Exploring Western Blotting Techniques and Innovations
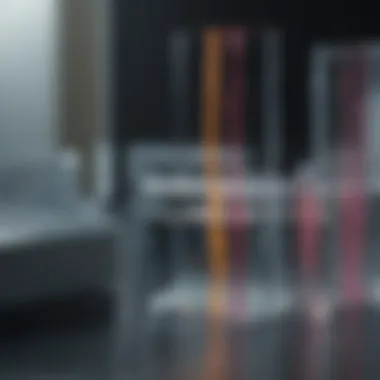
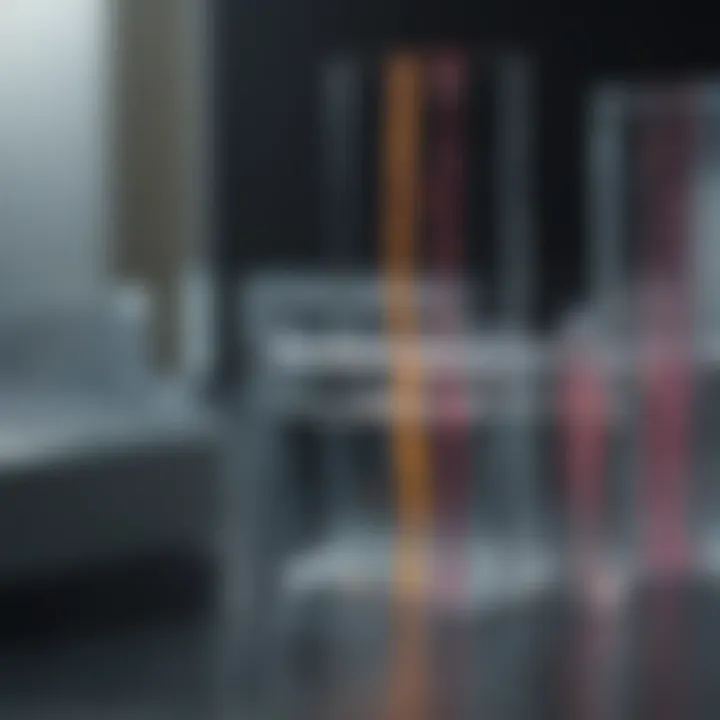
Article Overview
Western blotting stands as a cornerstone technique in biochemical and molecular biology studies. As a method tailored for protein detection and analysis, it serves not only in academic labs but also in clinical environments, showcasing its varied applications across multiple disciplines. This comprehensive examination aims to provide a clear understanding of the overall methodology, the challenges faced, and the innovations being introduced into the realm of western blotting.
Purpose of the Article
The primary focus of this article is to scrutinize the intricate details of western blotting, elucidating each step, from sample preparation to detection. Readers will find a thorough exploration of both traditional methods and the latest advancements, such as enhanced imaging techniques. By digging into the nitty-gritty of the process, the article seeks to equip students, researchers, and professionals with knowledge that can improve their experimentation and troubleshooting skills in protein analysis.
Relevance to Multiple Disciplines
Western blotting is not confined to a single field but stretches its wings over various scientific disciplines. From proteomics to diagnostics in medical research, the insights provided through western blotting can lead to significant breakthroughs. Its importance in understanding diseases, such as cancer and infections, makes it invaluable not just for researchers but also for those involved in developing therapeutic strategies.
Research Background
A comprehensive grasp of western blotting requires an appreciation of its historical evolution and core concepts.
Historical Context
The inception of western blotting traces back to the late 1970s, credited largely to the pioneering efforts of W. Keith Wallace. Originally developed as a method to detect specific proteins in a complex mixture, western blotting has drastically evolved across the decades, refining its specificity, sensitivity, and overall efficiency. As research progressed, so did techniques, with enhancements such as membrane technology and the advent of enhanced chemiluminescence (ECL).
Key Concepts and Definitions
For anyone approaching this technique, understanding some fundamental terms is crucial:
- Electrophoresis: A method used to separate proteins based on their size and charge, crucial in the early step of western blotting.
- Transfer: This phase involves moving proteins from the gel to a membrane, usually made from nitrocellulose or PVDF.
- Blocking: A process to prevent non-specific binding, which can lead to background noise in the detection phase.
By delving into the background and key concepts related to western blotting, readers will better appreciate the complexity and significance of this widely used laboratory technique.
Preface to Western Blotting
Western blotting is more than just a laboratory technique; it serves as a cornerstone of proteomics and molecular biology. This advanced method enables researchers to detect and analyze specific proteins in a mixture, offering invaluable insights for a wide array of applications. Understanding the principles and procedures involved in Western blotting not only aids in data accuracy but also enhances the quality of research outcomes.
Historical Context
The origins of Western blotting trace back to the 1970s when researchers sought effective methods to identify proteins amongst a complex mixture. This technique was first introduced by Stephen M. Daugherty in the context of immunology research. Eventually, it gained traction in various fields such as biochemistry and cellular biology. The juxtaposition of its straightforwardness and versatility led to widespread adoption, making it a staple in laboratories worldwide. As a historical note, the term ‘Western blot’ was coined by Gary W. Blume as a playful reference to the ‘Southern blot’ method invented by Edwin Southern, which was aimed at detecting specific DNA sequences. This whimsical naming convention marked a shift in the landscape of molecular biology techniques, highlighting innovation in detection methods.
Definition and Purpose
In essence, the Western blot is a technique that permits the visualization of specific proteins within a sample. The primary purpose revolves around protein detection and quantification, allowing researchers to understand protein expression levels, modifications, and interactions. This is critical, as variations in protein levels can indicate physiological or pathological conditions. For instance, elevation or reduction in certain proteins often serves as biomarkers for diseases like cancer or infectious pathogens. By systematically isolating proteins, researchers can not only confirm hypotheses but also generate insights that drive scientific discovery.
Utilizing this method involves several steps including protein separation, transfer to a membrane, and detection using antibodies.
In summary, grasping the foundations of Western blotting equips students, researchers, and professionals with the knowledge necessary to explore deeper biological questions and contribute to advancements in science.
"Understanding the components and processes of Western blotting can illuminate pathways in both basic and applied research."
"Understanding the components and processes of Western blotting can illuminate pathways in both basic and applied research."
In the following sections, we will delve into the principles, methodologies, and applications that characterize this essential technique.
Principles of Western Blotting
Western blotting holds a prominent place in molecular biology and biochemistry, allowing researchers to analyze proteins in their samples. Understanding the fundamental principles that guide this technique is crucial, as it encompasses various components, such as protein separation and transfer methods. These principles not only outline the methodology but also pave the way for applications in clinical diagnostics and research studies.
Protein Separation Techniques
SDS-PAGE
SDS-PAGE, short for Sodium Dodecyl Sulfate Polyacrylamide Gel Electrophoresis, is one of the cornerstones of Western blotting. This technique utilizes an electrical field to separate proteins based on their molecular weight. SDS, an anionic detergent, denatures proteins and imparts a negative charge, allowing them to migrate through a polyacrylamide gel matrix. The size of proteins dictates their migration speed—smaller proteins traverse the gel more quickly than larger ones.
One of the compelling characteristics of SDS-PAGE is its efficiency in resolving proteins with high precision. This feature makes SDS-PAGE a go-to method for numerous labs. However, keep in mind that SDS-PAGE cannot accurately separate proteins with very similar molecular weights, leading to potential identification issues.
Key Advantages of SDS-PAGE:
- High resolution of protein bands
- Suitable for a broad range of protein sizes
- Simple and relatively quick procedures
Disadvantages of SDS-PAGE:
- Lacks the ability to maintain protein function
- Requires thorough optimization of gel concentration for specific applications
Isoelectric Focusing
Isoelectric focusing is another pivotal protein separation technique used in Western blotting. This method relies on the principle of a protein's isoelectric point (pI) - the point at which the net charge of that protein is zero. By applying a pH gradient within the gel, proteins will migrate to a certain point corresponding to their pI during electrophoresis.
The essential asset of isoelectric focusing is its exceptional capacity to separate proteins that have similar molecular weights but different pIs. This improves the resolution of complex protein mixtures often encountered in biological samples.
Key Features of Isoelectric Focusing:
- Excellent resolution for proteins with similar weights
- Maintains protein functionalities for downstream applications
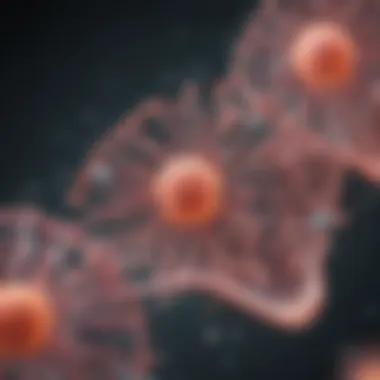
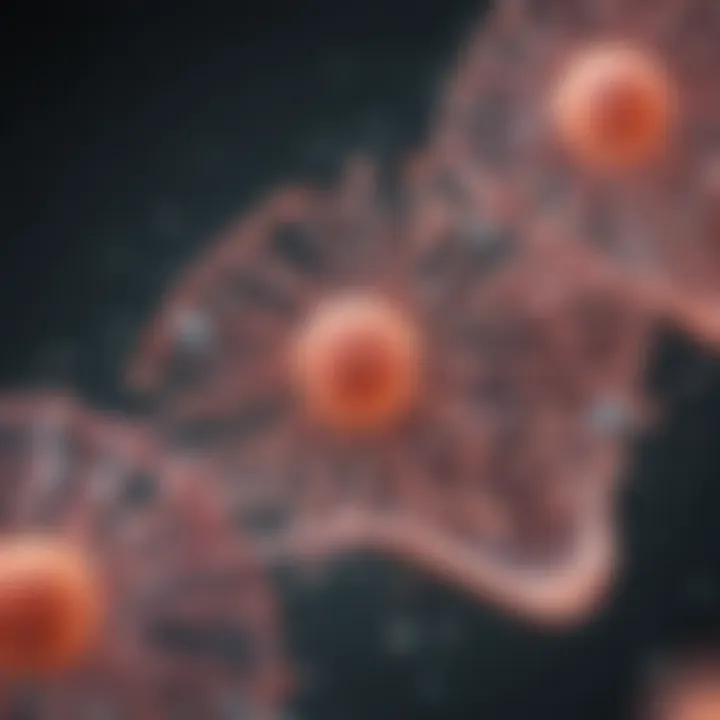
Advantages and Disadvantages:
- Can be time-consuming due to pH gradient establishment
- Specialized equipment required for optimal results
Transfer Methods
Electroblotting
Capable of quickly transferring proteins from a gel to a solid support, electroblotting is a hallmark procedure in Western blotting. By applying an electric field, proteins are driven out of the gel and onto a membrane, typically made of nitrocellulose or PVDF. The beauty of this process lies in its efficiency; it takes only a matter of hours to achieve effective protein transfer, a stark contrast to earlier methods like diffusion transfer, which can take much longer.
Electroblotting is favored for its rapidity and adaptability. The method can be tailored to suit various protein types and sizes, making it a flexible choice for diverse experiments.
Key Characteristics of Electroblotting:
- Fast transfer of proteins
- Highly effective for low-abundance proteins
Advantages and Disadvantages:
- Requires specialized equipment for execution
- Some risk of protein loss due to excessive voltage
Nitrocellulose Membranes
Nitrocellulose membranes have been a staple in Western blotting since the method's inception. These membranes possess a high protein-binding capacity, which effectively traps proteins post-transfer, allowing for reliable detection.
The distinctive feature of nitrocellulose membranes is their compatibility with various detection methods. This robust nature means they can be used with both chemiluminescence and colorimetric techniques, making them a versatile option in a lab setting.
Key Characteristics of Nitrocellulose Membranes:
- High binding capacity for a wide range of proteins
- Compatible with multiple detection methods
Advantages and Disadvantages:
- May autofluoresce, which can interfere with some detection methods
- Limited reusability depending on the complexity of the sample
Effective Western blotting demands a solid understanding of its principles, making protein separation and transfer methods vital for successful experimentation.
Effective Western blotting demands a solid understanding of its principles, making protein separation and transfer methods vital for successful experimentation.
Reagents and Materials in Western Blotting
Reagents and materials play a crucial role in the accuracy and efficiency of western blotting. They are the backbone that supports every step of the process, from protein separation to detection. The selection of high-quality reagents ensures reproducibility and reliability, which are essential in both clinical and research settings. Understanding the specific elements involved allows researchers to tailor their approaches to suit the requirements of their experiments.
Antibodies
Antibodies are among the most vital reagents in western blotting, serving as the bridge between the proteins of interest and the detection methods used to visualize them. They come in two major forms: primary and secondary antibodies, each with its unique purpose in the experiment.
Primary Antibodies
Primary antibodies bind directly to the target protein in the sample. One key characteristic of primary antibodies is their specificity; they are designed to recognize and attach to a particular epitope on the protein. This selectivity is beneficial because it increases the accuracy of the detection. Researchers have a vast array of primary antibodies to choose from, tailored to various proteins across different species.
A unique feature of primary antibodies is their availability in different formats, including monoclonal and polyclonal types. Monoclonal antibodies are produced by identical immune cells, providing consistency and uniformity across experiments. In contrast, polyclonal antibodies combine multiple antibodies derived from different immune cells, giving a broader recognition of epitopes. However, the latter can sometimes lead to variability in results due to batch differences.
Some advantages of using primary antibodies include:
- High specificity for the target protein.
- Variety of sources and types available.
- The ability to detect various post-translational modifications.
On the downside, finding the right primary antibody can sometimes be challenging due to extensive catalog options, and the necessity for optimization in dilution and incubation times can be a hassle.
Secondary Antibodies
Secondary antibodies complement the role of primary antibodies. These are designed to bind to the primary antibodies and often carry a reporter enzyme or a fluorescent tag that facilitates the detection process. One of the key characteristics is their ability to amplify the signal from the primary antibodies, thus enhancing visualization of the target protein. They are typically derived from a different species than the primary antibody, which allows for the formation of a stable bond without cross-reactivity.
A unique feature of secondary antibodies is their versatility; researchers can choose enzymes like horseradish peroxidase or alkaline phosphatase, depending on the detection method they plan to use, whether it be chemiluminescent or colorimetric. This versatility provides flexibility in experimental design.
Advantages of incorporating secondary antibodies into an experiment include:
- Increased signal amplification, improving detection sensitivity.
- Capability for multiplexing, which allows the simultaneous detection of multiple proteins.
- More economical because one secondary antibody can be used with various primary antibodies.
However, they can also introduce complexities, such as the need for additional optimization steps to determine the appropriate dilution and incubation times. Moreover, the potential for non-specific binding can complicate the results.
Buffers and Solutions
The buffers and solutions used in western blotting are key players that facilitate the interaction between proteins and antibodies, maintain pH levels, and ensure proper conditions for protein stability and mobility during electrophoresis. For instance, Tris-Glycine buffer is a commonly used buffer for electrophoresis because it provides good conductivity and stability. It's essential to use the right buffer concentrations to prevent unwanted reactions or degradation of proteins.
Additionally, blocking solutions made from BSA or non-fat dry milk are used to reduce non-specific binding during the antibody incubation steps. The effectiveness of these solutions is paramount for obtaining clean results, without background noise that could cloud the interpretation of data.
Buffing solutions also help regulate ionic strength and pH, which can influence protein conformation and interaction with antibodies, further emphasizing their importance in achieving reliable outcomes in western blotting.
In summary, the right selection and application of reagents and materials not only streamline the entire process of western blotting but also enhance the accuracy and reliability of the results obtained, making them indispensable for anyone venturing into this essential technique in molecular biology.
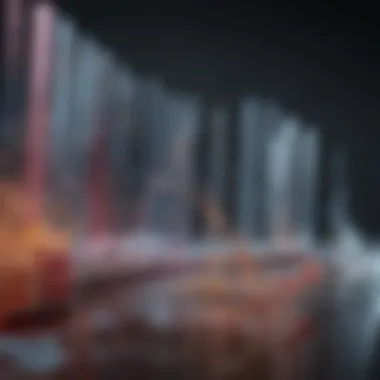
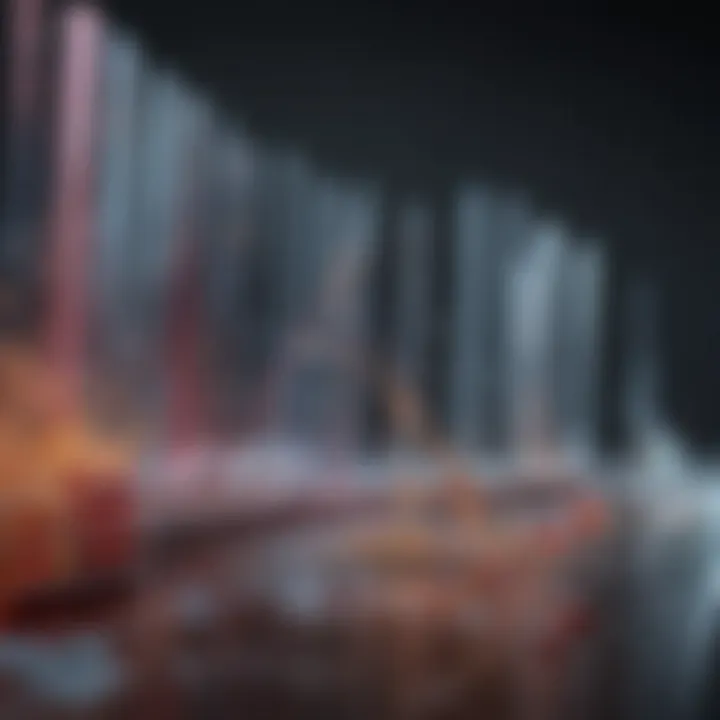
Step-by-Step Protocol
The protocol for western blotting is pivotal, serving as a detailed guide that can make or break the success of this intricate technique. Each step in the process must be followed meticulously, not only to ensure accurate results but also to maintain the integrity of the samples. A sound protocol allows researchers to replicate their findings, which is the cornerstone of scientific validation. Additionally, understanding this step-by-step approach arms one with the knowledge to troubleshoot any issues that may pop up along the way.
Sample Preparation
Sample preparation is the first crucial step in western blotting. It involves extracting proteins from biological samples, which could range from cell cultures to tissue homogenates. Getting the sample right can be likened to setting a solid foundation before constructing a building. An inadequate or improper sample could lead to misleading results. Key considerations include the choice of lysis buffer, which may contain detergents and protease inhibitors to preserve protein integrity. The choice of method for cell lysis can also impact the yield and quality of the proteins extracted.
It’s important to maintain the samples on ice during preparation to prevent protein degradation. Once lysed, samples often require centrifugation to remove cellular debris, thus ensuring a cleaner solution for subsequent steps.
Gel Electrophoresis
Following sample preparation is gel electrophoresis, where proteins are separated based on their size. This step is crucial because it allows for the resolution of proteins within the sample, making it possible to analyze their properties later on. Sodium dodecyl sulfate-polyacrylamide gel electrophoresis (SDS-PAGE) is predominantly used due to its efficacy in denaturing proteins and providing uniformity in charge-to-mass ratio.
During electrophoresis, an electric current passes through the gel, which causes proteins to migrate towards the positive electrode. Small proteins tend to move faster compared to larger ones, thus creating a distinct separation band. Paying attention to the gel concentration based on the size of the target proteins is essential as well—too high a concentration may lead to resolution issues, while too low can lead to poor separation.
Blotting Technique
The blotting phase is where proteins, now separated through gel electrophoresis, are transferred to a solid membrane, typically made from nitrocellulose or PVDF (polyvinylidene fluoride). This is a delicate process as it preserves the spatial arrangement of the proteins while providing a suitable substrate for subsequent antibody binding. Electroblotting is commonly employed, wherein an electric field drives proteins from the gel onto the membrane.
The transfer efficiency is paramount; proteins that fail to transfer well can result in faint or absent bands in the final analysis. After transferring, membranes undergo blocking to prevent non-specific binding, ensuring that any signals detected can be attributed only to the target proteins.
Detection Methods
Once blotting is successfully completed, detection methods bridge the analysis with observable results. There are various approaches, but two prominent methods include chemiluminescence and colorimetric detection. Each method carries unique features and serves differing purposes within the context of western blotting.
Chemiluminescence
Chemiluminescence is a popular detection method for western blotting, mainly due to its sensitivity. In this technique, enzymes linked to secondary antibodies catalyze a reaction to produce light, which can be captured on X-ray film or digital imaging systems. The key characteristic of chemiluminescence is its ability to detect even minor amounts of target proteins, making it ideal for applications where protein expression is low.
However, one should note that chemiluminescence generally offers a relatively short window for imaging post-reaction, as the emitted light fades quickly. Thus, it requires precise timing in capturing images, which, while an added layer of complexity, greatly enhances the specificity of results.
Colorimetric
Colorimetric detection presents another approach for analyzing proteins on membranes. This method relies on enzyme-catalyzed reactions that create colored products; the intensity of the color produced is proportional to the amount of the target protein present.
A notable advantage of colorimetric methods is their simplicity and the immediate visual results they provide, allowing for straightforward interpretation. However, the sensitivity of colorimetric detection often doesn’t match that of chemiluminescence, making it more suitable for certain applications where protein quantities are higher.
Ultimately, the choice between chemiluminescence and colorimetric detection boils down to the specific needs of the experiment and the expected abundance of the target proteins. Understanding both methods provides researchers with flexible options for achieving their analysis goals.
Applications of Western Blotting
Western blotting serves as a cornerstone in protein analysis and detection, wielding significant implications across multiple scientific domains. This technique's versatility makes it invaluable, particularly when delving into the realms of clinical diagnostics and advanced research. Not only is it pivotal for confirming the presence of specific proteins, but it also throws light on their functional roles within various biological processes. Understanding these applications offers a profound appreciation of western blotting’s role in both healthcare and science.
Clinical Diagnostics
In the clinical sphere, western blotting plays a critical role in diagnosing diseases, particularly infectious diseases and certain cancers. The technique helps detect disease-specific proteins, serving as a reliable marker for conditions like HIV, where it confirms the presence of antibodies that indicate infection. This method stands out for its specificity and sensitivity, allowing healthcare professionals to differentiate between positive and negative cases with impressive accuracy.
A notable aspect of clinical diagnostics using western blotting is its ability to provide results that often are not apparent through other testing methods. For instance, in the case of Lyme disease, the presence of specific antibodies against the bacterium can be verified justly. Yet, healthcare providers must consider that the interpretation of results can be complex due to potential cross-reactivity with proteins from other conditions.
Research Applications
Research applications of western blotting are extensive, spanning various areas of scientific inquiry. From studying the cellular mechanisms involved in cancer to understanding the immune response in infections, the contributions of this technique are profound.
Cancer Research
Cancer research encapsulates a specific realm where western blotting shines as a popular and beneficial methodology. The main reason its favored is its ability to elucidate the expression levels of oncogenes and tumor suppressor proteins. For example, variations in the levels of p53 protein can be assessed, which plays a crucial role in cancer prevention. This insight into protein activity assists researchers in understanding tumorigenesis and identifying potential biomarkers for early detection.
A unique feature of cancer research utilizing western blotting is its capacity to provide quantitative data. Researchers can compare expression levels in cancerous versus non-cancerous tissues, promoting a clearer understanding of malignancy progression. However, it’s important to mention that while western blotting is an effective tool, it can sometimes lead to false positives, necessitating corroborative analyses with other techniques to ensure reliability.
Immunology Studies
Equally relevant is the application of western blotting in immunology studies. In this field, the technique is instrumental for detecting specific antibodies produced in response to pathogens or vaccines. A common practice includes evaluating the antibody response in human samples post-vaccination, which is crucial for assessing vaccine efficacy.
What makes immunology studies particularly appealing in conjunction with western blotting is its robust method for distinguishing between similar antibody isotypes. Researchers can analyze different classes of antibodies to get insights into immune responses at various stages, enhancing our understanding of immunological behaviors. Still, like cancer research, this area faces challenges; the presence of non-specific binding can complicate results, requiring meticulous attention to experimental design.
In summary, the applications of western blotting extend from healthcare diagnostics to essential research. Understanding these facets is pivotal for leveraging its full potential in both clinical and experimental settings.
Challenges in Western Blotting
Western blotting is not just a straightforward technique, it comes with its own set of challenges that researchers must navigate to ensure accurate results. Understanding these challenges is pivotal because even minor blunders can lead to misinterpretations and disastrous conclusions in research. Acknowledging the roadblocks can lead to improved techniques and enhanced outcomes. Here, we dive into some of the prominent challenges, with a keen focus on specific errors commonly encountered and the issue of contamination, which can significantly compromise the integrity of results.
Common Errors
In the world of western blotting, making errors is like a rite of passage for many scientists. Such mistakes can stem from a multitude of sources, which makes it essential to be aware of them. Here are several common errors that can flummox even seasoned investigators:
- Improper sample preparation: Not all samples are created equal. Failing to adequately break down tissues or lysing cells efficiently can result in insufficient protein extraction. This leads to faint bands, giving the impression that a target protein is absent.
- Gel electrophoresis mistakes: From loading the sample improperly to running the gel at the wrong voltage, many factors can skew the separation of proteins. Additionally, using old or expired reagents can cause variability in results.
- Antibody specificity issues: Sometimes, the antibodies used can be a source of frustration. Non-specific binding of antibodies can create unexpected bands during detection, causing confusion about the results.
"It's crucial to double-check your workflow and maintain high standards in every step, as overlooking even the tiniest detail can lead to significant problems."
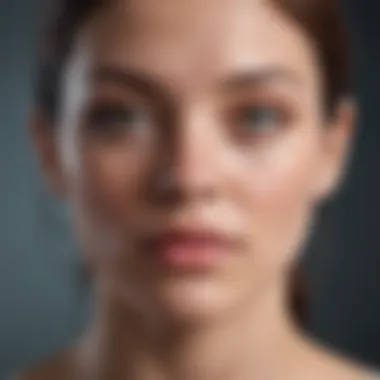
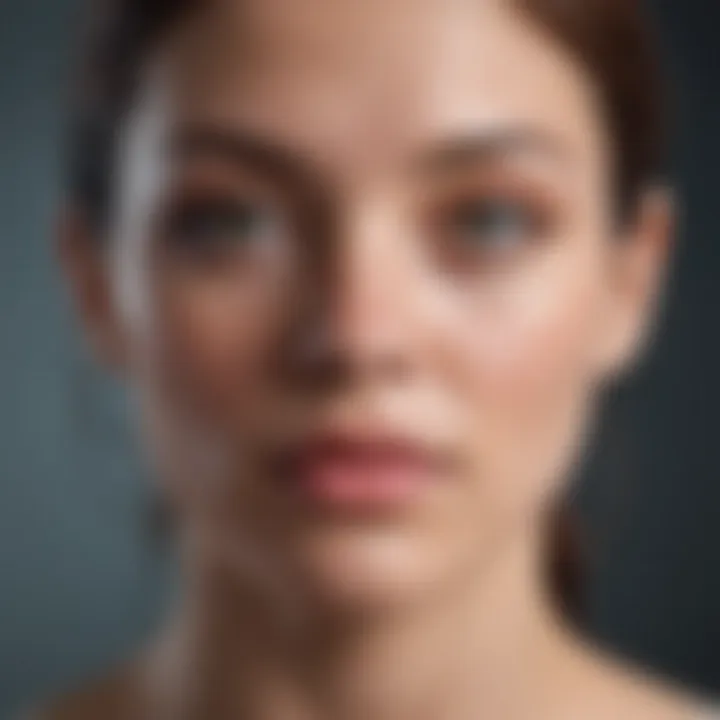
"It's crucial to double-check your workflow and maintain high standards in every step, as overlooking even the tiniest detail can lead to significant problems."
Contamination Issues
Contamination is the unwelcome guest at the party of western blotting. It can creep in without warning and wreak havoc on results. Various sources can introduce contaminants during different stages of the process:
- Reagency contamination: This can come from cross-contamination among reagents, especially in shared lab environments. For instance, having multiple people working with shared antibody stocks may lead to the introduction of unwanted proteins, skewing your data.
- Surface contamination: The surfaces of gel boxes, pipettes, and other lab equipment can harbor foreign proteins or nucleic acids. A simple wipe down with non-sterile paper towels can disrupt the results.
- Airborne contaminants: In labs where sensitive work is conducted, even particles floating in the air can be problematic. Techniques employed to minimize these risks, such as using laminar flow hoods or filtered environment, are crucial.
Each of these challenges requires diligent attention and a methodical approach to mitigate the impact on results. By understanding common pitfalls and contamination issues, researchers can enhance the reliability of their findings and contribute meaningfully to scientific advancement.
Troubleshooting Western Blotting Results
Troubleshooting is a pivotal aspect of western blotting, as even subtle errors can lead to significant consequences in data interpretation. It’s crucial for researchers to have the skill set to identify problems swiftly to ensure that the results are reliable and interpretable. Many factors come into play when analyzing western blot results, and knowing how to navigate these challenges can drastically enhance the validity of the findings.
A common area where issues arise is during the examination of faint bands. These can signal that something in the workflow might have faltered, and it’s essential to dissect these occurrences rather than dismiss them.
Analyzing Faint Bands
Faint bands can be quite the mystery in a western blot. It’s often perplexing when one doesn’t see strong signals, but fret not; there are numerous reasons this can occur. Here are some considerations:
- Antibody Concentration: Sometimes, the concentration of the antibody used may be too low. Increasing the antibody concentration or the incubation time can improve visibility.
- Protein Loading: Ensure that equal amounts of protein are loaded in each well. If the load is too low, you could end up seeing faint bands.
- Transfer Efficiency: Poor transfer efficiency from the gel to the membrane can also lead to weak signals. Make sure the transfer conditions, such as voltage and time, are optimal.
- Detection reagents: The reagents used in the detection phase may be expired or have been improperly stored, resulting in decreased sensitivity.
After making adjustments, if you observe improvements, that’s a good indication you’ve pinpointed the issue. Always remember to keep a close record of every adjustment — it’s all part of the troubleshooting journey.
"Understanding faint bands is like solving a puzzle; each piece must fit perfectly to reveal the complete picture."
"Understanding faint bands is like solving a puzzle; each piece must fit perfectly to reveal the complete picture."
Non-Specific Binding Solutions
Non-specific binding can muddy the waters of your western blot results, leading to noise that can be misinterpreted as signals. When proteins stick where they shouldn’t, it complicates the analysis significantly. Here are some effective strategies to mitigate this problem:
- Blocking Agents: Use appropriate blocking agents, such as BSA or milk, to cover potential binding sites on the membrane. This step is non-negotiable.
- Dilution of Antibodies: Sometimes, less is more, particularly with antibodies. Diluting the primary and secondary antibodies can help minimize non-specific interactions.
- Washing Steps: Incorporate adequate washing steps between the antibody incubations. This ensures that any loosely bound antibodies are washed away, reducing background noise.
- Temperature Control: Conducting incubations at lower temperatures can also help, as it tends to reduce the chance of non-specific binding.
By addressing non-specific binding effectively, researchers can reveal clearer results, yielding a more accurate understanding of their experiments. The emphasis here is on refining the process to yield confident results.
Advancements in Western Blotting
Western blotting, a venerable technique in molecular biology, has seen substantial improvements over the years. These advancements not only enhance its precision in protein detection but also broaden its applicability across various scientific domains. Innovations in this field reflect the ongoing pursuit for increased efficiency, accuracy, and throughput, essential for addressing the complex queries lodged in today's biomolecular research landscape.
Automated Western Blotting
Automation brings a breath of fresh air to western blotting procedures, lifting some of the heavy lifting off researchers. Manual methods can be time-consuming and subject to human error. In contrast, automated systems streamline many steps of the process, including sample loading, incubation, and washing. For instance, systems such as the Bio-Rad VersaDoc or the GE Healthcare's automated blotting systems have emerged, allowing for a standardized workflow that ensures consistency.
The key benefits of automation include:
- Enhanced Reproducibility: By minimizing the variability that can come from manual handling, robotic systems produce more reliable results.
- Time Efficiency: Automating repetitive tasks lets researchers focus on data analysis and interpretation rather than spending hours on procedural details.
- Scalability: As demands for research increase, the ability to run multiple samples simultaneously becomes increasingly valuable.
Considerations when utilizing automation are also important. Equipment costs can be significant, and there's a learning curve associated with integrating these systems into existing lab practices. However, for many, the upsurge in productivity may well justify those initial investments.
High-Throughput Techniques
High-throughput techniques are designed to maximize the number of samples processed in a shorter time, a crucial factor in fast-paced research settings. Implementing high-throughput western blotting means labs can analyze hundreds of samples in a single run.
The advantages of high-throughput methods are evident:
- Increased Data Generation: Researchers can collect a wealth of data more quickly which is vital in comparative studies and screening assays.
- Cost-Effective: By processing many samples at once, per-sample costs can be significantly reduced.
- Greater Experimental Flexibility: High-throughput systems often allow researchers to easily manipulate experimental conditions, facilitating novel exploration.
Some notable high-throughput approaches include the use of multiplexing techniques, where multiple proteins can be detected simultaneously. Products like Millipore’s multiplex assays allow scientists to gather more information with less material, which is particularly beneficial when dealing with scarce samples.
As with automation, there are drawbacks. Workflows may require careful optimization, and data analysis can become more complex with the increased sample size. Nevertheless, when executed properly, high-throughput techniques can propel research forward, offering insights that were previously unattainable due to scaling limitations.
"The evolution of western blotting reflects not just technological progress, but also our growing understanding of intricate biological processes."
"The evolution of western blotting reflects not just technological progress, but also our growing understanding of intricate biological processes."
Through these advancements, western blotting continues to adapt, reinforcing its significance in scientific exploration. As researchers harness these new methods, the potential for groundbreaking discoveries becomes more tangible.
Epilogue
In summarizing the discussion about western blotting, the technique emerges as a pivotal method for protein analysis, bridging gaps between initial discovery and practical application. Its reliability in detecting specific proteins makes it indispensable in fields like clinical diagnostics and research. The evolution of this technique over the years showcases significant technological advancements, enhancing its usability in various research environments.
Future Prospects for Western Blotting
Looking ahead, the future of western blotting holds promising potential. Innovations such as integration with mass spectrometry are on the rise, potentially offering even more precise identification of proteins in complex samples. For instance, the combination of these technologies could streamline workflows in laboratories, making them more efficient. Furthermore, as artificial intelligence continues to permeate science, algorithms designed to analyze blotting data might emerge, providing deeper insights far quicker than current methods.
In addition to technological enhancements, the consistency in improving protocols for better reproducibility is also an area of significant promise. As laboratories around the world standardize procedures to minimize variability, the overall reliability of western blotting results is likely to rise. This standardization can enhance the technique's acceptance and reliability across different scientific disciplines, ensuring that findings are robust and applicable worldwide.
The Importance of Continued Research
Continued research is vital for the ongoing advancement of western blotting techniques. New findings not only inform improvements on existing practices but also challenge scientists to rethink traditional methodologies. By fostering innovation through research, the scientific community can address current limitations in sensitivity, specificity, and throughput of the technique. Moreover, understanding the biological nuances behind protein behavior and interactions could refine the applications of western blotting, leading to breakthroughs in various fields including cancer research and immunology.
Ongoing investigations also allow for an improved comprehension of antibody specificity and optimization of reagents, which are critical for achieving accurate results. As more researchers push the boundaries of what is known, the lessons learned can lead to better reagents, enhanced automation techniques, or alternative methods that can complement western blotting.
"The future of scientific research hinges on the ability to adapt and refine methodologies to unveil the complexities of biological systems."
"The future of scientific research hinges on the ability to adapt and refine methodologies to unveil the complexities of biological systems."