natural processes. Issues such as biodiversity loss, unintended consequences, and health risks must be carefully evaluated.
- Legal Regulations: A structured legal framework is essential to govern the use of genetic technologies. Current laws must adapt continually to address new developments, ensuring safety while fostering innovation.
- Future Trends: With ongoing advancements in areas like synthetic biology and next-generation sequencing, the landscape of genetic engineering is rapidly evolving. Awareness of these trends can help guide responsible research practices and public policy decisions.
"The real challenge is not only to harness the power of genetic engineering but to ensure that its applications are safe, equitable, and sustainable."
"The real challenge is not only to harness the power of genetic engineering but to ensure that its applications are safe, equitable, and sustainable."
Call to Further Research and Discussion
Further exploration in genetic engineering is vital to understand its full implications. Researchers must prioritize studies that address both the benefits and the risks associated with genetic modifications. Collaborative efforts across disciplines can shed light on effective regulatory measures that align scientific innovation with societal values.
- Engagement with Stakeholders: Educators, policymakers, and scientists should actively engage with each other. By fostering dialogue, diverse perspectives can be integrated into decision-making processes.
- Public Awareness: There needs to be a concerted effort to increase public understanding of genetic technologies. Facts and ethical considerations should be communicated effectively to inform public opinion and policy.
- International Cooperation: As genetic engineering knows no borders, international cooperation is essential to ensure that best practices are shared and global agreements are upheld. This can lead to a more holistic understanding of challenges and solutions surrounding genetic manipulation.
As we continue to explore this evolving landscape, a commitment to responsible practices and transparent discussions is imperative for maximizing the benefits of genetic engineering while minimizing potential harms.