Fluorescent Dye: Applications and Future Directions
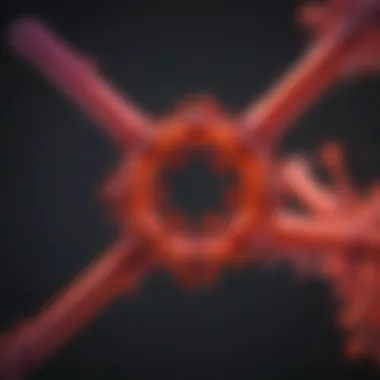
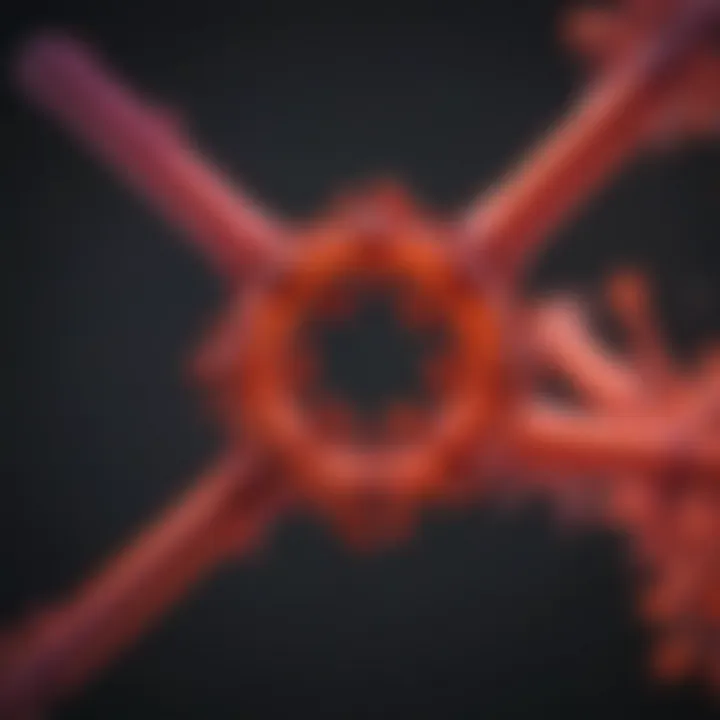
Article Overview
Purpose of the Article
This article aims to provide a comprehensive understanding of fluorescent dyes. It covers their unique chemical properties, the mechanisms behind their fluorescence, and the various applications they serve across multiple scientific disciplines. This analysis reveals not only current uses but also emerging trends and innovations in the field, thereby enhancing the reader's grasp of this multifaceted topic.
Relevance to Multiple Disciplines
Fluorescent dyes play a crucial role in various scientific areas, such as biology, medicine, chemistry, and materials science. Their ability to emit light when excited makes them invaluable for imaging, diagnostics, and developing new materials with specific properties. This article underscores their importance, showcasing how they bridge multiple fields by offering solutions to complex challenges in research and industry.
Research Background
Historical Context
The study of fluorescent dyes began in the late 19th century when scientists started exploring the properties of certain compounds under ultraviolet light. The advancements in organic and polymer chemistry have since paved the way for the development of a variety of synthetic fluorescent dyes. Early applications focused on biological research, but over time, the capabilities of these dyes have expanded significantly, now encompassing a wide array of fields.
Key Concepts and Definitions
Understanding fluorescent dyes requires familiarity with some key concepts:
- Fluorescence: The process by which a substance absorbs light or electromagnetic radiation and then re-emits it. This happens almost instantaneously, making it a very useful tool in various applications.
- Quantum Yield: A measure of the efficiency of fluorescence. It refers to the ratio of photons emitted to photons absorbed. A higher quantum yield indicates more efficient fluorescent dyes.
- Stokes Shift: This is the difference in wavelength between the absorbed light and emitted light. It is crucial in preventing overlap between excitation and emission spectra, which can impact imaging quality.
Fluorescent dyes are typically characterized by their optical properties, which can be manipulated depending on their chemical structure and the environment in which they are used. This versatility has led to innovative applications in medical diagnostics, environmental monitoring, and numerous other areas.
Prolusion to Fluorescent Dyes
Fluorescent dyes have transformed how researchers and scientists analyze biological and chemical processes. Their unique properties make them essential tools in various fields, from basic research to applied sciences. By emitting light upon excitation, these dyes provide vital visualizations that enhance our understanding of complex systems.
The significance of fluorescent dyes lies in their ability to highlight specific cellular components, aiding in real-time imaging and diagnostics. This capability allows for advancements in medical research and therapeutic applications. Moreover, the integration of these dyes into materials science has led to new innovations in product design, coatings, and inks.
In this section, we will define fluorescent dyes and explore their historical context. Understanding the foundational aspects of fluorescent dyes is crucial for grasping their applications and mechanisms, which we will delve into in subsequent sections.
Definition of Fluorescent Dyes
Fluorescent dyes are organic compounds that exhibit fluorescence. This means they can absorb light at one wavelength and then emit light at a longer wavelength. This process occurs due to electronic transitions in the dye molecules. Generally, these dyes are highly sensitive and can be tailored to respond to specific wavelengths of light.
Their versatility allows them to be used in various applications, including staining in microscopy, where they help in visualizing cells and tissues. Fluorescent dyes come in different colors, each suited for particular uses, providing researchers with a robust set of tools for analysis and imaging.
History and Evolution
The history of fluorescent dyes is marked by significant milestones that reflect advancements in chemistry and material science. The first synthetic dyes emerged in the late 19th century. This era was characterized by the search for chemical compounds that would change colors and provide vibrant pigments.
In the early 20th century, researchers began to understand the principles of fluorescence. The development of fluorescent molecules progressed slowly until around the 1950s, when biologists started employing these dyes for electron microscopy. Their use in fluorescence microscopy revolutionized biological imaging, enabling scientists to view cellular processes with unprecedented clarity.
Today, the field continues to evolve with the synthesis of new, more efficient dyes. Advances in nanotechnology and bioengineering have opened new avenues for developing specialized fluorescent probes that cater to specific research needs. Understanding this evolution is vital for appreciating how fluorescent dyes have become a cornerstone in scientific research and applications.
Chemical Properties of Fluorescent Dyes
Fluorescent dyes exhibit distinct chemical properties that significantly influence their application in various scientific fields. Understanding these properties is crucial for researchers and professionals engaged in areas such as biological imaging, diagnostics, and materials science. The chemical properties determine how these dyes interact with light, their stability under environmental conditions, and their compatibility with biological systems. In this section, we explore three fundamental aspects: molecular structure, absorption and emission spectra, and stability and solubility.
Molecular Structure
The molecular structure of fluorescent dyes plays a pivotal role in their functionality. Fluorescent dyes are typically composed of a conjugated system, which consists of alternating single and double bonds. This feature allows for delocalization of electrons, making the molecule more responsive to light. The structural framework often includes various functional groups that can affect solubility and binding affinity to specific targets in biological systems.
For example, xanthene dyes, such as fluorescein, have a basic structure that enhances their ability to fluoresce strongly. Likewise, rhodamine dyes derive their fluorescence from a similar core structure, modified by additional functional groups. These variations in molecular structure allow for a diverse range of fluorescent properties, providing researchers with choices suited to various applications.
Absorption and Emission Spectra
Absorption and emission spectra are essential to understanding how fluorescent dyes function. Each dye has specific wavelengths at which it absorbs light. When excited by a photon of light at the absorption wavelength, the dye transitions to a higher energy state. It subsequently releases energy as it returns to the ground state, emitting light at a different wavelength. The difference between the absorption and emission wavelengths is known as the Stokes shift.
"The Stokes shift is a critical parameter; it helps reduce background noise in imaging applications."
"The Stokes shift is a critical parameter; it helps reduce background noise in imaging applications."
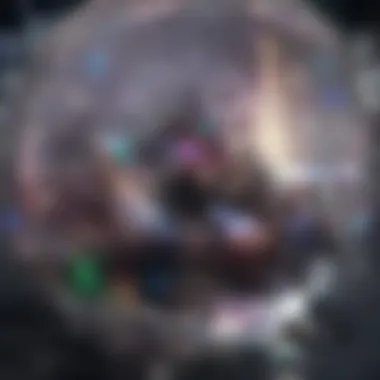
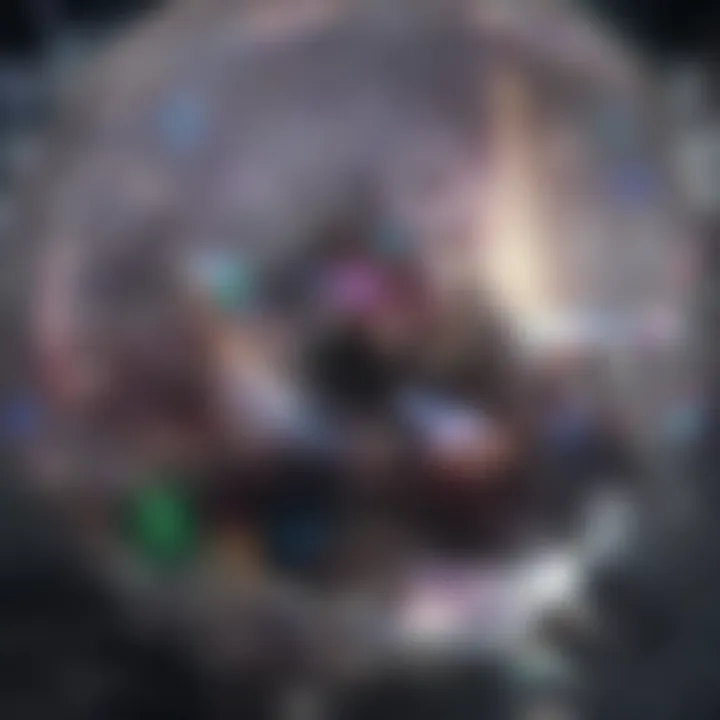
The efficiency of this process can influence the brightness of the dye and its suitability for particular applications, such as fluorescent microscopy or flow cytometry. Dyes with large Stokes shifts are often preferred, as they minimize overlap with the excitation light, improving image clarity.
Stability and Solubility
Stability and solubility are considerable considerations when selecting fluorescent dyes for research and application. Fluorescent dyes must maintain their properties over time, even when exposed to light, heat, and varying pH levels. Photobleaching, the process where dyes lose their ability to fluoresce upon prolonged exposure to light, is a significant challenge. To counteract this, researchers often seek dyes that are chemically stable and have high resistance to photodegradation.
Additionally, solubility in biological fluids is crucial for in vivo applications. Dyes should be biologically compatible, minimizing toxicity while ensuring efficient delivery and reporting. For instance, dyes that can be functionalized to increase their water solubility are often preferred for biological imaging.
In summary, the chemical properties of fluorescent dyes are fundamental to their effective application in scientific research. Understanding their molecular structure, absorption and emission spectra, and stability and solubility ensures that researchers can choose the appropriate dyes for their experimental needs.
Mechanisms of Fluorescence
Understanding the mechanisms of fluorescence is essential for utilizing fluorescent dyes effectively in various applications. These mechanisms underlie the behavior of fluorescent compounds and how they interact with light. Knowledge of these processes can enhance the choice and design of fluorescent dyes for specific applications in research and industry.
Photon Absorption
Photon absorption is the initial step in the fluorescence process. When a fluorescent dye is exposed to light within a specific wavelength range, usually ultraviolet or visible light, it absorbs photons. This leads the dye molecules to transition from their ground state to an excited electronic state. The efficiency of this absorption process depends on various factors including the dye's molecular structure, solvent environment, and the intensity of light applied.
The absorption spectrum of the dye indicates the wavelengths that will excite the molecules effectively. It is crucial to match the light source to the dyeβs absorption spectrum for optimal performance. For instance, if a dye has an absorption peak at 500 nm, using light close to this value will enhance the intensity of fluorescence observed.
Excited State Dynamics
Once the dye molecules are excited, they enter a higher energy state. This intermediate phase is pivotal, as it includes both the excited state lifetime and the internal conversion processes. The energy absorbed from photons can dissipate in several ways, including vibrational relaxation, where energy is redistributed within the molecule before light emission occurs.
The excited state lifetime varies between different fluorescent dyes. Longer lifetimes often increase the likelihood of emitting a photon rather than losing energy non-radiatively. Understanding these dynamics helps in designing better fluorescent probes that can be used in high-resolution imaging procedures, where precise timing and signal clarity matter.
Radiative vs Non-Radiative Transitions
The distinction between radiative and non-radiative transitions is fundamental to the understanding of fluorescent efficiency. Radiative transitions occur when the excited molecule returns to its ground state and emits a photon, which is the observable fluorescence. Non-radiative transitions, however, dissipate energy as heat without emitting light.
Minimizing non-radiative losses is critical to maximize the brightness of a fluorescent dye. Factors such as molecular rigidity and the presence of substituents can affect these transitions. For instance, fluorescent dyes with rigid structures tend to promote radiative processes by limiting the vibrational modes available for non-radiative decay.
In summary, the mechanisms of fluorescence are crucial for the effective application of fluorescent dyes. Photon absorption initiates the process, excited state dynamics determine the efficiency of fluorescence, and the balance between radiative and non-radiative transitions influences the overall brightness and utility of the dye.
In summary, the mechanisms of fluorescence are crucial for the effective application of fluorescent dyes. Photon absorption initiates the process, excited state dynamics determine the efficiency of fluorescence, and the balance between radiative and non-radiative transitions influences the overall brightness and utility of the dye.
Applications in Biological Imaging
Fluorescent dyes have transformed the landscape of biological imaging. These dyes serve as invaluable tools in various fields, including cell biology, molecular biology, and medical diagnostics. The primary benefit of using fluorescent dyes is their ability to emit light of specific wavelengths when excited by light of a shorter wavelength. This property enhances the contrast of biological samples, allowing researchers to visualize structures and processes that are otherwise difficult to discern.
The significance of this section lies in exploring key applications of fluorescent dyes in biological imaging and understanding the underlying techniques. Their relevance is underscored by advances in microscopy and other imaging modalities that rely on these dyes to reveal detailed insights into cellular functions. Moreover, the growing interest in personalized medicine and targeted therapies further highlights the critical role of these dyes in clinical research.
Fluorescent Microscopy Techniques
Fluorescent microscopy is a cornerstone technique in the field of biological imaging. Different types of microscopy techniques utilize fluorescent dyes in unique ways. For instance, wide-field fluorescence microscopy allows for the imaging of large areas of a sample, making it ideal for assessing cell populations. This technique is particularly useful in observing cellular morphology and interactions within tissues.
On the other hand, confocal microscopy provides improved optical resolution and contrast by using point illumination and spatial filtering. This enables detailed three-dimensional reconstructions of biological specimens. By employing fluorescent dyes with different spectra, researchers can perform multi-color imaging, allowing the concurrent tracking of multiple cellular components.
Another noteworthy technique is super-resolution microscopy. This approach disrupts the diffraction limit of traditional microscopy, achieving resolutions that exceed 200 nanometers. It allows for the visualization of sub-cellular structures and dynamics at unprecedented levels of detail. Evaluating the effectiveness of these techniques can enhance the precision of biological studies and foster new discoveries in the field.
Flow Cytometry
Flow cytometry is a powerful technique that leverages fluorescent dyes to analyze the physical and chemical characteristics of cells. By labeling cells with specific fluorescent dyes, researchers can examine multiple parameters simultaneously. This application is essential in immunology, where it aids in the identification and characterization of various immune cell types.
One of the key advantages of flow cytometry is its ability to analyze thousands of cells per second, allowing for high-throughput screening of cell populations. Moreover, it provides quantitative data regarding the expression levels of targeted proteins. This application is significant in the study of diseases, such as cancer, where understanding the heterogeneity of cell populations can assist in diagnosis and treatment strategies. Flow cytometry continues to be a mainstay in clinical labs and research facilities, underscoring its importance in modern biological imaging.
In Vivo Imaging
In vivo imaging using fluorescent dyes has opened up new dimensions in the way researchers study biological processes within living organisms. This technique allows for the visualization of dynamic processes such as protein interactions, cellular trafficking, and metabolic pathways in real-time. By utilizing fluorescent probes that can target specific cells or tissues, scientists can gather insights about disease progression and treatment responses.
For example, the advent of near-infrared fluorescent dyes has enhanced in vivo imaging capabilities. These dyes penetrate deeper into tissues and reduce background signal interference, making them suitable for imaging in live animals. Techniques such as fluorescence molecular tomography and bioluminescence imaging are increasingly being employed to study tumor behavior and therapeutic efficacy.
The integration of fluorescent dyes in in vivo imaging underscores their pivotal role in advancing our understanding of biological systems, offering a pathway to revolutionize diagnostics and therapeutic interventions.
Diagnostic Applications
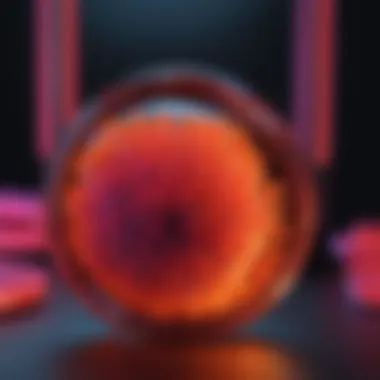
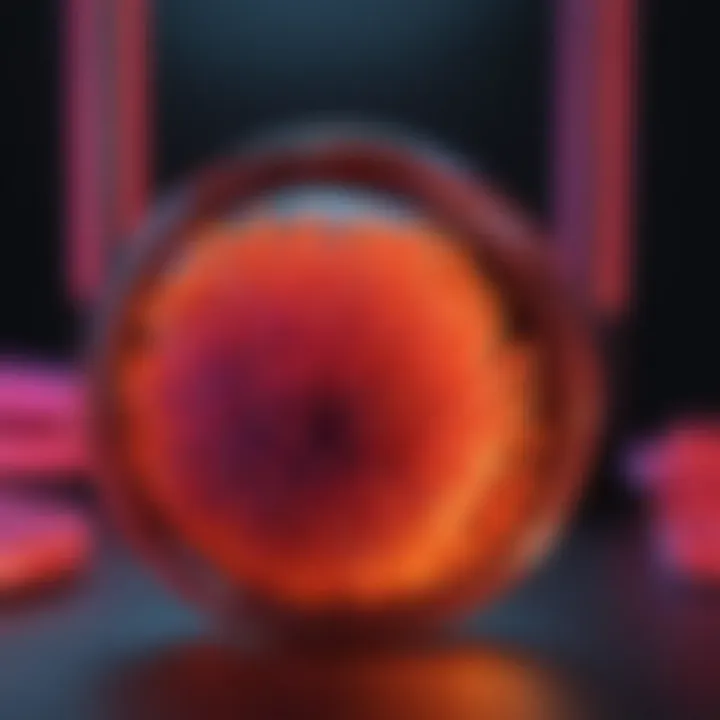
Diagnostic applications of fluorescent dyes play a crucial role in modern medicine and research. These applications allow for precise identification and quantification of various biological substances. The use of these dyes enhances the sensitivity and specificity of diagnostic tests. Consequently, they become invaluable for detecting diseases, monitoring health, and guiding treatment decisions.
Clinical Diagnostics
Fluorescent dyes are widely used in clinical diagnostics due to their ability to highlight specific biomolecules in complex samples. Techniques such as fluorescent microscopy allow clinicians to visualize and assess cellular structures in real-time. For example, fluorescent dyes can tag antibodies, which then bind to target antigens. This process enables detection of pathogens or abnormal cells in a specimen.
The advantages of using fluorescent dyes include increased detection sensitivity and precision. They can be employed in various assays such as enzyme-linked immunosorbent assays (ELISA). Additionally, tools like the.bioplex assay system rely on fluorescent signaling for multiplex analysis, permitting simultaneous detection of multiple targets in one sample.
Pathogen Detection
The rapid and accurate detection of pathogens is essential in controlling infectious diseases. Fluorescent dyes enhance molecular techniques such as polymerase chain reaction (PCR). For instance, dyes like SYBR Green or TaqMan probes bind to the DNA during amplification providing fluorescent signals that indicate the presence of specific pathogens.
Using fluorescent dyes raises the efficiency of pathogen detection. They can be utilized in food safety, environmental monitoring, and clinical microbiology. This capability is critical in managing outbreaks and ensuring public health safety. Fluorescent techniques also facilitate the identification of antibiotic resistance genes, further elevating their importance in clinical diagnostics.
Cancer Biomarkers
Fluorescent dyes have become instrumental in identifying cancer biomarkers. These markers are indicators of cancer presence and can be measured using fluorescent-based assays. For example, dyes can label specific RNA or protein biomarkers associated with different cancer types. Techniques such as fluorescent in situ hybridization (FISH) allow pathologists to visualize genetic abnormalities.
The utilization of fluorescent dyes in cancer diagnostics provides several benefits. They enable early detection of tumors, which is crucial for improving patient outcomes. Moreover, fluorescence-based techniques are adapted for high-throughput screening of candidate drugs in research settings. Hence, fluorescent dyes contribute significantly to advancing personalized medicine by allowing tailored treatment plans based on detected biomarkers.
Fluorescent dyes not only enhance current diagnostic capabilities but also pave the way for innovative approaches in disease management and therapeutic interventions.
Advancements in Material Sciences
The field of material sciences has seen significant progress in the integration of fluorescent dyes. These compounds are not just tools for imaging; they have become pivotal in enhancing material properties. Their applications in polymers and coatings have revolutionized the production processes and the final characteristics of various products.
Fluorescent dyes have the ability to improve visual properties, detect defects, and even provide functionalities like UV protection. In this section, we explore how these dyes enhance polymers and their use in coatings and inks, along with the implications of these advancements.
Fluorescent Dyes in Polymers
Incorporating fluorescent dyes into polymers can yield materials with superior optical properties. The vivid colors and brightness derived from these dyes are essential in various applications, ranging from consumer goods to sophisticated industrial products. These polymers can serve multiple purposes, including those in protective equipment and sensors.
Benefits of Using Fluorescent Dyes in Polymers
- Improved Aesthetics: The vibrant coloring enhances product appeal.
- Functional Insights: They can provide feedback on polymer behavior under various conditions.
- Detection Capabilities: Fluorescent dyes can highlight wear and tear in polymers, simplifying maintenance and repair processes.
Challenges also exist. It is essential to ensure that the dyes are stable under light and heat to prevent fading and degradation of the material. Furthermore, compatibility with the polymer matrix can affect the performance and longevity of the final product.
Use in Coatings and Inks
Fluorescent dyes play a crucial role in coatings and inks, offering opportunities for innovation in both aesthetic and functional applications. They are increasingly used in safety markings, decorative finishes, and even in industrial coatings to enhance visual markers or alerts.
Key Uses in Coatings and Inks
- Safety Applications: Bright hues improve visibility in low-light conditions, crucial for safety signage.
- Quality Control: Inks enriched with fluorescent dyes can signal defects or improper application during manufacturing.
- Dynamic Features: Some coatings change color in response to environmental factors, providing real-time information.
The challenge here often revolves around ensuring the durability of the dyes and preventing photobleaching, which can render the coatings ineffective over time. Innovations in encapsulation techniques have also emerged to address this issue, allowing for longer-lasting applications.
Challenges in the Use of Fluorescent Dyes
Fluorescent dyes have significantly advanced scientific research and applications. However, their use is accompanied by certain challenges that can hinder their effectiveness and reliability. Addressing these challenges is crucial for maximizing the potential of fluorescent dyes in various fields. Understanding these issues allows researchers to improve methodologies and enhance the quality of results.
Photobleaching
Photobleaching is a notable challenge when working with fluorescent dyes. It refers to the irreversible loss of fluorescence intensity over time due to excessive exposure to light. This phenomenon occurs because the excited dye molecules can undergo chemical changes, leading to a decrease in their luminescent properties. The rate of photobleaching can vary depending on the dye, its environment, and the intensity of illumination.
To mitigate photobleaching, researchers can adopt several strategies:
- Use lower light intensities during imaging.
- Implement pulsed laser excitation to reduce continuous exposure.
- Choose photostable dyes that are less prone to degradation.
Despite these strategies, photobleaching remains a concern, especially in long-term experiments. Researchers must consider this when designing experiments to ensure accurate interpretation of fluorescence data.
Toxicity Concerns
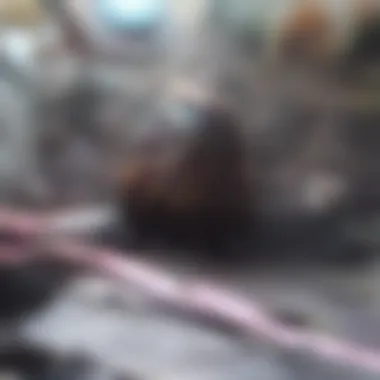
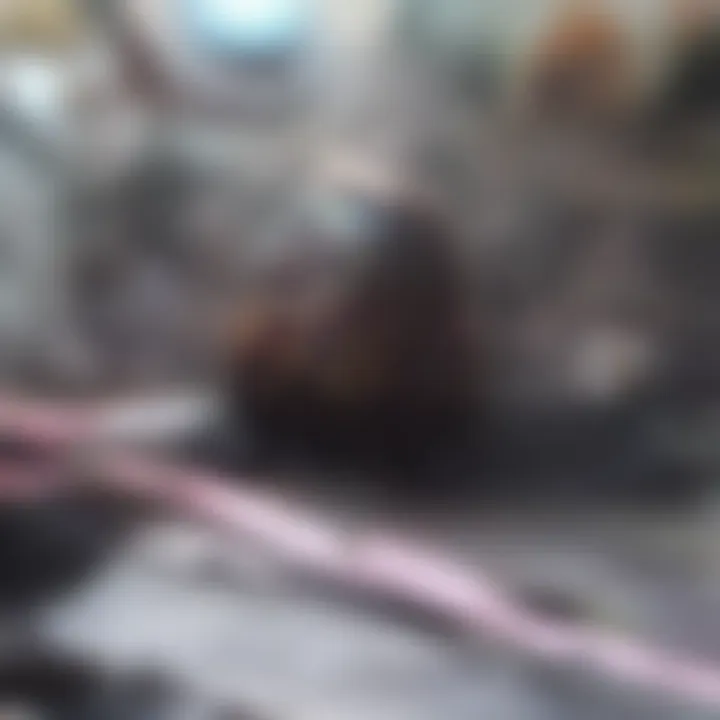
Another significant barrier to using fluorescent dyes is the potential toxicity associated with specific compounds. Many dyes may have harmful effects on biological systems, affecting cell viability and function. For instance, dyes like fluorescein and rhodamine can be cytotoxic at high concentrations. Additionally, the toxic effects can vary across different cell lines and organisms.
To address toxicity concerns:
- Select fluorescent dyes with lower toxicity profiles where possible.
- Use appropriate controls to ascertain the impacts on biological assays.
- Optimize dye concentrations to minimize adverse effects on living tissues.
Researchers must balance the desire for high-contrast imaging with the need to maintain biological integrity during experiments. Understanding the toxicity mechanisms can foster the development of safer alternatives.
Signal Overlap in Complex Samples
Signal overlap poses another challenge in utilizing fluorescent dyes effectively. In complex samples, multiple fluorescent species can emit at similar wavelengths, leading to spectral overlap and difficulties in quantifying signals accurately. This is particularly problematic in multicolor imaging where differentiating between overlapping signals is essential for correct data interpretation.
To combat signal overlap:
- Employ spectral unmixing techniques to isolate signals.
- Utilize dyes with maximized spectral separation.
- Optimize experimental conditions to reduce interference from auto-fluorescence in samples.
The complexity of biological systems often necessitates careful planning and execution to avoid misinterpretation of results. Overcoming these challenges requires ongoing innovation and adaptability in experimental design.
By recognizing and addressing these challenges, researchers can enhance the reliability of fluorescent dyes in scientific applications, paving the way for future advancements in this field.
By recognizing and addressing these challenges, researchers can enhance the reliability of fluorescent dyes in scientific applications, paving the way for future advancements in this field.
Future Directions in Fluorescent Dye Research
The exploration of fluorescent dyes is consistently evolving, leading to new frontiers in research and application. Understanding the future directions in this field is essential for researchers and professionals who wish to stay at the forefront of scientific advancements. Technological innovations are often pivotal in unlocking the full potential of fluorescent dyes, enhancing their utility in various domains. This section will discuss the development of new fluorescent probes, the integration with nanotechnology, and the expansion into new fields. Each of these elements presents unique benefits and challenges that can shape the trajectory of research in this domain.
Development of New Fluorescent Probes
Creating new fluorescent probes is crucial for improving specificity and sensitivity in various applications, particularly in biological imaging and diagnostics. New probes can be designed to target specific biomolecules or cellular processes, enabling more precise measurements in complex environments. The design factors such as brightness, photostability, and compatibility with biological systems are taken into consideration. Innovations in synthetic methodologies can lead to the discovery of novel compounds that exhibit desired properties.
- Benefits:
- Enhanced imaging capabilities.
- Targeted detection of biomarkers.
- Improved resolution in fluorescence microscopy.
While synthesizing new probes, challenges remain. For instance, ensuring minimal toxicity while retaining efficacy is critical in biological contexts. Moreover, it is essential to consider the stability of these probes under various environmental conditions. Researchers are motivated to develop dyes that can maintain their fluorescent properties even in dynamic biological systems.
Integration with Nanotechnology
The convergence of fluorescent dyes and nanotechnology opens remarkable avenues for their application. Nanoparticles can be functionalized with fluorescent dyes, improving their photophysical properties and facilitating targeted delivery to specific cells or tissues. This combination not only enhances imaging techniques but also leads to the development of more effective diagnostic tools.
"Nanotechnology can significantly amplify the benefits of fluorescent probes, creating more robust and versatile tools for scientific investigation."
"Nanotechnology can significantly amplify the benefits of fluorescent probes, creating more robust and versatile tools for scientific investigation."
Additionally, exploring the interactions between fluorescent dyes and nanomaterials can lead to the development of hybrid systems that exhibit unique optical and electronic properties. These systems can find applications in sensors, drug delivery, and therapeutic agents. However, researchers must be cautious of potential challenges regarding biocompatibility and long-term stability in vivo.
Expansion into New Fields
Fluorescent dyes are increasingly being recognized for their potential in unconventional areas beyond traditional biomedical applications. As researchers delve into fields such as environmental monitoring and food safety, fluorescent dyes can serve as powerful tools for detecting pollutants or contaminants.
- Emerging Applications:
- Environmental Science: Using fluorescent dyes to track pollutants in water sources.
- Food Safety: Detecting harmful substances or pathogens in food products.
- Material Science: Investigating properties of new materials through fluorescence.
This expansion necessitates a robust understanding of the environmental impact of these dyes and the consideration of sustainable practices in their synthesis and application.
In summary, these future directions not only promise enhanced capabilities of fluorescent dyes in current applications but also encourage innovation into new territories. The continued research and development in the area of fluorescent dyes will undoubtedly contribute to significant advancements across multiple scientific disciplines.
Epilogue
In this article, we have delved into the multifaceted world of fluorescent dyes, an essential tool in various scientific domains. The conclusion highlights the importance of synthesizing the components discussed, reinforcing the scientific and practical significance of fluorescent dyes. The diverse applications span biological imaging and diagnostic techniques, showcasing the critical role these dyes play in advancing research and improving medical outcomes.
Summary of Key Points
- Definition and Properties: Fluorescent dyes possess unique chemical properties, including their molecular structure and spectral characteristics. This allows for effective absorption and emission of light, key to their applications.
- Mechanisms of Fluorescence: Understanding the fundamentals of photon absorption and subsequent dynamic processes leading to fluorescence is pivotal. Knowledge of radiative and non-radiative transitions sheds light on optimizing dye performance.
- Versatile Applications: As we explored, fluorescent dyes serve integral roles in biological imaging, clinical diagnostics, and material sciences. They enable detailed visualizations and sensitive detections, which are crucial for research and diagnoses.
- Challenges and Future Directions: Awareness of issues like photobleaching and toxicity informs better practices. Ongoing research is vital for developing innovative dyes, expanding their utility through integration with nanotechnology, and pushing the boundaries into new scientific fields.
Closing Thoughts
As research continues, the applications of fluorescent dyes are expected to evolve. There is great potential for breakthroughs in how we utilize these tools for deeper understanding across disciplines. The integration of fluorescent dyes into advanced technologies could open doors for novel discoveries.
Fluorescent dyes will likely remain at the forefront of scientific inquiry due to their utility and versatility. Continued exploration is paramount, as it promises to enhance our capability to address complex biological and chemical questions. Ultimately, as we expand our knowledge and refine techniques, the future of fluorescent dye technology appears bright.