Exploring mRNA Sources and Methods for Vaccines
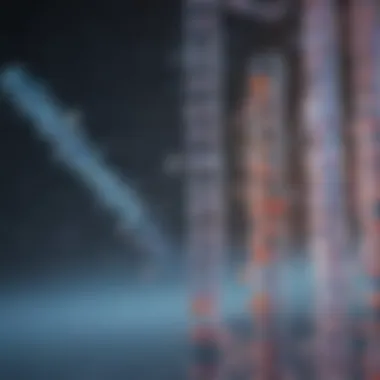
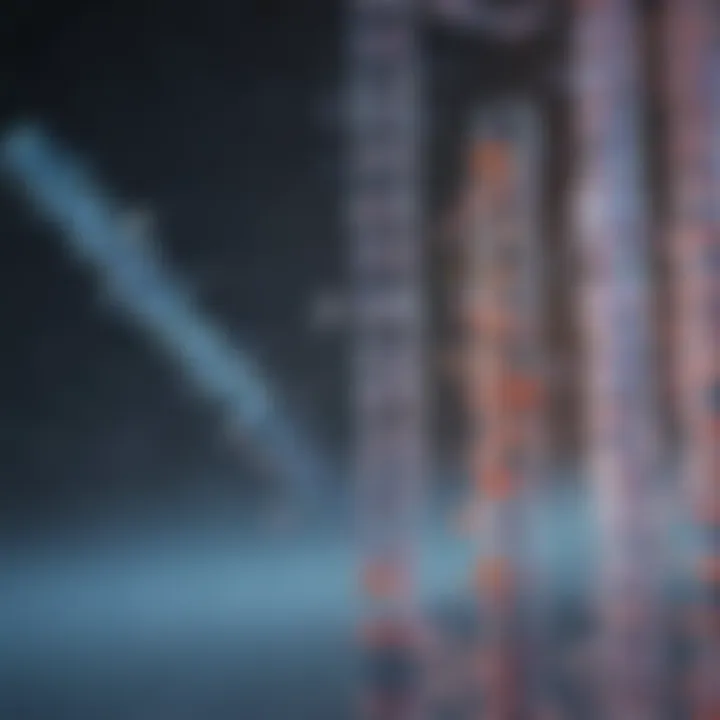
Intro
The landscape of vaccine development has evolved significantly with the introduction of mRNA technology. This innovative approach not only hastens the production of vaccines but also enhances their efficacy against infectious diseases. Understanding how mRNA is sourced and synthesized is critical for appreciating the complexities involved in this advancement. The molecules serve as the blueprint for producing viral proteins, which, in turn, elicit an immune response. Consequently, the exploration of mRNA acquisition methods offers insight into the broader implications for health and biotechnology.
Article Overview
Purpose of the Article
The primary aim of this article is to dissect the various sources and synthesis methods of mRNA used in vaccine development. By offering a thorough examination, it seeks to clarify existing knowledge gaps and promote awareness of the scientific processes behind mRNA vaccines.
Relevance to Multiple Disciplines
The topic intersects various fields, including molecular biology, immunology, and biotechnology. As this technology continues to influence global health strategies and responses to pandemics, insights into mRNA acquisition have implications for researchers, healthcare professionals, and policymakers. It fosters an understanding that transcends scientific boundaries.
Research Background
Historical Context
The concept of mRNA vaccines is not entirely new. Research into mRNA as a vaccine platform began in the early 1990s. However, it gained unprecedented attention following the COVID-19 pandemic. Companies like Pfizer and Moderna successfully employed this technology to develop vaccines within a remarkable timeframe. The historical development provides valuable context for understanding the increased reliance on mRNA in modern medicine.
Key Concepts and Definitions
It is essential to understand several key terms related to mRNA vaccines:
- Messenger RNA (mRNA): This type of RNA serves as a template for protein synthesis in cells.
- Transfection: The process of introducing nucleic acids into cells, which is pivotal for mRNA delivery.
- Lipid Nanoparticles: These are tiny particles used to encapsulate mRNA strands, facilitating their transport into cells.
- Synthesis: The process of creating mRNA from DNA templates in a lab setting, often using in vitro techniques.
This foundational knowledge is critical as we delve deeper into the sources and methodologies utilized in the development of mRNA vaccines.
Prelude to mRNA Vaccines
The advent of mRNA vaccines marks a pivotal moment in immunology and public health. These vaccines represent revolutionary technology that has been rapidly developed and deployed to address global health crises, particularly COVID-19. Understanding the underlying principles and sources of mRNA is crucial for comprehending how these vaccines function and their potential for future applications.
Understanding mRNA
Messenger RNA, or mRNA, is a single-stranded molecule that plays a crucial role in the process of gene expression. It acts as an intermediary between DNA and protein synthesis. In the context of vaccines, synthetic mRNA is designed to instruct cells to produce a specific protein. This protein is typically a harmless component of a pathogen, such as the spike protein of the SARS-CoV-2 virus. Once produced, this protein triggers an immune response, teaching the body to recognize and combat the actual virus if exposed in the future.
The design and synthesis of mRNA require precise scientific knowledge and techniques. Synthetic mRNA is carefully crafted in laboratories, ensuring that the structure and sequence align with the target protein's genetic blueprint. Researchers focus on several factors, including mRNA stability, efficiency of translation, and the ability to stimulate a robust immune response. Understanding these elements is essential, as they determine the effectiveness of the vaccine.
The Role of mRNA in Vaccines
mRNA serves as the backbone of many modern vaccines, particularly those aimed at preventing infectious diseases. By using mRNA, vaccine developers can create a safer and more efficient way to elicit an immune response. Unlike traditional vaccines, which often use weakened or inactivated pathogens, mRNA vaccines contain no live virus. This feature reduces the risk of disease associated with vaccination.
The benefits of mRNA vaccines extend beyond safety. Their design process allows for rapid modifications. When new variants of a virus emerge, updating the mRNA sequence is relatively straightforward. Such adaptability is crucial in a fast-evolving landscape of viral pathogens.
In summary, mRNA is a powerful tool in vaccine development, providing a faster and safer avenue for combating infectious diseases. Its role in modern vaccines cannot be overstated, as it lays the groundwork for future innovations in immunization technologies. The ongoing research in this area promises to expand the applications of mRNA beyond infectious diseases to possibly include cancer and other medical conditions.
Historical Context of mRNA Technology
Understanding the historical context of mRNA technology is essential to appreciate the complexities behind its development and the vaccines derived from it. This background provides insight into the evolution of scientific thought regarding genetic material, as well as the exploration of therapeutic possibilities using mRNA. The emergence of mRNA vaccines represents a culmination of decades of research that not only laid the groundwork for current applications but also established a promising pathway for future medicinal advancements.
Early Research on mRNA
The inception of research into mRNA can be traced to the early 1960s. At that time, scientists were beginning to decipher the role of mRNA as a messenger between DNA and protein synthesis. The groundbreaking work by researchers such as Sydney Brenner, François Jacob, and Jacques Monod provided a framework for understanding how genetic information is transcribed and translated into functional proteins. Their studies demonstrated that mRNA serves as the intermediary that relays genetic instructions from DNA to ribosomes, where proteins are assembled.
In the ensuing years, investigations into the properties and behavior of mRNA became more focused. The discovery of mRNA’s crucial function opened avenues for exploring its potential as a therapeutic agent. However, translating these early theoretical insights into practical applications remained a challenging prospect for researchers.
Development of mRNA Vaccines
The journey toward mRNA vaccines began in earnest in the 1990s, although the groundwork was laid much earlier. Scientists like Katalin Karikó and Drew Weissman contributed significantly to making mRNA a viable platform for vaccines. Their research centered on modifying mRNA to enhance stability and translation efficiency within the cell, thus overcoming earlier challenges that hindered its application in immunization.
The breakthrough came with the use of lipid nanoparticles, which serve as delivery mechanisms for mRNA. This innovation allowed for more effective transport of mRNA into human cells, significantly boosting the efficacy of vaccines. The application of mRNA technology surged during the COVID-19 pandemic, leading to the rapid development of vaccines like those from Moderna and Pfizer-BioNTech.
These vaccines leverage the instructions contained in synthetic mRNA to prompt the immune system to recognize and combat the virus effectively. The success of these vaccines has not only changed the landscape of public health but also showcased the versatility of mRNA technology. It illustrates how a fundamental understanding of molecular biology can translate into life-saving innovations.
"The emergence of mRNA vaccines has provided public health with unprecedented speed and adaptability, showcasing how foundational science can lead to direct and impactful therapeutic solutions."
"The emergence of mRNA vaccines has provided public health with unprecedented speed and adaptability, showcasing how foundational science can lead to direct and impactful therapeutic solutions."
Sources of mRNA for Vaccines
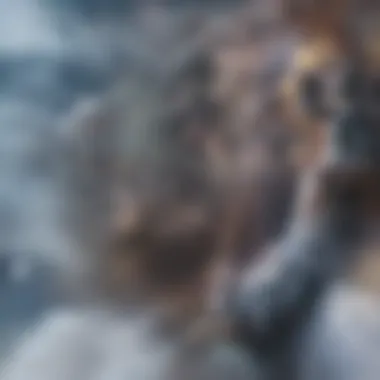
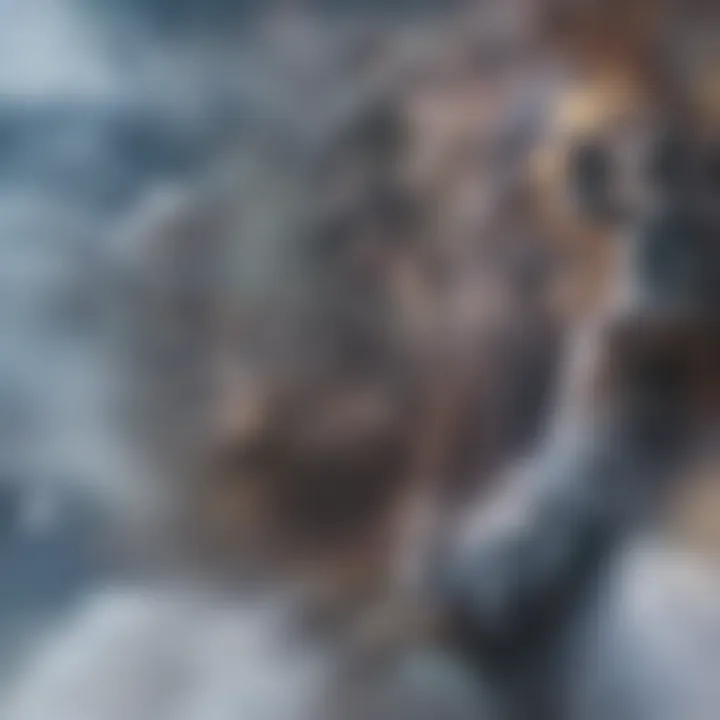
The sourcing of mRNA is integral to the development of vaccines, making it a vital discussion point in this article. Understanding this topic unveils the foundation upon which mRNA vaccine technology stands. Effective vaccine design relies on having high-quality mRNA that can elicit an immune response. Identifying sources of mRNA can also have implications for scalability and regulatory compliance.
Synthetic Production of mRNA
Synthetic production of mRNA has revolutionized how vaccines are developed. Through this method, scientists can create custom mRNA sequences to target specific pathogens. The process involves using in vitro transcription, where DNA templates are transcribed into mRNA using RNA polymerases. This allows for precise control over the mRNA sequence, which is crucial for ensuring efficacy. Synthetic production is advantageous because it can be scaled up rapidly during pandemics. Also, it facilitates the quick adaptation of vaccines in response to emerging variants of viruses, which is essential for maintaining public health.
Natural Sources of mRNA
Natural sources of mRNA play a critical role in vaccine development, offering both benefits and challenges. These sources include both viral and cellular origins, each having unique characteristics.
From Viruses
Using mRNA derived from viruses is a prevalent strategy in vaccine development. The key characteristic of this method is its ability to harness a virus's own genetic instructions to produce proteins that can trigger immune responses. This approach is beneficial because it can closely mimic how natural infections work, thereby enhancing vaccine effectiveness.
However, there are considerations involved. The unique feature of using viral mRNA is its efficiency in eliciting a strong immune reaction due to its biological design meant for rapid replication and protein production. Yet, one must also weigh the disadvantages, such as potential safety concerns arising from using live-virus components and the necessity for careful purification processes.
From Cells
Extracting mRNA from cells is another method used to create vaccines. This approach involves isolating mRNA from human or animal cells. The key characteristic here is the potential to utilize the host's cellular machinery for producing mRNA that matches the antigens of a targeted pathogen. This method is advantageous because it allows for the incorporation of post-transcriptional modifications that enhance mRNA stability and translation efficiency.
One unique feature of cell-derived mRNA is its complex structure, which can lead to better translation into proteins. However, the disadvantages include the challenges in scalability and the potential for variability in mRNA quality based on the source. Overall, both sources provide valuable pathways for obtaining mRNA and highlight the diverse methods that can be employed in vaccine development.
Synthesis of mRNA
The synthesis of mRNA is a pivotal element in the development of effective mRNA vaccines. Understanding the nuances of this process provides insight into the reliability and efficiency of vaccine production. Through various methods, researchers can create mRNA that mimics natural processes, ensuring that the immune system responds appropriately. This section explores the different methods and considerations involved in synthesizing mRNA, emphasizing their significance in the context of vaccine development.
In Vitro Transcription Methods
In vitro transcription is one of the primary techniques used to synthesize mRNA for vaccines. This method involves using a DNA template to produce RNA. It is favored for its efficiency and the ability to produce large quantities of mRNA quickly.
A major component of in vitro transcription involves RNA polymerases, which are enzymes that catalyze the synthesis of RNA from a DNA template. T7, T3, and SP6 RNA polymerases are commonly used, each depending on the specific promoter sequence in the DNA template. This selective choice of polymerase plays a significant role in determining the yield and quality of the mRNA produced. Factors such as temperature, nucleotides, and reaction time must be optimized to achieve high integrity and functional mRNA.
Selection of Template DNA
The choice of template DNA is crucial in mRNA synthesis. It includes aspects such as the promoter region and the sequence that encodes the desired protein. A strong promoter ensures robust expression of mRNA, while the sequence must align with the goals of vaccination.
There are generally two types of DNA templates: plasmid DNA and linearized DNA. Plasmid DNA can be amplified and is often used for bulk production. Linearized DNA, on the other hand, provides a more straightforward approach for synthesis as it eliminates the need for additional steps to prepare the DNA. Researchers must consider the purpose of the mRNA and the efficiency of its intended use when selecting the template. This decision directly impacts the effectiveness of the resulting vaccine.
Purification Processes
After synthesis, the purity and integrity of the mRNA must be assessed. Purification processes are essential to eliminate contaminants such as residual DNA, proteins, and nucleotides. Common purification methods include phenol-chloroform extraction and column chromatography.
- Phenol-chloroform extraction is a classic technique that separates RNA from proteins and DNA based on solubility differences. This method is effective but requires careful handling due to the toxic chemicals involved.
- Column chromatography utilizes resin-based methods to selectively isolate mRNA. It ensures high purity and can be scaled for large production.
- Gel electrophoresis can also verify the integrity of mRNA by separating it based on size, allowing researchers to confirm that the desired mRNA length has been achieved.
The success of mRNA vaccines hinges upon the meticulous adherence to synthesis and purification protocols. Even minor deviations can affect vaccine efficacy.
The success of mRNA vaccines hinges upon the meticulous adherence to synthesis and purification protocols. Even minor deviations can affect vaccine efficacy.
In summary, the synthesis of mRNA is a complex but critical process involving in vitro transcription, careful selection of template DNA, and rigorous purification methods. Each step requires precision and optimization to ensure the resulting mRNA is suitable for use in vaccines. Understanding these processes enhances the overall appreciation for the scientific achievements underlying mRNA vaccine technology.
Quality Control in mRNA Production
Quality control is vital in the production of mRNA vaccines. It ensures that the mRNA synthesized is both effective and safe for human use. The process of quality control encompasses several critical elements, each addressing different stages of mRNA production. Ensuring integrity, purity, and optimal yield are fundamental aspects that directly impact the efficacy of the resulting vaccine.
Quality control not only verifies the final product but also confirms that each step of the production process adheres to stringent standards. This reduces risks associated with mRNA degradation or contamination, which can arise during synthesis, purification, and storage. Proper quality control measures contribute to the overall reliability of mRNA vaccines in public health initiatives.
Testing for Integrity and Purity
Testing for integrity and purity of mRNA is a cornerstone of quality control. This involves a series of assessments to confirm that the mRNA molecules are complete and free from contaminants. The structure of mRNA must remain intact; otherwise, its ability to encode the target protein can be compromised.
Some common methods used for testing integrity include:
- Gel electrophoresis: This technique helps visualize the size and integrity of the mRNA, revealing whether the molecules are intact or degraded.
- Nanodrop spectrophotometry: This method quantifies the concentration of mRNA while also providing a purity ratio by measuring absorbance at specific wavelengths.
- Reverse transcription quantitative PCR (RT-qPCR): This sensitive technique can detect specific RNA sequences, ensuring that the intended mRNA transcript is present and in sufficient quantities.
Additionally, purification steps are closely monitored. Contaminants such as DNA, proteins, or other RNA species must be eliminated to uphold mRNA purity. A clean product leads to more reliable vaccine performance and safety for recipients.
Optimizing Yield
Optimizing yield is another crucial aspect of quality control in mRNA production. Yield refers to the amount of mRNA generated in relation to the starting material, which affects the cost and efficiency of vaccine production. Higher yields can make the manufacturing process more sustainable and cost-effective.
Several factors influence yield, including:
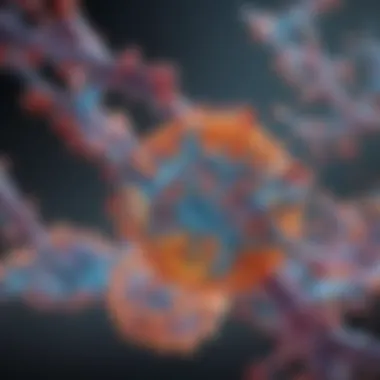
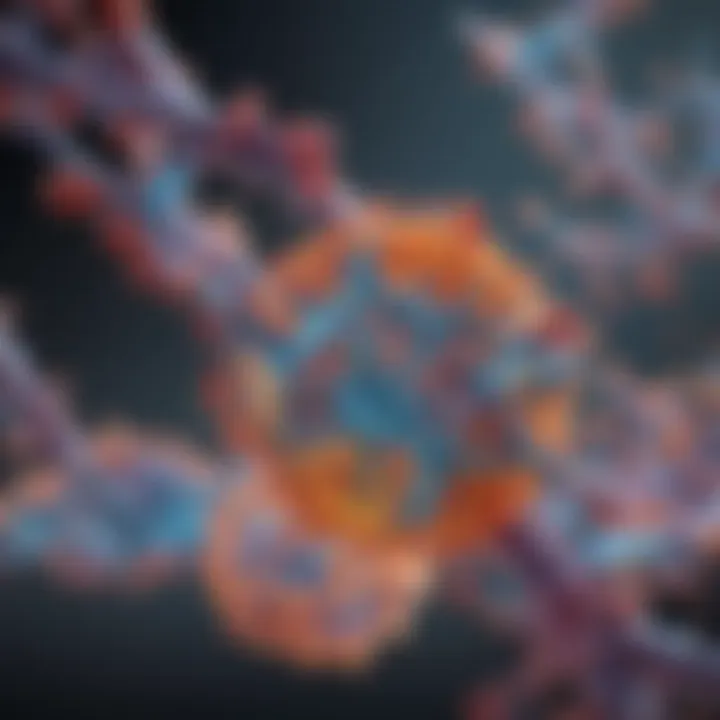
- Template DNA quality: The quality of the template DNA used in in vitro transcription has a direct effect on mRNA yield. Ensuring high purity and integrity of template DNA is essential.
- Transcription conditions: Parameters such as temperature, enzyme concentrations, and reaction time can be fine-tuned to maximize yield. Each optimization contributes to more efficient transcription processes.
- Purification methods: The methods employed for purification can also influence yield. Techniques that effectively isolate mRNA from other products without significant loss can enhance overall yield.
Ultimately, by focusing on optimizing yield, companies can improve their vaccine production capabilities while maintaining high standards of safety and efficacy. This will be essential as demand for mRNA vaccines continues to grow in response to public health needs.
"Effective quality control in mRNA production not only protects patient safety but also ensures that public trust in vaccines remains intact."
"Effective quality control in mRNA production not only protects patient safety but also ensures that public trust in vaccines remains intact."
By ensuring robust testing for integrity and purity, while optimizing yield, the mRNA production process can achieve its goal of providing safe and effective vaccines.
Key Players in mRNA Vaccine Development
The development of mRNA vaccines has been an intricate process, characterized by the collaboration and innovation of various entities. Understanding the key players involved offers insight into the dynamics of this groundbreaking technology. The players consist primarily of pharmaceutical companies, research institutions, and collaborative networks that combine resources and expertise.
Pharmaceutical Companies
Pharmaceutical companies are at the forefront of mRNA vaccine development. They play a crucial role in the research, synthesis, and commercialization of mRNA vaccines. Leading companies like Pfizer and Moderna took significant leaps in the mRNA technology landscape during the COVID-19 pandemic. Their investment in research and development facilitated rapid vaccine deployment.
Benefits of pharmaceutical companies include:
- Financial Resources: They have the capital necessary for large-scale production and clinical trials.
- Regulatory Knowledge: These companies navigate complex regulatory environments to achieve approvals efficiently.
- Manufacturing Capabilities: Advanced manufacturing facilities help in producing vaccines at scale, ensuring global availability.
Research Institutions
Research institutions contribute significantly to the foundational science underpinning mRNA technology. They conduct basic research that drives innovation in synthesis techniques and delivery methods. Organizations such as the National Institutes of Health (NIH) play a vital role in advancing our understanding of mRNA biology and its application in vaccines.
Key considerations about research institutions include:
- Innovation: These institutions often lead in developing new methodologies that enhance mRNA vaccine effectiveness.
- Academic Collaborations: Partnerships with industry enhance the commercialization potential of academic discoveries.
- Funding Opportunities: Research grants and government funding facilitate ambitious projects that can pave the way for new vaccine solutions.
Collaborative Networks
Collaborative networks are essential for successful mRNA vaccine development. These networks often incorporate multiple stakeholders, including academia, industry, and governmental agencies. The nimbleness provided by such networks accelerates research and development timelines.
Considerations for collaborative networks include:
- Cross-disciplinary Expertise: By bringing together diverse expertise, these networks can tackle complex challenges more effectively.
- Shared Resources: Collaborations often allow for the sharing of resources, including technology platforms and data.
- Increased Visibility: Networking can enhance the visibility of new findings, facilitating faster adoption and integration into practice.
The interplay among pharmaceutical companies, research institutions, and collaborative networks is crucial for driving forward mRNA vaccine innovation.
The interplay among pharmaceutical companies, research institutions, and collaborative networks is crucial for driving forward mRNA vaccine innovation.
Regulatory Framework Governing mRNA Vaccines
The significance of the regulatory framework governing mRNA vaccines cannot be overstated. As these vaccines represent a novel approach to immunization, ensuring their safety and efficacy is of utmost importance. The regulatory guidelines establish a comprehensive system that oversees the development, testing, and distribution of these vaccines. This framework is designed to protect public health while also fostering innovation in vaccine technology.
Key elements of the regulatory framework include compliance with safety protocols, evaluation of clinical trial data, and post-market surveillance. These components work together to create a robust structure that can respond to both scientific advancements and potential public health threats.
Furthermore, the regulatory framework affects multiple stakeholders, including pharmaceutical companies, healthcare providers, and patients. Adherence to these regulations helps instill public confidence in mRNA vaccines, ensuring that the benefits of vaccination outweigh any associated risks. In this section, we will detail the specific guidelines set by the FDA and the international regulations that govern mRNA vaccines.
FDA Guidelines for mRNA Vaccines
The Food and Drug Administration (FDA) plays a pivotal role in the regulation of mRNA vaccines in the United States. The guidelines set forth by the FDA are designed to ensure that vaccines are safe, effective, and manufactured consistently.
- Preclinical Testing: Before any clinical trials, mRNA vaccines undergo preclinical testing in laboratory settings. This stage assesses toxicity and the immune response in animal models.
- Clinical Trials Phases: The clinical trial process is divided into three phases:
- Emergency Use Authorization (EUA): In public health emergencies, the FDA may provide an EUA, allowing for quicker access to vaccines while still ensuring that they meet adequate safety and efficacy standards. This pathway was notably used during the COVID-19 pandemic, allowing for the rapid deployment of mRNA vaccines like those from Pfizer-BioNTech and Moderna.
- Post-Market Surveillance: After approval, the safety of mRNA vaccines continues to be monitored through various systems, including the Vaccine Adverse Event Reporting System (VAERS). This ongoing assessment is crucial for identifying any rare side effects that may not have been evident in clinical trials.
- Phase 1: Focuses on safety, dosing, and the immune response in a small group of participants.
- Phase 2: Expands the cohort to further assess safety and begins to evaluate efficacy.
- Phase 3: Involves thousands of participants to determine the vaccine’s effectiveness and monitor adverse reactions over a longer duration.
The FDA thus employs a thorough approach to ensure that mRNA vaccines are introduced safely into the market, significantly contributing to public health.
International Regulations
International regulations are essential for harmonizing vaccine approval processes across countries. Different regions may have various requirements, but organizations like the World Health Organization (WHO) offer guidance to facilitate global trust in vaccines.
Each country has its own regulatory body similar to the FDA. For example, in the European Union, the European Medicines Agency (EMA) oversees the evaluation of mRNA vaccines. The process includes:
- Centralized Authorization Procedure: This allows a single application to cover multiple EU member states, streamlining the approval process for mRNA vaccines.
- Periodic Safety Update Reports (PSURs): Manufacturers must submit regular reports to track the safety of vaccines and identify any emerging concerns.
- Quality Assurance: Pre- and post-market quality checks are mandatory, ensuring that manufacturers adhere to Good Manufacturing Practice (GMP).
Overall, the goal of international regulations is to ensure that mRNA vaccines are both safe and effective, promoting their acceptance worldwide while minimizing the risks associated with their deployment.
Overall, the goal of international regulations is to ensure that mRNA vaccines are both safe and effective, promoting their acceptance worldwide while minimizing the risks associated with their deployment.
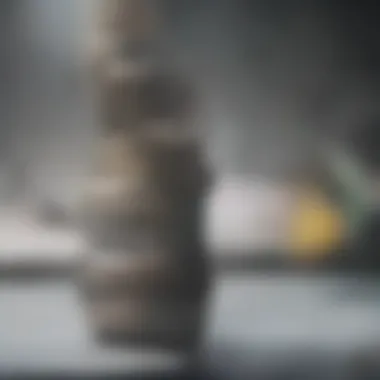
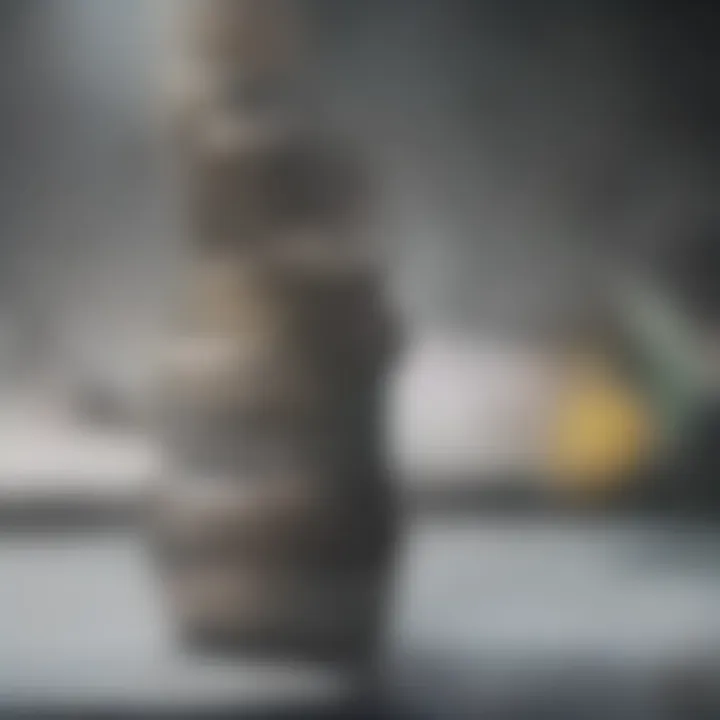
Challenges in mRNA Vaccine Production
The manufacture of mRNA vaccines involves various complexities that can significantly impact their availability and effectiveness. This section highlights the crucial elements defining the challenges in mRNA vaccine production and emphasizes the need for efficient processes to address these concerns.
Scalability of Production
Scalability is one of the foremost challenges in mRNA vaccine production. As demand for vaccines rises, particularly in times of public health emergencies, the production must increase rapidly.
Achieving scalability involves several components, including:
- Bioreactor Size: Increasing the capacity of bioreactors can help produce larger volumes of mRNA. However, this raises concerns regarding consistency and quality control.
- Streamlining Processes: Optimization of synthesis and purification processes is essential. Any inefficiencies at this stage can bottleneck production.
- Supply Chain Management: The availability of raw materials like plasmids and enzymes can affect the scalability of production. Managing these supplies effectively is critical.
Ensuring that production can meet the urgent needs without sacrificing quality is paramount.
Cost Considerations
Cost is a vital aspect of mRNA vaccine production that can affect the potential for widespread vaccination. The economic implications associated with producing these vaccines involve a number of factors:
- Development Costs: Initial costs related to research, development, and clinical trials can be quite high. This impacts the pricing of the final vaccine.
- Production Costs: Expenses linked to raw materials, labor, and technology influence the overall production cost.
- Economies of Scale: If production is not scaled effectively, costs will remain high, limiting accessibility to the vaccines.
The interplay of these factors can pose significant barriers to deploying vaccines efficiently across populations.
Addressing these challenges is crucial for realizing the potential of mRNA vaccines in future public health initiatives.
Addressing these challenges is crucial for realizing the potential of mRNA vaccines in future public health initiatives.
Future Directions for mRNA Technology
The field of mRNA technology is poised for substantial growth and expansion. As the understanding of mRNA’s mechanisms continues to evolve, researchers are exploring novel applications beyond vaccines, indicating a pivotal shift in its potential uses. This section delves into the importance of these developments in the broader context of biomedical advancements.
Potential Applications Beyond Vaccines
The focus of mRNA extends well beyond infectious disease prevention. One of the most promising applications is in the area of cancer therapy. mRNA can be designed to encode for tumor-specific antigens, enabling the immune system to recognize and attack cancer cells more effectively. This personalized approach may lead to more successful outcomes in treating various cancer types.
Additionally, mRNA has potential uses in protein replacement therapies for genetic disorders. Disorders like cystic fibrosis or hemophilia could benefit from treatments that deliver mRNA encoding functional versions of deficient proteins, providing a solution for patients who lack these essential components.
This versatility expands the implications of mRNA technology into fields such as regenerative medicine. For instance, mRNA could facilitate tissue engineering by instructing cells to produce necessary growth factors or extracellular matrix components required for tissue regeneration.
Innovations in Synthesis Techniques
As mRNA technology progresses, synthesis techniques are adapting and improving. Innovations in this area are crucial for enhancing the scalability and efficiency of mRNA production. Current methods like in vitro transcription have undergone refinements to increase yields and decrease production times, making the synthesis process more cost-effective.
Recent advancements include the use of nanoparticle delivery systems. These systems can enhance the efficiency and stability of mRNA, improving its performance when introduced into human cells. Furthermore, research is underway into optimized template construction, which can streamline the synthesis process and further enhance the purity and integrity of the final product.
Moreover, automation plays a key role. Automated systems for mRNA synthesis can increase throughput and minimize human error. This shift not only improves the reliability of mRNA production but also lowers the costs associated with scaling the technology for wide-ranging applications.
In summary, the future of mRNA technology holds immense potential. As researchers explore various applications and improve synthesis methods, the possibilities for mRNA's role in medicine are becoming increasingly expansive.
Finale
The conclusion of this article encapsulates the crucial aspects of mRNA acquisition for vaccine development. Understanding the sources and methods for obtaining mRNA is essential. This knowledge empowers researchers and practitioners in the field to navigate the complexities involved in vaccine production effectively.
One significant element is the transition from natural and synthetic sources. Synthetic production of mRNA has revolutionized vaccine development, providing greater control over the mRNA sequence and characteristics. In contrast, natural sources provide insights into how mRNA operates within biological systems, potentially offering leads for innovation.
Another key point is the synthesis methods. The processes involved in synthesizing mRNA, such as in vitro transcription, are fundamental to ensuring high-quality mRNA. This involves precise selection of template DNA and robust purification processes to achieve the desired integrity and purity.
Addressing quality control has notable benefits as it ensures vaccines produced are consistent and reliable. Optimized yield directly correlates with cost-effectiveness, further enhancing the feasibility of mRNA vaccines on a larger scale.
Finally, recognizing the ecological and collaborative frameworks facilitates a more extensive understanding of how advancements in mRNA technology are achieved. The partnership between pharmaceutical companies and research institutions strengthens innovation, propelling the field forward.
In summary, grasping the sources and methods of mRNA acquisition is a defining aspect of developing successful mRNA vaccines. This comprehension is vital not just for current applications but also for paving the way for future advancements in vaccine technology and potential therapeutic interventions beyond infectious diseases.
Key Benefits of References:
- Verification of Claims: Reliable references substantiate the assertions made in mRNA research, ensuring integrity in scientific communication.
- Historical Context: They enable readers to trace the evolution of mRNA technologies, offering insights into prior challenges and breakthroughs.
- Cross-disciplinary Insights: Access to a wide array of publications can spark new ideas and foster collaboration among various scientific disciplines.
Considerations About References:
- Quality Over Quantity: Sifting through numerous sources to select those that have undergone rigorous peer review is crucial.
- Relevance: Staying current with the latest research and methodologies is essential, as findings in this field can change rapidly.
- Diversity of Sources: Incorporating articles from different fields, countries, and institutions can enrich the narrative and lead to a broader understanding of mRNA technology.
As the field of mRNA vaccines continues to advance, maintaining a continually updated and well-sourced reference list is imperative for all stakeholders involved.
As the field of mRNA vaccines continues to advance, maintaining a continually updated and well-sourced reference list is imperative for all stakeholders involved.