Fiber Photometry and Calcium Imaging in Neuroscience
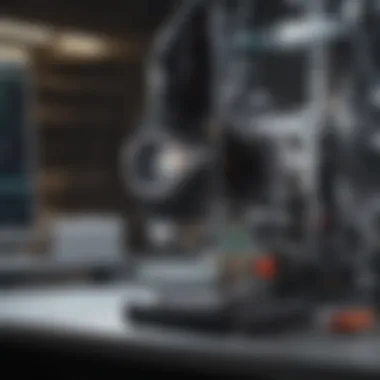
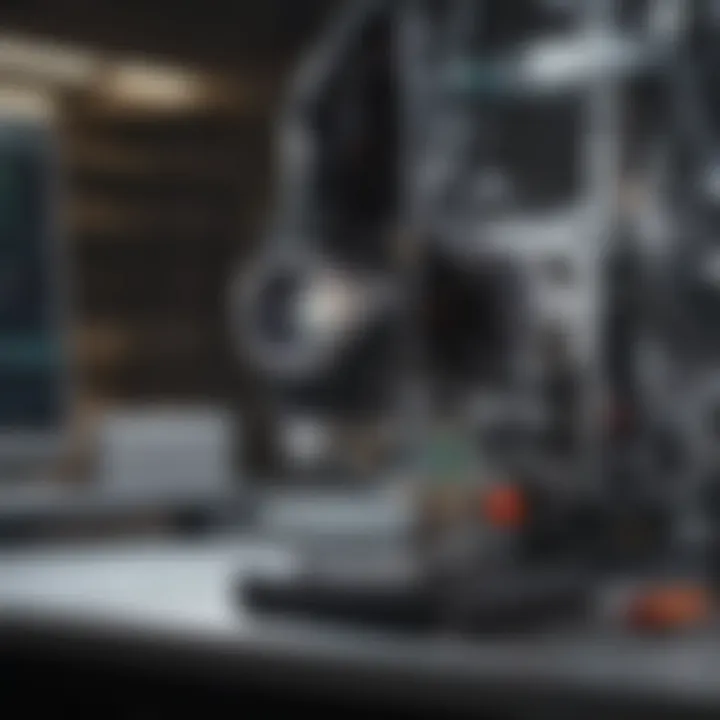
Intro
Fiber photometry and calcium imaging have revolutionized how researchers study neuronal activity in real time. These techniques enable scientists to observe the dynamics of calcium ions within neuronal cells, providing insight into how these cells communicate and function under various conditions. By employing optical methods, researchers can track changes in intracellular calcium concentration, which is integral to understanding the signaling processes in the brain.
The implications of these tools extend far beyond basic neuroscience. They play a critical role in diverse fields such as pharmacology, psychiatry, and cognitive neuroscience, offering advanced techniques to probe the workings of the nervous system.
Article Overview
Purpose of the Article
The purpose of this article is to provide an in-depth exploration of fiber photometry and calcium imaging, addressing both methodologies and their applications. By examining these techniques, we aim to illuminate how they contribute to a richer understanding of neuronal mechanisms. We will highlight recent advancements in technology that have improved resolution and allowed for more nuanced investigations into neuronal activity.
Relevance to Multiple Disciplines
Understanding neuronal functions is relevant to various disciplines, including:
- Neuroscience: Fundamental principles of brain activity are explored in-depth.
- Psychology: Insights into brain processes aid in understanding behavior and cognition.
- Pharmacology: Calcium dynamics inform drug action and efficacy in treating disorders.
Researchers across these fields utilize techniques such as fiber photometry and calcium imaging to contribute to a more comprehensive understanding of neuronal mechanisms.
Research Background
Historical Context
The development of fiber photometry and calcium imaging traces back to earlier optical techniques. Initial methodologies focused on luminescence and fluorescence. Over the years, advancements in materials and engineering have led to more sophisticated tools, enabling real-time measurements of neuronal activity in freely moving animals.
Key Concepts and Definitions
- Fiber Photometry: A technique that measures fluorescence from neuronal populations via an optical fiber. It allows researchers to gauge calcium levels in real time.
- Calcium Imaging: This involves using fluorescent indicators to visualize calcium dynamics. It plays a crucial role in understanding various neuronal processes, such as synaptic transmission.
Through this article, we will explore both techniques in detail, clarifying their methodologies and elucidating a range of applications in contemporary research.
Prolusion to Fiber Photometry and Calcium Imaging
Fiber photometry and calcium imaging are pivotal techniques in the field of neuroscience, with their importance growing in tandem with advancements in technology. Understanding these methods is essential for researchers aiming to investigate neuronal behaviors in real-time. This article seeks to elucidate the nuances of fiber photometry and calcium imaging, covering their principles, applications, and implications for neuroscience research.
The advancements in these imaging techniques have not only made it easier to observe neuronal activity but have also opened new avenues for research. They enable researchers to track physiological processes as they occur, providing insights into how neurons communicate and respond to various stimuli. The relevance of fiber photometry and calcium imaging extends beyond mere observation; they allow scientists to explore the complexities of neural circuits and their underlying mechanisms.
Benefits of Fiber Photometry and Calcium Imaging
- Real-time Monitoring: These techniques allow for continuous observation of neuronal activity in living organisms.
- In Vivo Application: Researchers can assess neuronal functions in a natural environment, helping to bridge the gap between lab findings and real-world applications.
- Diverse Applications: From functional connectivity studies to behavioral assessments, these methods are versatile tools in neuroscience research.
As the field progresses, it is important to consider both the potential and the limitations of these methodologies. While they offer a wealth of information, challenges related to data interpretation, specificity, and sensitivity must be addressed. Nevertheless, the ongoing evolution of these neuroimaging techniques holds promise for the future of brain research.
The Evolution of Neuroimaging Techniques
The history of neuroimaging is marked by continuous innovation. Early techniques, such as traditional histology, allowed scientists to view brain structures, but did not provide dynamic insights into neuronal activity. The development of electrophysiological methods, although groundbreaking, often fell short in spatial resolution.
The introduction of techniques like functional Magnetic Resonance Imaging (fMRI) and Positron Emission Tomography (PET) made it possible to visualize brain function. However, these methods have limitations regarding temporal resolution and the type of data they provide.
Fiber photometry and calcium imaging represent a significant leap forward.
Key Milestones in Neuroimaging Evolution:
- Early Techniques: Histology and basic electrophysiology provided static images of brain anatomy.
- Introduction of fMRI and PET: Created opportunities for tracking brain function over time but with limitations in resolution.
- Development of Fiber Photometry and Calcium Imaging: Combined advantages of spatial and temporal resolution, allowing for real-time monitoring of neuronal activity.
As neuroimaging evolves, the adoption of advanced technologies such as machine learning and novel imaging sensors continues to enhance these techniques, driving the field toward new heights of understanding.
Significance of Calcium Imaging in Neuroscience
Calcium imaging has emerged as a vital method for understanding neuronal function due to its ability to quantify changes in calcium ion concentrations. Calcium ions are crucial for synaptic transmission and signal propagation, making their monitoring essential for grasping neuronal dynamics.
The significance of calcium imaging lies in its capability to provide insights into the mechanisms underpinning neuronal activity. This technique enables researchers to visualize and measure calcium fluctuations in real-time, thus linking activity to behavior.
Importance of Calcium Dynamics:
- Cellular Communication: Changes in intracellular calcium levels signal events such as neurotransmitter release, impacting neural communication.
- Pathological Insights: Understanding calcium signaling pathways may reveal dysfunctions in disease states such as Alzheimer’s or schizophrenia.
- Integration of Complex Signals: Calcium imaging helps explore how neurons integrate information over time and in various contexts, shedding light on learning and memory processes.
Calcium imaging, thus, is not only a tool for observing neuronal behavior, but a window into the very processes that make cognition and memory possible. It is foundational in linking physiological data to behavioral outcomes, further emphasizing its essential role in contemporary neuroscience.
Principles of Fiber Photometry
Fiber photometry represents a critical advancement in the field of neuroscience, providing insights into the dynamic processes of neuronal activity. This technique enables researchers to monitor and measure fluctuations in calcium levels within specific neuronal circuits in real time. The significance of fiber photometry lies in its ability to bridge the gap between observational and experimental neuroscience, allowing for detailed analysis of neuronal behavior in diverse settings.
Optical Components and Setup
The optical setup in fiber photometry is essential for obtaining accurate and reliable measurements. At the core of this setup are optical fibers that deliver light from a laser source to the target neurons. The light used in these systems typically interacts with calcium indicators, such as GCaMP, which fluoresce when bound to calcium ions. The components involved include:
- Light Source: High-intensity lasers are preferred for effective excitation of fluorophores.
- Optical Fibers: These fibers not only deliver light but also collect emitted fluorescence, thus limiting light scattering and improving signal quality.
- Photodetectors: The collected emitted light is then captured by photodetectors, converting it into quantifiable electrical signals.
Setting up these components requires meticulous alignment and calibration to ensure optimal performance. Any misalignment can lead to suboptimal data collection, resulting in challenges in interpreting calcium dynamics accurately.
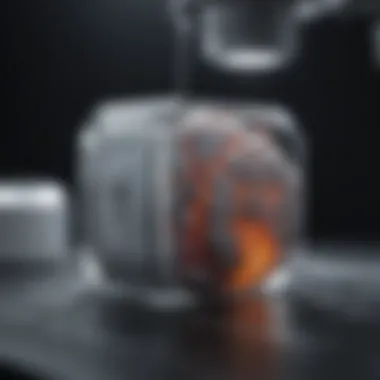
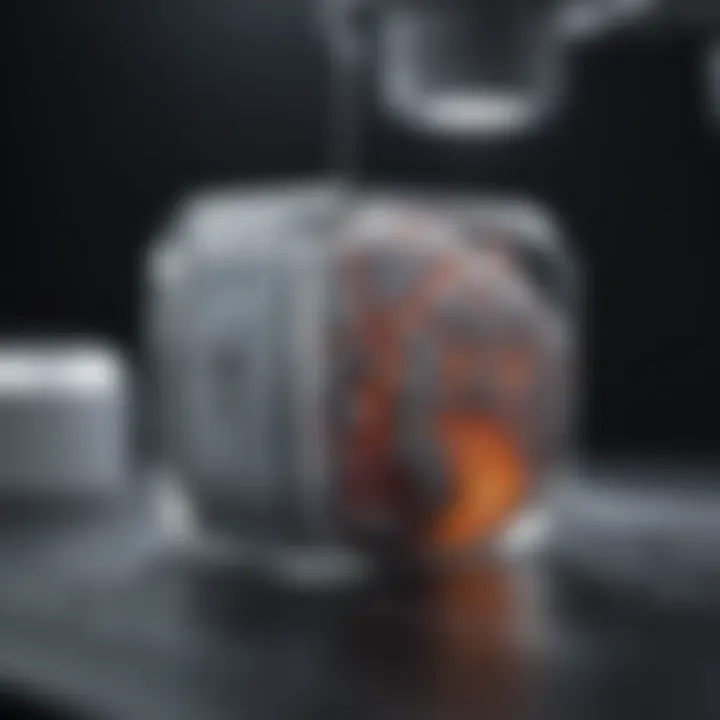
Signal Processing and Interpretation
Once the signal is obtained, the next step involves signal processing, which is crucial for extracting meaningful information from raw data. Fiber photometry data can be inherently noisy, thus necessitating robust processing techniques. Key steps in this process include:
- Baseline Correction: This step removes any offset in the signal, enabling a more accurate assessment of calcium transient peaks.
- Filtering: Digital filters are applied to enhance the signal-to-noise ratio, facilitating clearer detection of calcium fluctuations.
- Normalization: The processed signals are often normalized to account for variations in expression levels of calcium indicators among different samples.
Interpreting the normalized signal allows researchers to draw conclusions on neuronal activity related to behavioral states or external stimuli. By correlating these signals with behavioral outputs or physiological measurements, fiber photometry thus lays the groundwork for understanding complex neuronal networks and their functions in living organisms.
Important Note: The reliability of fiber photometry as a tool for monitoring calcium dynamics in vivo significantly hinges on the quality of the optical setup and the rigor of the signal processing pipeline.
Important Note: The reliability of fiber photometry as a tool for monitoring calcium dynamics in vivo significantly hinges on the quality of the optical setup and the rigor of the signal processing pipeline.
The Mechanics of Calcium Imaging
The mechanics of calcium imaging play a pivotal role in unlocking the complexities of neuronal activity. Understanding these mechanics helps researchers explore how calcium ions function as vital second messengers within neurons. Calcium ions are integral to numerous cellular processes, impacting neurotransmitter release, signal transduction, and gene expression. Therefore, effective visualization of calcium dynamics offers deep insights into both normal and pathological brain functions.
Calcium imaging techniques allow researchers to observe calcium influx in real-time. This is significant because neuronal firing and synaptic activity are closely linked to alterations in calcium levels. With enhanced knowledge of calcium signaling, researchers can investigate various physiological behaviors and changes in response to stimuli or drugs. The effectiveness of calcium imaging is significantly influenced by the choice of calcium indicators and imaging methodologies.
However, there are specific considerations to keep in mind. While calcium imaging offers excellent temporal resolution, it also comes with challenges. It is crucial to select proper indicators that can provide accurate readings without being overly disruptive to the cell. Additionally, imaging conditions should minimize noise and artifacts that can obscure the biological signals.
Using calcium imaging to monitor neuronal activity opens new avenues for understanding how brain circuitry operates, facilitating advancements in neurophysiological research.
Using calcium imaging to monitor neuronal activity opens new avenues for understanding how brain circuitry operates, facilitating advancements in neurophysiological research.
Calcium Indicators: Types and Functionality
Calcium indicators are essential components of calcium imaging. They enable the detection and quantification of calcium levels within cells. Different types of calcium indicators are used, each with specific characteristics suited for various applications.
- Fluorescent Indicators: These indicators fluoresce in response to calcium binding. Common examples include Fura-2, Fluo-4, and GCaMP. Fura-2 operates through a ratiometric technique, offering superiority in measuring calcium levels across different settings.
- Genetically Encoded Indicators: These are proteins that can be expressed within neurons. GCaMP is a prime example. They provide the advantage of being less toxic than chemical indicators and allow for prolonged monitoring of neuronal activity.
- Chemically Sensitive Dyes: These dyes respond to changes in local ion concentration, helping pinpoint calcium levels in specific cellular compartments.
Each type of indicator has unique advantages and limitations, which can impact data fidelity during cerebral studies. Choosing the right calcium indicator enhances measurement accuracy, enabling better interpretation of physiological states.
Imaging Methods and Techniques
In the realm of calcium imaging, various methods and techniques are employed, each with distinct operational mechanics.
- Two-Photon Microscopy: This technique enables deep tissue imaging with high spatial resolution. It is particularly useful in living tissues, providing real-time data about calcium dynamics in neuronal networks.
- Widefield Fluorescence Microscopy: This approach allows for rapid imaging of large areas of tissue. While it offers less spatial resolution than two-photon microscopy, it can efficiently capture the activity of multiple neurons simultaneously.
- Light Sheet Microscopy: This novel technique minimizes photo-bleaching and photo-damage while providing excellent imaging speeds. It offers a unique perspective for capturing calcium dynamics in three-dimensional structures.
When selecting an imaging method, researchers must consider factors like spatial and temporal resolution as well as the specific biological questions they aim to address. The appropriate methodology ultimately enhances the quality of insights gained from calcium imaging studies.
Comparative Analysis of Imaging Techniques
The comparative analysis of imaging techniques stands as a pivotal component of this article. Through this scrutiny, we assess the distinct merits and drawbacks of fiber photometry and traditional calcium imaging methods. Understanding these differences can illuminate how researchers select the appropriate technique for their specific experimental needs. The analysis also underscores the role of these techniques within the broader context of neuroscience research. With an increasing demand for precision and depth in brain studies, it becomes essential to evaluate how these methodologies contribute uniquely to our understanding of neuronal activity.
Fiber Photometry vs. Traditional Calcium Imaging
When comparing fiber photometry and traditional calcium imaging, one must consider various factors such as sensitivity, spatial resolution, and application contexts. Fiber photometry boasts the capability to monitor calcium sensing protein dynamics in a more holistic manner, facilitating real-time activity recording across larger populations of neurons. It operates on a simpler setup and can be seamlessly integrated into live subjects, yielding dynamic insights over time.
In contrast, traditional calcium imaging excels in spatial resolution. It allows for the observation of calcium dynamics within a confined area or individual neurons. By employing fluorescent calcium indicators, researchers can capture fine-grained data about cellular events. However, the limitation here lies in the often cumbersome process that requires elaborate equipment and processing, potentially prohibiting its use in freely moving subjects.
The choice between these methods ultimately pivots on the research objectives. For instance, a study aimed at understanding synaptic connectivity might prefer traditional calcium imaging for its focused detail, while an investigation looking at global brain activity would benefit from fiber photometry. Thus, the analysis reveals that both methods have their rightful place in neuroscientific inquiry, each offering distinct insights that complement each other.
Integration of Multiple Techniques
The integration of multiple imaging techniques emerges as a strategy that brings about synergistic advantages in neuroscience research. By combining fiber photometry with traditional calcium imaging, researchers can obtain a more nuanced understanding of neuronal behavior. This multi-faceted approach can capture both the broader population dynamics and the intricate details of individual neuron functions, creating a comprehensive view of brain activity.
Several studies have begun to exploit this integrative approach, leading to innovative methodologies that increase the robustness of experimental data. For example, one might record global calcium transients via fiber photometry while simultaneously monitoring individual neuron spikes with traditional imaging methods.
Moreover, advancements in data analysis techniques, including machine learning, can enhance the ability to interpret multifaceted datasets generated from these combined methods. As a result, researchers can navigate the complexities of neural circuits with improved accuracy.
Overall, integrating multiple imaging techniques fosters a richer comprehension of neuronal dynamics, which is crucial in advancing neuroscience research._
Overall, integrating multiple imaging techniques fosters a richer comprehension of neuronal dynamics, which is crucial in advancing neuroscience research._
Through this section, it becomes clear that while each method has its strengths and limitations, their collaboration can yield more comprehensive insights into the workings of the brain.
Applications of Fiber Photometry in Research
Fiber photometry has emerged as a pivotal tool in neuroscience, enabling researchers to investigate brain activity with high temporal resolution. This technique has become essential for various research applications, offering unique insights into neural dynamics and functioning. By facilitating real-time monitoring of neuronal activity, fiber photometry helps unravel complex brain mechanisms that former methods struggled to address.
The primary benefits of fiber photometry in research include its ability to monitor large populations of neurons simultaneously, which provides a more comprehensive view of brain activity. Additionally, its minimally invasive nature allows for studies over extended periods. Such qualities are particularly crucial for studying functional connectivity and behavioral assessments, both of which are significant for advancing our understanding of brain processes.
In this section, we will explore two critical applications of fiber photometry: functional connectivity studies and behavioral assessments in animal models.
Functional Connectivity Studies
Functional connectivity studies investigate the relationships and interactions between different brain regions. This research is vital for understanding how various brain areas communicate and cooperate during specific tasks or behaviors. Fiber photometry enables these studies by simultaneously measuring neuronal calcium signals across multiple regions.
One significant advantage of using fiber photometry in functional connectivity studies is the high temporal resolution it offers. Researchers can observe changes in neuronal activity in real-time, linking them to behavioral outputs or stimuli. By employing this method, scientists can trace how activation patterns distribute across different brain areas during complex tasks.
Using fiber photometry, studies have shown how certain brain regions, such as the prefrontal cortex and the amygdala, are involved in processes like decision-making and emotional responses. By understanding these interactions, researchers gain insights into normal brain functioning as well as potential disruptions caused by diseases.
Behavioral Assessments in Animal Models
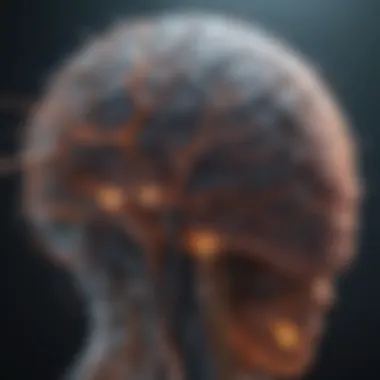
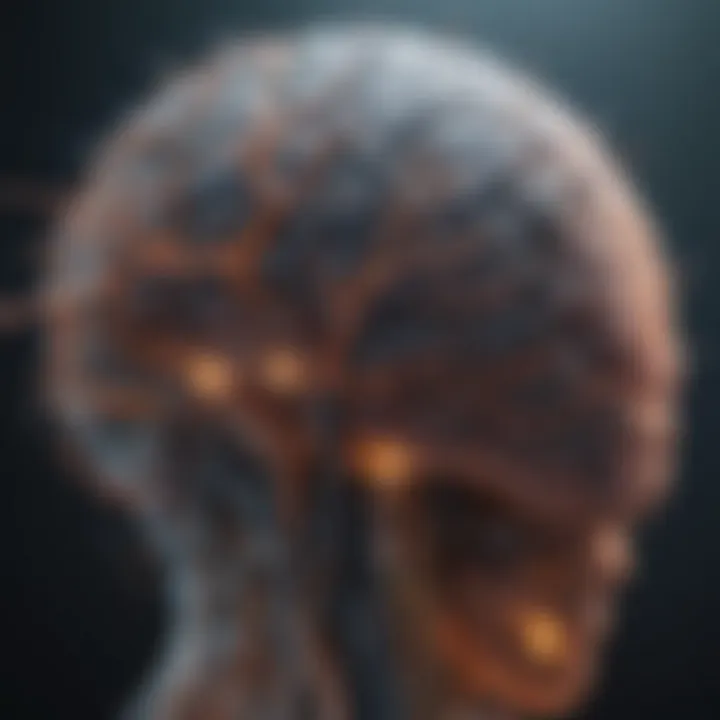
Behavioral assessments in animal models play a crucial role in preclinical research. These studies aim to understand the neural basis of behavior and how it relates to different physiological and pathological states. Fiber photometry allows researchers to correlate behavior with neuronal activity, uncovering the mechanistic underpinnings of various actions.
For instance, fiber photometry can be used to study the neural circuits involved in reward-seeking behaviors or fear responses. By monitoring calcium activity while an animal engages in a specific task, researchers can establish which neuronal populations are crucial for that behavior. Such data are invaluable for developing targeted therapies for disorders characterized by dysfunctional behaviors, such as addiction or anxiety disorders.
In these assessments, the ability to conduct longitudinal studies is a notable advantage of fiber photometry. Researchers can track the same subjects over time, allowing for a detailed analysis of how behavior evolves in response to both natural and experimental perturbations.
"Fiber photometry represents a significant advance in our ability to study the dynamic interplay of neural activity and behavior, bridging the gap between physiology and behavioral outcomes."
"Fiber photometry represents a significant advance in our ability to study the dynamic interplay of neural activity and behavior, bridging the gap between physiology and behavioral outcomes."
The Role of Calcium Imaging in Disease Research
Calcium imaging has emerged as a powerful technique in neuroscience, particularly in the context of disease research. This section will explore the significance of calcium imaging, especially concerning its applications in studying various disorders. Understanding how calcium dynamics relate to neuronal activity can provide insights into the underlying mechanisms of many neurological and psychiatric conditions.
The benefits of calcium imaging in disease research are manifold. Firstly, it offers real-time analysis of calcium signals, which are crucial for neuronal communication. This ability to monitor changes in neuronal activity allows researchers to draw correlations between abnormal calcium signaling and the onset of disease symptoms. Additionally, calcium imaging can be employed to assess the efficacy of potential therapeutic interventions.
Overall, calcium imaging serves as a vital tool. It helps not only in understanding disease etiology but also in developing strategies for treatment. This section will delve deeper into specific applications concerning neurodegenerative and mental health disorders.
Neurodegenerative Disorders
Neurodegenerative disorders such as Alzheimer’s disease and Parkinson’s disease are key areas where calcium imaging plays an important role. The dysregulation of calcium homeostasis has been implicated in the pathophysiology of these diseases. In conditions like Alzheimer’s, abnormal calcium signaling can lead to synaptic dysfunction, contributing to cognitive decline.
Calcium imaging allows researchers to observe the dynamics of calcium in living neurons over time. This helps in identifying the changes in calcium flux that may precede or accompany neuronal death. For instance, studies using calcium indicators have shown increased neuronal calcium signaling in animal models of Alzheimer’s, suggesting a link between heightened calcium levels and the progression of neurodegeneration.
Furthermore, calcium imaging facilitates the exploration of potential therapeutic measures. By examining how certain drugs affect calcium signaling pathways, researchers can identify candidates for preventing or treating these disorders. Overall, the application of calcium imaging in studying neurodegenerative diseases offers valuable insights into both disease mechanisms and intervention strategies.
Mental Health Disorders
Mental health disorders are increasingly recognized as conditions that may stem from neuronal activity anomalies. Calcium imaging holds significant promise in this realm. Disorders such as depression, anxiety, and schizophrenia can often be associated with dysfunctional neuronal circuits, where calcium signaling plays a critical role.
For example, research has indicated that altered calcium signaling may lead to synaptic changes in mice models of depression. These changes are linked to behavioral outcomes that mimic human depression. By utilizing calcium imaging, researchers can identify specific brain regions where these dysregulations occur and monitor the effects of various treatments.
Additionally, calcium imaging can also aid in the understanding of stress responses that are dysfunctional in mental health disorders. It allows the investigation of neuronal responses to stressors in real time. Such insights can inform therapeutic approaches and guide treatments tailored to individual patient needs.
Technological Advancements Enhancing Imaging Techniques
Recent advances in technology significantly enhance fiber photometry and calcium imaging techniques. These advancements not only improve the resolution and accuracy of data obtained but also expand the scope and applicability of these methods in research. Increased precision in imaging represents a transformative change, allowing researchers to gather more detailed insights into neuronal activity and related processes. As such, understanding these advancements is crucial for appreciating the full potential of these imaging modalities.
Development of Novel Calcium Sensors
The progress in calcium sensor technology has been pivotal in the evolution of calcium imaging techniques. Novel sensors, such as genetically encoded calcium indicators like GCaMP, enable researchers to visualize and quantify calcium fluctuations in neurons with high spatial and temporal resolutions. These innovations have several key benefits:
- Improved Sensitivity: Novel sensors often exhibit increased sensitivity to calcium ions, allowing for more accurate recordings of neuronal activity.
- Genetic Targeting: The ability to target specific cell types genetically makes it possible to delineate neuronal networks more effectively.
- Extended Applicability: New calcium indicators can be engineered to function within differing physiological conditions, thus broadening their application range.
However, it is crucial to consider not just the advancements but also the limitations. Factors such as phototoxicity and cellular dynamics must be carefully evaluated in experimental designs to ensure that data truly reflect physiological conditions.
High-Throughput Imaging Systems
The rise of high-throughput imaging systems represents another significant advancement in the field. These systems enable rapid acquisition of large datasets, accommodating the increasing demand for detailed, high-resolution images of neuronal activity across broad populations of cells.
The benefits of high-throughput imaging systems include:
- Increased Data Volume: By capturing images at unprecedented speeds, researchers can analyze more extensive datasets without sacrificing quality.
- Real-Time Analysis: Many high-throughput systems support real-time data analysis, which allows for immediate feedback during experiments.
- Automation and Efficiency: Automation in data acquisition reduces manual errors and enhances reproducibility across experiments.
Integrating these systems into routine research workflows can lead to new discoveries by revealing patterns and connections that may not be obvious with traditional imaging techniques.
High-throughput imaging systems and novel calcium sensors together create a robust framework for advancing our understanding of neuronal functions.
High-throughput imaging systems and novel calcium sensors together create a robust framework for advancing our understanding of neuronal functions.
In summary, technological advancements in sensor development and imaging systems are vital to enhancing our research capabilities. They deepen our insights into the complexity of brain functions and neurophysiological processes, allowing scientists to approach questions previously thought elusive. As such, embracing these innovations ensures that the field of neuroscience continues to thrive and evolve.
Data Analysis and Modeling Techniques
Data analysis and modeling play a crucial role in interpreting the vast amounts of data generated by fiber photometry and calcium imaging experiments. These techniques provide researchers with a way to assess neuronal activity and understand complex patterns that emerge from biological systems. As technology advances, the amount of data collected grows exponentially, making effective analysis methods essential for deriving meaningful conclusions from experiments.
Statistical approaches in data interpretation offer a systematic way to uncover insights from the data. By applying rigorous statistical models, scientists can distinguish between genuine neuronal signals and background noise. This process is essential for validating the accuracy and reliability of experimental findings. Additionally, statistical methods can also quantify variations in neuronal activities across different conditions or populations.
"Data analysis is the backbone of successful experimental neuroscience; without it, results remain ambiguous and unvalidated."
"Data analysis is the backbone of successful experimental neuroscience; without it, results remain ambiguous and unvalidated."
Statistical Approaches in Data Interpretation
Statistical methods encompass a variety of approaches that can be tailored to the specific needs of different studies. Common techniques include:
- Descriptive statistics: Summarize data features, providing a useful overview of trends and distributions.
- Inferential statistics: Help in making predictions and generalizations about larger populations based on sample data.
- Multivariate analysis: Allows researchers to explore relationships between multiple variables simultaneously, revealing complex patterns in neuronal signaling.
Each of these methods not only helps in data interpretation, but also enhances reproducibility and transparency in research, two elements now critical in scientific investigation.
Machine Learning in Imaging Data Processing
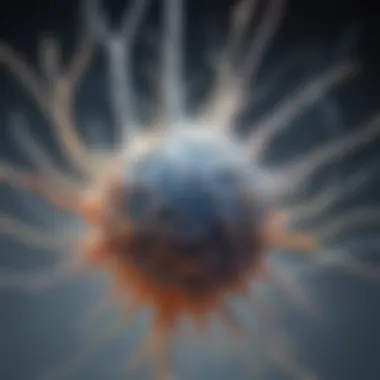
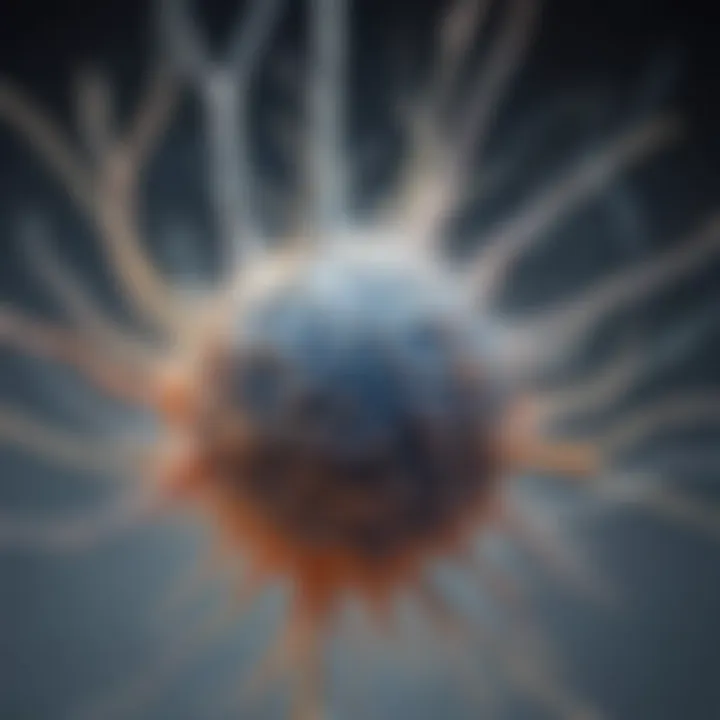
Machine learning (ML) offers innovative solutions to tackle the challenges posed by imaging data. As imaging techniques evolve, the volume and complexity of data can become overwhelming. Machine learning algorithms can improve both speed and precision in data analysis.
Some key applications of machine learning in this context include:
- Automated image segmentation: Machine learning helps identify and delineate cellular structures from raw imaging data efficiently, reducing manual intervention.
- Pattern recognition: Algorithms can learn to recognize specific neuronal activity patterns, offering insights that might be missed by traditional statistical methods.
- Predictive modeling: Researchers can develop models that anticipate neuronal behavior under various conditions, contributing to personalized and targeted research strategies.
In summary, effective data analysis and modeling techniques are pivotal for advancing the field of neuroscience. By combining traditional statistical methods with cutting-edge machine learning approaches, researchers can extract meaningful insights from the complexities of fiber photometry and calcium imaging.
Challenges and Limitations of Imaging Techniques
In the realm of neuroscience, both fiber photometry and calcium imaging have become essential tools for monitoring neuronal activity. However, despite their innovative design and application, these techniques are not without their challenges and limitations. Understanding these challenges is crucial for researchers, as it informs better experimental design and interpretation of results. The complexities intrinsic to these imaging modalities can impact the accuracy, effectiveness, and overall utility of the gathered data.
Techniques Sensitivity and Specificity
Sensitivity and specificity are two paramount aspects that can influence the results obtained from fiber photometry and calcium imaging. Sensitivity refers to the ability of a technique to detect true positives, meaning it measures the neuronal activities effectively even at low levels of fluorescence. On the other hand, specificity concerns the method's capability to exclude false positives or signals that do not come from the targeted neurons.
A frequent issue with sensitivity is that although most calcium indicators function adequately under optimal conditions, their performance may vary significantly under physiological conditions. For example, when a calcium indicator, like GCaMP, is used, factors such as temperature, pH levels, and the density of the indicator within the neuron can affect its reliability in producing accurate signals. The result may lead researchers to either overestimate or underestimate the calcium dynamics and, consequently, the neuronal firing activity.
In addition, specificity can be challenged due to the possible cross-reactivity of indicators. For instance, some calcium indicators may respond to other ions or even external light sources not related to neuronal activity. This nonspecificity dilutes the clarity of the signals and complicates the analysis process. Therefore, researchers must carefully choose their indicators and consider these factors during their experiments to minimize these issues.
Addressing Artifacts in Data
Artifacts in data can hinder the precise interpretation of results in both fiber photometry and calcium imaging. Artifacts may arise from diverse sources, including instrumentation issues, environmental factors, and biological variability. Identifying and mitigating these artifacts is crucial to maintain the integrity of the data collected.
Many common artifacts are related to motion. For example, movement of the subject can cause fluctuations in signal due to noise interference. This motion can lead to spurious signals or result in the loss of certain essential data. To address this, researchers often implement motion correction algorithms during data analysis. Techniques such as optical flow or feature tracking can assist in mitigating motion-related inaccuracies, thereby refining the quality of the data.
Additionally, other artifacts may stem from background fluorescence, which can skew readings. It is essential to establish a baseline measurement and apply correction factors to subtract this background signal, ensuring that only relevant neuronal activity is analyzed.
"Accurate data interpretation hinges on recognizing artifacts and employing robust correction techniques."
"Accurate data interpretation hinges on recognizing artifacts and employing robust correction techniques."
Furthermore, the selection of appropriate controls and calibration strategies can help in minimizing the influence of artifacts. Continuous validation of results through replicative studies enhances reliability, which can lead researchers to more conclusive findings in their investigations.
In summary, while fiber photometry and calcium imaging open up new frontiers for understanding brain functions, recognizing their limitations helps refine methodologies, improving the outcome of neuroscience research.
Future Directions in Fiber Photometry and Calcium Imaging
The exploration of future directions in fiber photometry and calcium imaging marks a crucial intersection of technology and neuroscience. As research intensifies, the methods applied in these imaging techniques evolve. This evolution is significant not only for enhancing current capabilities but also for opening avenues of discovery that were previously unattainable. Improved methodologies will further our understanding of neuronal mechanisms and how they govern behavior.
One specific element to consider is the integration of higher spatial and temporal resolution in imaging techniques. Advancements in optical components could lead to unprecedented detail in observing calcium dynamics. This progress benefits researchers who require precise data about synaptic activity and neuronal signaling. Moreover, implementing multiplexed imaging systems will enable the simultaneous observation of multiple brain regions or cell types, providing a more comprehensive picture of neural circuits.
Incorporating machine learning algorithms into imaging data processing stands out as another significant aspect. This development will allow for better analysis and interpretation of complex data sets. Neural networks can identify patterns that are not immediately visible to the human eye, enhancing our ability to relate calcium signaling to behavioral outcomes.
Benefits of Addressing Future Directions:
- Enhancing accuracy in capturing neuronal activity.
- Enabling real-time monitoring of complex behaviors.
- Improving reliability of data interpretation in large datasets.
As researchers embrace these developments, it is essential to remain cognizant of ethical considerations when employing these advanced technologies. Conducting experiments that involve living organisms necessitates thorough ethical oversight, especially as the precision of these methods increases.
"The future of neuroimaging relies not only on technological advancements but also on our commitment to ethical research practices."
"The future of neuroimaging relies not only on technological advancements but also on our commitment to ethical research practices."
Additionally, collaboration across disciplines will prove vital. As neuroscience, computer science, and engineering converge, innovations in imaging techniques will usher in a new era of research possibilities in understanding brain functions.
Emerging Trends and Innovations
Continuing research demonstrates promising trends in both fiber photometry and calcium imaging. These trends include enhanced calcium indicators that offer improved fluorescent properties. Recent developments in genetically encoded calcium indicators (GECIs) have yielded remarkable sensors like GCaMP6, which exhibit faster kinetics and increased brightness. Such advancements enhance the signal-to-noise ratio during imaging sessions.
The rise of miniaturized, portable imaging devices is another significant trend. These devices facilitate in vivo experiments without disturbing the natural behavior of subjects, particularly in freely moving animals. This trend is crucial since it allows for more ecologically valid behavioral assays that accurately reflect neurophysiological processes.
Prospective Impact on Neuroscience Research
The implications of advancing fiber photometry and calcium imaging techniques are vast. By leveraging these innovations, researchers can expect a shift in our understanding of neurological processes. The ability to capture real-time neuronal activity while subjects engage in various tasks will lead to profound insights into how specific circuits underpin behavior, cognition, and emotional states.
Moreover, the application of these techniques in studying diseases can significantly impact therapeutic interventions. Understanding calcium dynamics in the context of disorders such as Alzheimer’s disease or depression will allow for targeted treatment strategies. This understanding may enable researchers to elucidate the molecular pathways involved, creating a pathway for novel therapeutic approaches.
In summary, the future of fiber photometry and calcium imaging holds the potential to revolutionize neuroscience research. By embracing emerging trends and innovations, while also considering ethical frameworks, this field is poised for profound advancements.
Finale
In this article, we have examined the significance and implications of fiber photometry and calcium imaging in the field of neuroscience. These techniques serve as indispensable tools for real-time monitoring of neuronal activity. They provide critical insights into the functioning of the brain and open avenues for novel research directions. As various methods evolve, understanding their strengths and limitations becomes vital for researchers aiming to apply these techniques effectively.
The ability to track calcium dynamics in living neurons not only enhances the comprehension of neural signaling but also plays a pivotal role in uncovering mechanisms underlying various neurological and mental health conditions. The integration of these imaging methodologies with advancements in technology enriches our study of brain functions.
Summary of Key Points
- Fiber Photometry and Calcium Imaging are essential for understanding neuronal behavior in real time.
- These techniques facilitate the study of calcium indicators, which are integral to mapping neuronal activity.
- Evaluating both traditional and modern approaches reveals the continuous evolution of neuroimaging techniques.
- Applications in research extend from behavioral assessments to disease modeling, showcasing the versatility of these techniques.
- Technological advancements enhance resolution and improve the applicability of these imaging modalities in research.
Final Thoughts on the Future of Imaging Technologies
Looking ahead, the future of imaging technologies in neuroscience appears promising. Emerging trends, including novel calcium sensors and high-throughput imaging systems, will potentially reshape the way researchers investigate brain functions. Collaborations that integrate fiber photometry with genetic and computational approaches are likely to provide comprehensive insights into complex neural circuits. As the demand for precise and high-quality imaging increases, ongoing research will aim to refine these techniques further, making them even more valuable to the scientific community.
The continued evolution of imaging technologies will profoundly impact our understanding of the brain and the treatment of neurodegenerative diseases.
The continued evolution of imaging technologies will profoundly impact our understanding of the brain and the treatment of neurodegenerative diseases.
This discourse on fiber photometry and calcium imaging highlights their importance not only in academia but also in advancing therapeutic strategies in clinical settings. We can anticipate a future where these techniques will lead to significant breakthroughs in neurobiology.