The Organic Molecule Drawer: An In-Depth Analysis
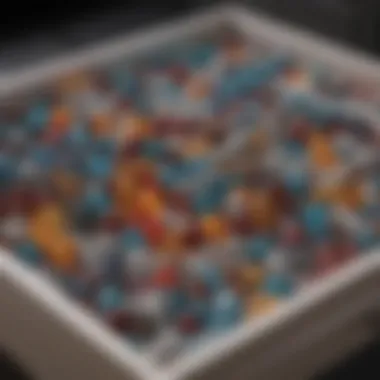
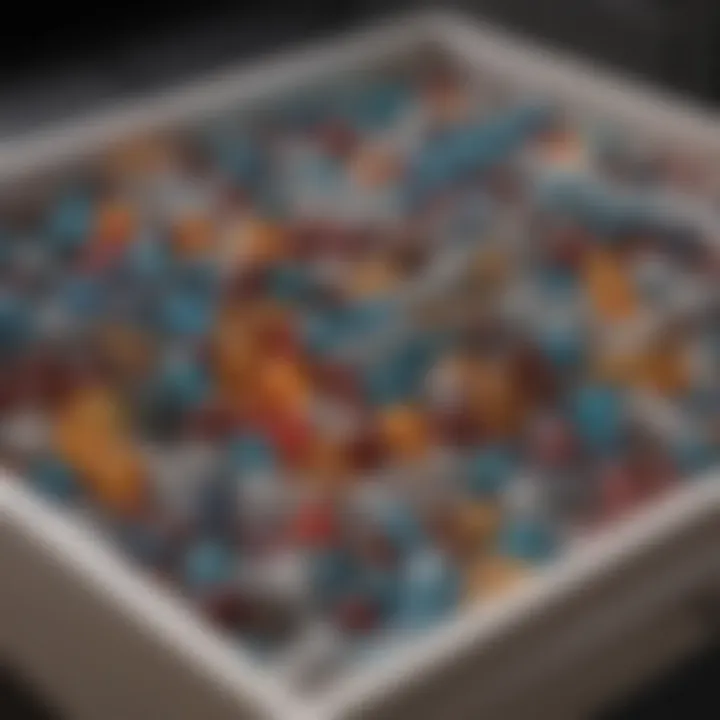
Intro
In the realm of chemistry, the need to systematically categorize and analyze vast networks of organic compounds is paramount. The organic molecule drawer emerges as a crucial construct that simplifies this task. It serves as more than just a metaphor; it's a comprehensive system aiding chemists, researchers, and educators alike. By organizing these myriad compounds, we not only facilitate easier access to information but also enhance understanding and promote scientific inquiry.
This exploration will take a closer look at how the organic molecule drawer functions in various contexts, its historical roots, and its significant implications across diverse fields. From fundamental principles to digital integration, our investigation promises to cover a broad spectrum of knowledge that viewers can tap into for both educational and practical applications.
Preamble to Organic Molecules
The world of organic molecules is vast and intricate, serving as a foundation for countless disciplines including chemistry, biology, and materials science. Understanding organic molecules is not just an academic exercise; it is essential for unraveling the complexities of life itself. As we venture into this exploration, we will discover not only the structure and function of these molecules but also their historical significance and their continuous impact on scientific advancement.
Defining Organic Molecules
At its core, an organic molecule is defined by the presence of carbon atoms, often bonded to hydrogen, oxygen, nitrogen, and other elements. The diverse combinations of these elements form the backbone of organic chemistry, leading to a myriad of compounds, ranging from the simplest hydrocarbons to complex biomolecules like proteins and nucleic acids. In distinguishing organic molecules, one must consider the role of functional groups - specific groupings of atoms that impart unique chemical properties to the compounds. For example, in alcohols, the hydroxyl group (-OH) is a defining feature that affects reactivity and solubility.
One might say, when it comes to defining organic molecules, carbon is king. This pivotal element enables an incredible range of functionalities, which is why organic compounds are central to biochemical reactions. It’s crucial to grasp that organic molecules can be found everywhere; they span from the food we consume to the pharmaceuticals that treat our illnesses, making their study immensely relevant.
Historical Context of Organic Chemistry
The historical roots of organic chemistry run deep. Chemistry as a discipline engaged with organic substances started gaining ground in the 19th century, although its origins can be traced back even further. Initially, organic compounds were considered to be derived only from living organisms. This fallacy was debunked with Friedrich Wöhler's synthesis of urea in 1828, showing that organic compounds could indeed be manufactured from inorganic materials.
As time marched on, systemic approaches to cataloging these compounds began to emerge. The creation of structural formulas, the development of the periodic table, and advances in spectroscopy led to the enlightenment of how we understand molecular structures. The late 1800s were particularly fertile ground for breakthroughs, introducing concepts like isomerism and functional groups, which are central to today's organic molecule identification.
“To understand organic molecules, one must journey through the annals of history where chemistry transformed from folklore to a structure of scientific rigor.”
“To understand organic molecules, one must journey through the annals of history where chemistry transformed from folklore to a structure of scientific rigor.”
Today, organic chemistry isn't just a historical saga – it's dynamic and progressive. The ongoing research continues to unveil the multitude of possibilities that organic molecules present, highlighting their importance for both academic inquiry and practical applications in industries ranging from pharmaceuticals to polymers. The narrative of organic molecules is a continuum of discovery, understanding, and innovation.
Understanding the Organic Molecule Drawer
Understanding the organic molecule drawer involves more than just a theoretical appreciation; it’s about recognizing its vital role in the organization and analysis of organic compounds. By grasping this concept, students, researchers, and educators can leverage these systems for a more effective approach to chemical studies. As the field of chemistry continues to grow, the tools used to manage organic molecules become increasingly significant.
Concept and Definition
At its core, the organic molecule drawer can be seen as a conceptual storage space. This 'drawer' encompasses everything from a simple database to sophisticated software systems that organize and display a broad range of organic compounds. These molecules not only include the conventional hydrocarbons, but also intricate frameworks involving heteroatoms and various functional groups.\n
To further elaborate, one could liken it to a librarian organizing a vast collection of books. Each book, representing a different organic compound, must be categorized effectively to allow for easy retrieval and study. This ensures that researchers can focus their time on scientific inquiry rather than becoming bogged down in the search for specific compounds.
The concept extends beyond mere organization; it is a crucial aspect of modern chemistry. The ability to efficiently navigate through complex libraries of molecular data significantly enhances research outcomes.
Physical vs. Digital Molecule Drawers
When distinguishing between physical and digital molecule drawers, the discussion often emphasizes the advantages of each system, alongside considerations for users.
- Physical Molecule Drawers
These may include traditional filing systems, where compounds are well labeled and categorized in labs. Often, physical samples allow for hands-on analysis, providing an immediate tactile experience. However, they come with limitations such as space constraints and challenges in keeping information up-to-date. - Digital Molecule Drawers
In contrast, digital molecule drawers utilize cutting-edge software to manage data. They are dynamic and can update information rapidly, accommodating new discoveries with ease. Such systems often allow researchers to input structural data, perform computational modeling, and even simulate chemical reactions. This digital transition facilitates a collaborative environment, essential for current scientific endeavors.
"Digital molecule drawers not only enhance efficiency but also foster collaboration across the globe, breaking down borders in chemistry research."
"Digital molecule drawers not only enhance efficiency but also foster collaboration across the globe, breaking down borders in chemistry research."
Inadequate organization can lead to wasted time and can potentially derail important research endeavors. By employing both physical and digital systems judiciously, chemists can craft a more effective approach to managing their molecular libraries. This blend enables a more holistic understanding of organic chemistry, marrying the tangible with the innovative.
The Role of Organic Molecule Drawers in Research
Understanding the role of organic molecule drawers in research is akin to appreciating the backbone of a well-structured building. Without it, much of the fragile structure we call organic chemistry would slump into disarray, disrupting the flow of information crucial for scientific exploration. The organization of chemical data not only enhances the efficiency of research but significantly amplifies the potential for groundbreaking discoveries.
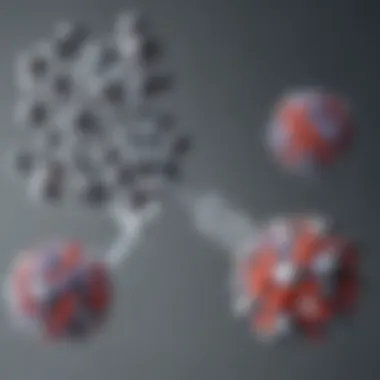
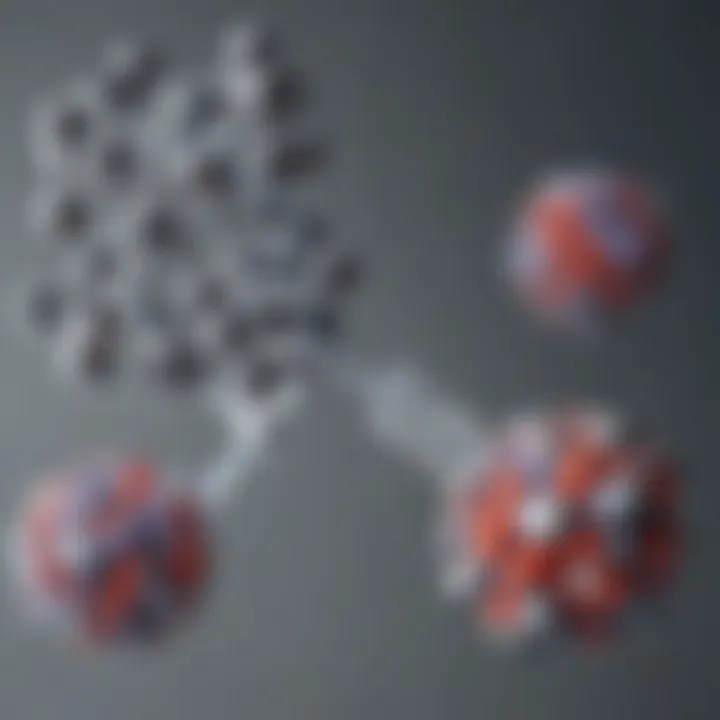
Facilitating Chemical Research
Organic molecule drawers play a key role in facilitating chemical research by offering a coherent framework through which scientists can systematically study and retrieve molecular information. The practical implications are multifaceted:
- Enhanced Accessibility: By organizing compounds into distinct categories, researchers can swiftly access the data they need. This streamlines the process, allowing for quicker decision-making in experimental design.
- Identification of Patterns: Having a well-organized dataset enables scientists to spot relationships and trends among different compounds. This is fundamental to hypothesis generation and subsequently, validates or refutes scientific theories.
- Data Visualization: Many digital drawers integrate advanced visualization tools, which help in representing complex molecular structures in a comprehensible manner. For instance, through 3D models, researchers can grasp the spatial arrangement of atoms, making it easier to predict molecular interactions.
- Collaborative Opportunities: Sharing organized molecular data with peers through digital platforms fosters collaboration across disciplines. By offering a common language through well-defined classifications, researchers can work together more effectively, leading to synergistic advances in various fields.
Case Studies in Molecular Organization
Delving into specific case studies within the realm of molecular organization illuminates how organic molecule drawers translate theoretical benefits into real-world innovations. One noteworthy example includes the integration of the Cambridge Structural Database (CSD) into pharmacological research.
Researchers utilized the CSD to explore new drug compounds aimed at combating antibiotic resistance. The organized structure allowed the team to rapidly identify existing molecular frameworks that shared structural features with known antibiotics. They could then modify these compounds, testing variations in molecular structure against bacterial strains. This iterative testing approach, supported by an organized database, led to the discovery of several promising candidates for future antibiotic development.
"A well-categorized molecule drawer isn’t just about neatness; it’s about unlocking potential that’s buried under heaps of unstructured data."
"A well-categorized molecule drawer isn’t just about neatness; it’s about unlocking potential that’s buried under heaps of unstructured data."
Another case study involves a university initiative that applied digital organic molecule drawers within its curriculum. By adopting tools such as Jmol, students were encouraged to interact with molecular structures directly. This hands-on experience demystified complex theories, allowing students to visualize molecular geometry while enhancing their understanding of stereochemistry. The immersive learning environment cultivated deeper interest and improved retention of information that would traditionally be difficult to grasp.
Organizing Organic Molecules: Methodologies
Organizing organic molecules is a critical aspect of chemistry that streamlines the handling and understanding of a vast array of compounds. Effective organization enables researchers to quickly identify, retrieve, and analyze molecules, enhancing workflow and promoting innovation in various fields like drug development, material science, and educational pedagogy. By implementing systematic methodologies, chemists can manage complex datasets and promote better data integrity, which ultimately supports advancements in both theoretical and applied chemistry.
Classification Schemes
Functional Groups
When considering functional groups, it's crucial to note their role as the backbone of organic chemistry. These groups dictate how organic molecules interact and react with one another, making them a cornerstone for classification systems. The key characteristic that sets functional groups apart is their reactivity patterns; they determine the chemical behavior of the entire molecule. By organizing compounds based on their functional groups, chemists can efficiently predict the outcome of reactions, thus simplifying the design of experiments.
For example, the distinction between alcohols and carboxylic acids hinges on their functional groups – hydroxyl (-OH) and carboxyl (-COOH), respectively. This functionality is not just a trifling detail; it plays a major role in defining the properties and behaviors of the molecules during reactions, reinforcing the notion that proper classification can yield significant insights.
However, while functional groups provide clarity, they can also introduce complications. Some molecules might contain multiple functional groups, complicating their classification and, consequently, their analysis. But overall, the functional group approach remains a widely appreciated methodology due to its clear relevance to chemical reactivity and synthesis.
Molecular Weight Distribution
In an entirely different vein, molecular weight distribution serves as another invaluable criterion for organizing organic compounds. Its significance lies in providing a quantitative way to understand the size of molecules, which is crucial for diverse applications, from pharmacology to polymer science. The ability to categorize molecules based on their molecular weight helps in predicting physical properties, biodegradability, and interactions with biological systems.
One notable aspect is the ability to utilize weight averages, such as number average and weight average molecular weights, to capture a clearer picture of a sample's distribution. This can reveal how a sample behaves in various environments, guiding researchers in material choices and enhancing the design of experiments. However, reliance solely on molecular weight can sometimes overlook critical structural details, thus requiring a balanced approach that considers both weight and structure.
Solubility Parameters
Solubility parameters are indispensable in the organization of organic molecules, especially when considering their environmental or biological context. This method encompasses several factors, such as polarity and hydrogen bonding capacity, to predict how molecules behave when dissolved in solvents. Importantly, solubility impacts reactions in real-world conditions, making this method particularly relevant for chemists involved in product development or natural resource management.
The unique feature of solubility parameters is their predictive nature; they can indicate which solvents will effectively dissolve a given compound without excessive trial and error. However, this simplicity is a double-edged sword; sometimes, exceptions can lead to unexpected results. Misjudgments regarding solubility can throw a wrench into experiments, which underscores the vital consideration for chemists to remain cautious and informed.
Electronic Structure Representation
The electronic structure representation is another major methodology for organizing organic molecules, focusing on the arrangement of electrons around nuclei. This methodology not only aids in theoretical predictions regarding molecular behavior but also plays a practical role in visualizing properties such as reactivity and stability. By employing various models, from Lewis structures to molecular orbitals, scientists can glean a different perspective on compounds that transcends mere elemental composition.
The melodic interplay of electrons shapes chemical bonding and reactivity, and an understanding of this structure enhances communication among scientists, helping standardize discourse. However, simplifications made during representations can sometimes lead to misconceptions if not carefully handled. Thus, maintaining a keen awareness of these methods' limitations is essential.
Overall, comprehensively organizing organic molecules is no simple task. Still, by employing unique methodologies – functional group classification, molecular weight distributions, solubility parameters, and electronic structure representation – chemists can navigate the complex landscape of organic compounds with greater ease and precision.
Applications of Organic Molecule Drawers
The applications of organic molecule drawers stretch far beyond mere organization. They serve as powerful tools in various fields, especially in pharmaceutical development and education. These drawers not only provide a systematic way to house organic compounds, but they also enhance collaboration, innovation, and understanding in chemical research and learning environments. Their significance cannot be overstated, as they are integral to simplifying complex chemical data while maximizing accessibility for students, researchers, and professionals alike.
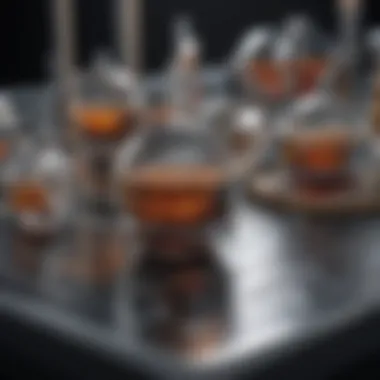
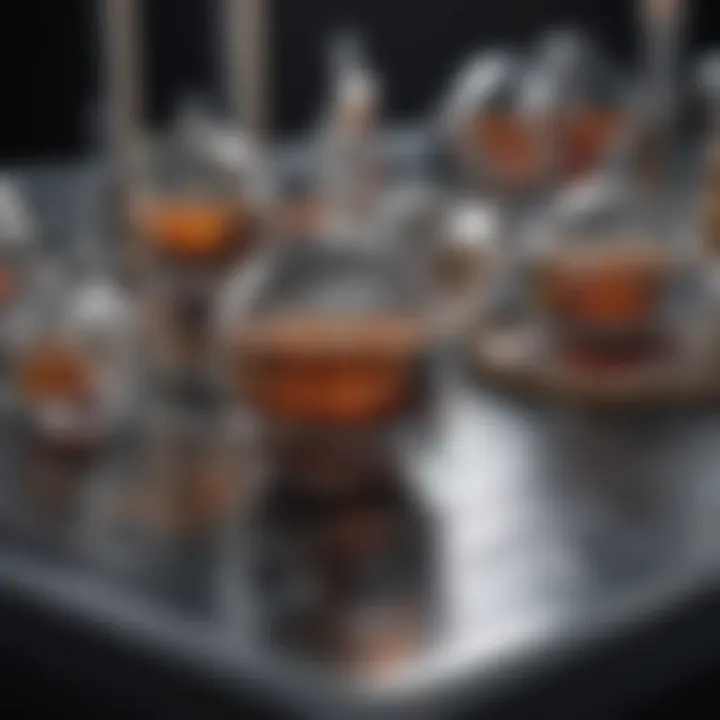
In Pharmaceutical Development
In the realm of pharmaceutical development, organic molecule drawers take center stage. They act as repositories that manage data about compounds that could potentially be turned into medications. When chemists are on the hunt for new drugs, accessing information about existing organic compounds can be critical. One immensely advantageous feature of these drawers is their ability to store detailed information on molecular structures, which can facilitate drug discovery and assist in predicting how new molecules might behave in biological systems.
- Compound Libraries: Researchers often use these drawers to create extensive libraries of compounds. This can aid in high-throughput screening, where numerous compounds can be tested quickly for biological activity.
- Data Management: With the complexity of modern data sets, an organic molecule drawer can integrate various types of data, from chemical properties to biological effects, facilitating better analysis and decision-making.
Thus, these applications lead to enhanced productivity in pharmaceutical research and a quicker path to drug development.
In Education and Learning
Teaching Tools and Resources
Teaching tools and resources centered around organic molecule drawers play a pivotal role in chemistry education. They provide students the means to visualize and interact with molecular structures, greatly hindering misconceptions that often arise from two-dimensional representations. These resources underscore the importance of hands-on learning.
A key characteristic of these teaching tools is their simulation abilities. Using software like ChemDraw or Avogadro, students can manipulate molecules in real-time, which provides a much richer educational experience. This immediacy of feedback helps students understand functional groups and molecular interactions more deeply.
The unique feature of using interactive software is its ability to integrate tutorials and quizzes, adding an element of gamification to learning chemistry. This approach makes the learning process feel less daunting and more enjoyable.
However, while these tools can enhance engagement, one drawback is the potential steep learning curve for certain software, which might confuse or even frustrate novice learners.
Interactive Learning Environments
Interactive learning environments built around organic molecule drawers also transform how chemical education is delivered. Platforms that allow collaborative work and real-time data sharing among students are becoming increasingly popular. They enable users to work together in constructing molecular models and brainstorming solutions to complex chemical problems.
The key characteristic of these environments is interactivity. By utilizing virtual labs or online collaboration tools, students can experience a dynamic learning atmosphere that encourages participation and discussion.
An essential unique feature is that these platforms often incorporate forums for discussion and peer feedback. This can lead to a deeper comprehension of material as students share diverse perspectives and insights. The collaborative nature replicates real-world scientific teamwork, preparing students for future careers in research and industry.
However, a consideration here is the reliance on technology, which can become a drawback in settings with limited resources or where accessibility is a concern.
"The essence of mastering organic chemistry lies not just in textbooks but in the tools and environments that engage the learner with the material."
"The essence of mastering organic chemistry lies not just in textbooks but in the tools and environments that engage the learner with the material."
In summary, the application of organic molecule drawers in both pharmaceutical settings and educational contexts speaks volumes about their importance. They not only streamline processes but also enrich learning experiences, thereby reshaping how organic chemistry is approached in both research and education.
Technological Advancements in Molecule Management
In the dynamic field of organic chemistry, the progress brought by technological advancements in molecule management cannot be overstated. These innovations serve as a lifeline for scientists, educators, and industry professionals alike, enabling more efficient practices and enhancing productivity across various tasks. Understanding these developments is essential, as they play a crucial role in shaping research, fueling discoveries, and optimizing educational methodologies.
Software Solutions and Platforms
A central player in the landscape of molecule management is sophisticated software solutions. These platforms often act as virtual laboratories for chemists, allowing for virtual modeling and simulation. For instance, tools like ChemSketch and Accelrys Discovery Studio provide users with the capability to draw molecular structures, predict their behaviors, and explore interactions with other compounds. Such software can be life-savers for researchers working under pressing timelines, effectively reducing the need for extensive lab work that can be both time-consuming and costly.
Moreover, the integration of artificial intelligence (AI) in these platforms has paved the way for predictive analysis in organic chemistry. AI algorithms can analyze large datasets quickly, enabling researchers to identify patterns that might not be immediately obvious. This could potentially lead to groundbreaking discoveries or the development of new compounds with specific desired properties, thereby accelerating the research process.
- Benefits of Software Solutions:
- Enhanced accuracy in molecular modeling
- Reduction in experimental costs
- Increased collaboration opportunities
- Streamlined data analysis processes
Data Sharing and Collaboration Tools
As the saying goes, "Sharing is caring"; in the realm of scientific research, it’s also about advancing knowledge. Effective data sharing and collaboration tools have become indispensable in modern organic chemistry. Platforms like GitHub and ResearchGate allow researchers across the globe to share findings and collaborate on projects seamlessly.
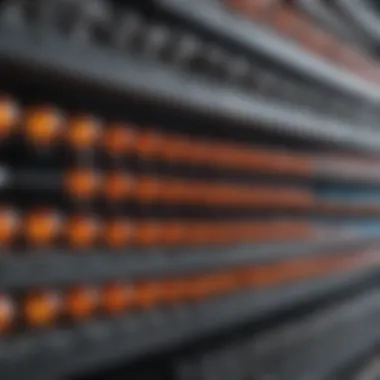
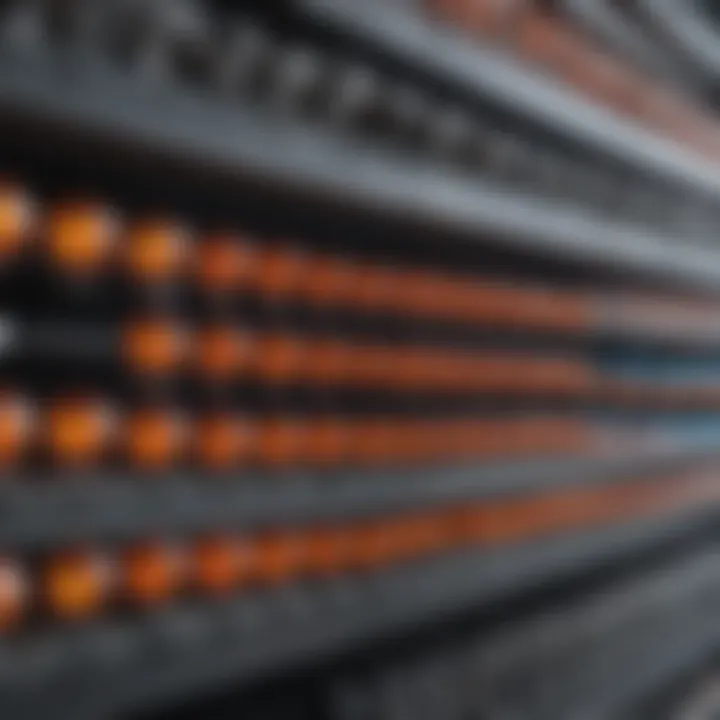
Attention to detail is paramount in chemical research, and collaborative tools help maintain this by ensuring that valuable insights and findings are easily accessible. For example, cloud-based platforms not only allow scientists to store data but also to work on shared projects in real-time, efficiently pooling expertise from various fields. An example is the ChemSpider database, which facilitates the sharing of compound information among scientists and students, creating an interconnected network of knowledge.
Effective collaboration enhances the ability of researchers to tackle complex challenges, leading to significant advancements in organic chemistry.
Effective collaboration enhances the ability of researchers to tackle complex challenges, leading to significant advancements in organic chemistry.
- Key Features of Collaboration Tools:
- Real-time updates on shared projects
- Comprehensive data storage solutions
- Enhanced communication channels for teams
- Easier integration of various research inputs
The intersection of technology and chemistry continues to blur boundaries and redefine approaches within the discipline. As professionals tap into these advancements, the future of organic molecule management stands bright, ready to unlock new levels of understanding and innovation in the field.
The Future of Organic Molecule Management
As we gaze into the crystal ball of organic molecule management, it becomes clear that the integration of advanced technologies and sustainable practices will dictate how we handle and study organic compounds. Organic chemistry is more than a scientific discipline; it’s a foundational pillar that supports various industries, from pharmaceuticals to environmental science. With the inflow of new techniques and tools, understanding their implications becomes crucial for students, researchers, educators, and industry professionals alike.
Integrating AI in Organic Chemistry
The march of artificial intelligence into the realm of organic chemistry is not just a trend—it’s a revolution. AI has begun to transform how chemists predict molecular behaviors, design complex compounds, and analyze vast datasets. For instance, machine learning algorithms can process patterns in molecular structure that would take a human considerable time to discern. These systems are proving invaluable in the drug discovery process, where speed and precision are paramount.
- Benefits of AI Integration:
- Predictive Modeling: Machine learning can predict how different molecules will interact, fundamentally enhancing our understanding of chemical reactions.
- Data Management: AI simplifies data organization, helping researchers navigate large chemical databases efficiently, thereby saving time and reducing the potential for human error.
- Automated Synthesis Planning: With tools like ChemBERT, chemists can have AI suggest synthetic pathways for complex molecules, allowing for innovative solutions that push the boundaries of traditional synthetic methods.
"As we harness AI, the potential for accelerated discovery in organic chemistry is boundless, paving paths to breakthroughs we’ve only dreamed of."
"As we harness AI, the potential for accelerated discovery in organic chemistry is boundless, paving paths to breakthroughs we’ve only dreamed of."
The ongoing dialogue about AI in this space emphasizes the importance of collaboration between chemists and computer scientists, enhancing the collective knowledge base and leading to more robust chemical research.
Sustainability in Chemical Research
Sustainability has emerged as a recurring theme in all scientific fields, and organic chemistry is no exception. The future of chemical research mandates that we focus increasingly on eco-friendly practices. This means developing methods that minimize waste and lessening our environmental footprint, particularly when synthesizing organic compounds.
Several key considerations illustrate the importance of sustainability:
- Green Chemistry Principles: Adopting principles such as waste prevention, resource efficiency, and renewable feedstocks not only conserves the environment but also improves the overall efficiency of chemical processes.
- Biodegradable Chemicals: More researchers are investigating natural compounds that offer a lower environmental impact—think of greener solvents and catalysts that perform efficiently without adverse effects on ecosystems.
- Circular Economy in Chemistry: This approach promotes recycling of materials, reducing the need for new raw materials. Practical implications include using waste as feedstock for new chemical processes, tapping into the idea that one chemist’s waste might just be another’s valuable resource.
By steering research toward these sustainable practices, the field of organic chemistry not only aligns itself with global sustainability efforts but also enhances its societal relevance. The commitment to sustainable practices can serve as a guide for future research directions, ensuring that chemistry contributes positively to the world.
In summary, the future of organic molecule management is bright, characterized by AI's innovative capabilities and a strong emphasis on sustainable practices. Both realms demand a solid understanding from current and future chemists, illustrating the need for education and adaptation in a rapidly evolving field.
Culmination
The exploration of organic molecule drawers uncovers their critical role in the landscape of chemistry today. This concept serves not just as a means of organization but as a bridge between theory and practice in scientific inquiry. By efficiently categorizing and managing organic compounds, these tools streamline the research process, making complex molecular data more accessible to students, educators, and researchers alike.
Summary of Key Insights
Throughout this article, several key insights emerged about the organic molecule drawer. First and foremost, it is evident that:
- Organization: A well-structured molecule drawer enhances the ease of access to vital information regarding organic compounds.
- Digital Tools: The integration of digital platforms has revolutionized the traditional methods of molecule organization, paving the way for real-time collaboration among scientists and contributing to a more interactive learning environment.
- Multifaceted Applications: Beyond research, these drawers have significant implications in education, simplifying complex topics through the use of practical examples and hands-on activities.
In essence, the organic molecule drawer encapsulates the multifaceted nature of chemistry—bridging gaps between various fields while promoting a deeper understanding of molecular interactions.
Future Directions for Research
Looking ahead, several future directions warrant attention in the realm of organic molecule management:
- AI Integration: As artificial intelligence continues to advance, there lies immense potential to enhance molecule drawers with intelligent algorithms that could offer predictive insights into molecular behavior.
- Sustainability Initiatives: Research should also consider sustainable practices in chemical management, focusing on reducing waste and optimizing resource use, aligning with global sustainability goals.
- Interdisciplinary Collaboration: Promoting collaboration among various scientific fields will enrich the utility of organic molecule drawers, tapping into innovative methodologies and applications.
By following these paths, researchers can progress toward building a more comprehensive and efficient future for organic chemistry, ensuring that the organic molecule drawer remains a cornerstone of scientific exploration.