Fuel Cell Carbon Capture: A Comprehensive Analysis
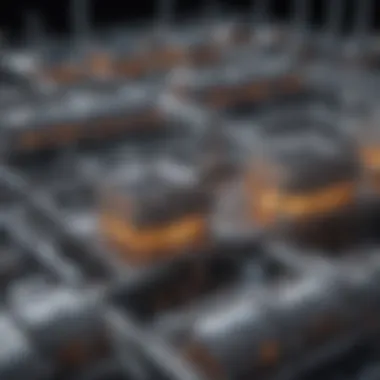
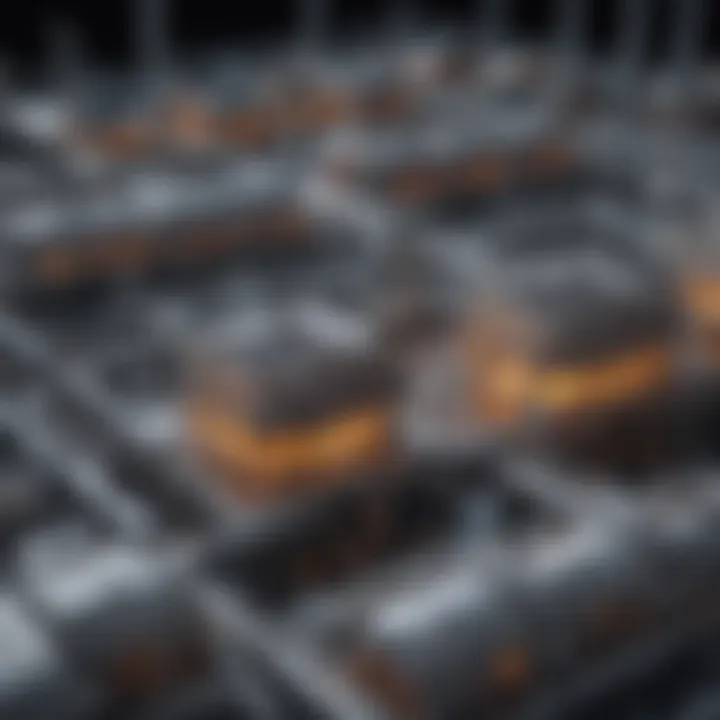
Article Overview
Purpose of the Article
The aim of this article is to provide a comprehensive understanding of the integration of fuel cells with carbon capture technologies. This is crucial in searching for solutions to combat climate change. The article will discuss how these two technologies function separately, and how they complement each other to enhance energy efficiency while significantly reducing carbon emissions. By offering insights into their mechanisms, benefits, challenges, and real-world applications, the article hopes to clarify the role fuel cell carbon capture can play in achieving a sustainable future.
Relevance to Multiple Disciplines
The intersection of fuel cells and carbon capture has implications in various fields like engineering, environmental science, and policy-making. Understanding this integration is crucial for students, researchers, and professionals alike. It touches upon innovative energy solutions, climate policy debates, and advancements in sustainable technology. Thus, a multidisciplinary approach is necessary for assessing its overall impact on our energy landscape.
Research Background
Historical Context
The pursuit of cleaner energy solutions is not recent. Fuel cell technology has its roots in the early 19th century but gained momentum in the mid-20th century due to the growing concern over fossil fuels and greenhouse gas emissions. Carbon capture began to be seriously explored in the late 20th century as a method for mitigating the environmental impact of conventional energy sources. Examining their evolution reveals a clear trend toward the integration of these technologies, shaped by policy incentives and a collective desire for sustainability.
Key Concepts and Definitions
Understanding the fundamental concepts is key to appreciating the synergy between fuel cells and carbon capture. Fuel cells convert chemical energy directly into electrical energy through an electrochemical reaction, primarily involving hydrogen and oxygen. On the other hand, carbon capture refers to a range of technologies aimed at capturing carbon dioxide emissions from sources like power plants. By integrating these concepts, we see a pathway to harness energy while simultaneously mitigating harmful emissions.
"Integrating fuel cells with carbon capture technologies may be one of the most transformative steps toward achieving energy sustainability."
"Integrating fuel cells with carbon capture technologies may be one of the most transformative steps toward achieving energy sustainability."
The combination of these technologies holds promise not only for industrial applications but also for domestic situations. Overall, a critical evaluation of this integration can lead to a more sustainable energy ecosystem.
Prolusion to Fuel Cells and Carbon Capture
The interconnection of fuel cells and carbon capture is gaining significance in today’s energy discourse. As the world grapples with climate change and a transition to sustainable energy sources, understanding this relationship is crucial. Fuel cells provide a clean method of energy production with water as the main byproduct. Integrating carbon capture technology allows the mitigation of greenhouse gases, particularly carbon dioxide, in processes that would otherwise emit these pollutants into the atmosphere.
The benefits of combining fuel cells with carbon capture are multifaceted. This integration could provide a pathway to carbon-negative energy systems. It represents an informed response to the urgent need for alternatives to fossil fuel dependency. Additionally, these technologies are aligned with global objectives of lowering carbon emissions and enhancing energy efficiency. However, the implementation of these systems presents various considerations including cost, technology maturity, and regulatory frameworks.
The Role of Fuel Cells in Energy Production
Fuel cells are pivotal in modern energy production. They operate by converting chemical energy from fuels directly into electricity. This process utilizes an electrochemical reaction between hydrogen and oxygen. The most prevalent types of fuel cells include Proton Exchange Membrane Cells, Solid Oxide Fuel Cells, and Alkaline Fuel Cells. Each varies in their operating temperatures, efficiency levels, and applications.
For instance, Proton Exchange Membrane Cells are favored for transportation applications due to their operational efficiency and quick start-up times. On the other hand, Solid Oxide Fuel Cells are typically used in stationary applications where higher efficiency at larger scales is needed. By shifting towards fuel cells as a primary means of energy production, we can harness cleaner emissions.
Understanding Carbon Capture Technology
Carbon capture technology is instrumental in the fight against climate change. It involves capturing carbon dioxide emissions at their source before they can enter the atmosphere. The three main capture methods are absorption, adsorption, and membrane separation. Each method employs distinct technologies to capture CO2.
- Absorption utilizes chemical reactions to take CO2 from flue gases.
- Adsorption relies on the physical adhesion of gas molecules to solid surfaces, and
- Membrane Separation uses selective barriers to separate CO2 from other gases.
Integrating these carbon capture methods with fuel cells may enhance the effectiveness of both technologies while reducing emissions. This combination increases overall energy efficiency by reducing the carbon footprint of energy production.
"The integration of fuel cells and carbon capture could revolutionize how we approach energy and environmental challenges."
"The integration of fuel cells and carbon capture could revolutionize how we approach energy and environmental challenges."
Through the understanding of both fuel cells and carbon capture, we can explore innovative solutions that meet energy demands while minimizing environmental impact.
Mechanisms of Fuel Cells
Understanding the mechanisms of fuel cells is essential to appreciate their role in the larger context of energy production and carbon capture. Fuel cells convert chemical energy directly into electrical energy through electrochemical reactions. They have distinct types, each with varying characteristics, efficiencies, and operational frameworks. This section will explore specific types of fuel cells and their operating principles, highlighting their importance in advanced energy solutions.
Types of Fuel Cells
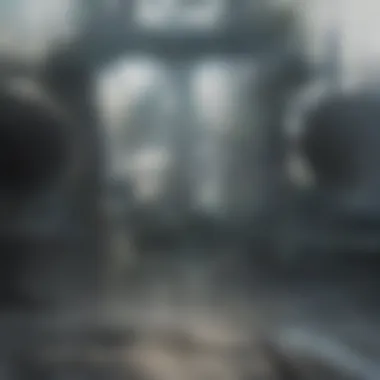
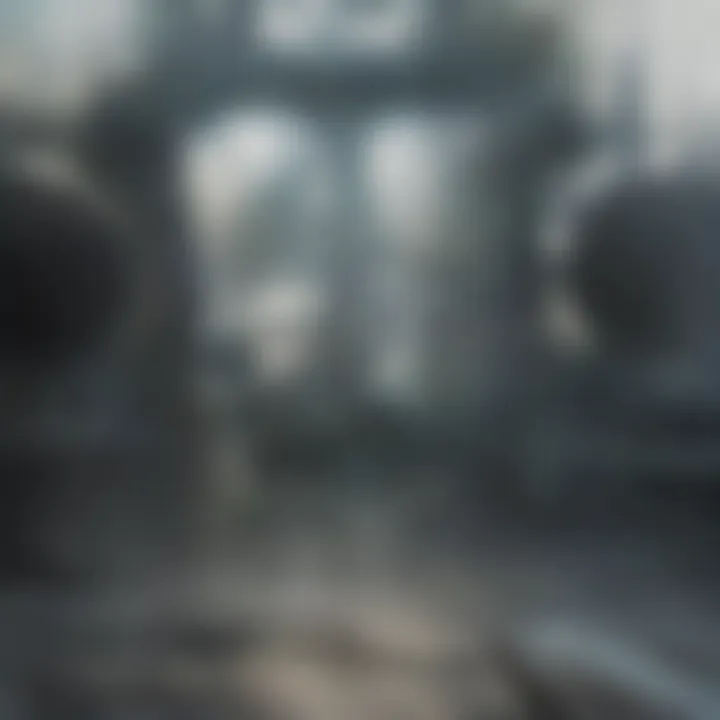
Proton Exchange Membrane Cells
Proton Exchange Membrane Cells (PEMFC) are notable for their efficiency and compact size. They utilize a polymer membrane to conduct protons while being impermeable to gases like hydrogen and oxygen. This characteristic makes them a favored choice in mobile applications such as vehicles. The key advantage of PEMFC is their ability to operate at relatively low temperatures, around 60 to 80 degrees Celsius, which facilitates quick start-up times. However, they are sensitivity to impurities in hydrogen fuel, which can affect their performance.
Solid Oxide Fuel Cells
Solid Oxide Fuel Cells (SOFC) operate at higher temperatures, typically between 600 and 1,000 degrees Celsius. They use a solid ceramic electrolyte to conduct oxygen ions. One of their main advantages is that they can run on a variety of fuels, including natural gas and biogas. This flexibility allows for substantial reductions in CO2 emissions when used with these fuels. However, their high operating temperatures lead to longer start-up times and the necessity for robust materials to withstand thermal stresses.
Alkaline Fuel Cells
Alkaline Fuel Cells (AFC) employ a liquid alkaline electrolyte. They have been utilized in space and military applications due to their reliability and efficiency in specific conditions. AFCs are more efficient than their counterparts (like PEMFC) at lower temperatures. However, they are susceptible to carbon dioxide in the atmosphere, which can form carbonate in the electrolyte, reducing overall effectiveness. This characteristic limits their applicability in certain environments, impacting their overall utility in broader energy contexts.
Operating Principles
Electrochemical Reactions
The foundation of fuel cell operation is electrochemical reactions. In these reactions, hydrogen molecules are split into protons and electrons. This separation occurs at the anode in the cell. The protons move through the electrolyte while the electrons travel through an external circuit, generating electricity. The oxygen from the air combines with the protons and electrons at the cathode, producing water as a by-product. This efficiency in converting chemical energy directly to electrical energy is a critical aspect that binds fuel cells to carbon capture technologies.
Energy Conversion Efficiency
Energy conversion efficiency is a hallmark of any fuel cell technology. Fuel cells can convert up to 60% of the energy in hydrogen to electrical power, depending on the type of cell and operating conditions. This aspect is crucial for the adoption of fuel cells in various sectors since higher efficiency can lead to more sustainable energy practices. However, efficiency can be affected by various factors, including system design and fuel quality. Understanding these efficiencies guides research and advancements in both fuel cell and carbon capture technologies.
Principles of Carbon Capture
The integration of carbon capture technology plays a crucial role in mitigating climate change. It aims to prevent carbon dioxide emissions from entering the atmosphere while promoting sustainable energy practices. Carbon capture has gained attention as a strategic solution to address global warming. By trapping carbon dioxide produced from industrial processes, this technology has the potential to significantly reduce the carbon footprint of various sectors. This section will cover different capture technologies, with a focus on their unique characteristics and how they enhance fuel cell systems.
Capture Technologies Overview
The effectiveness of carbon capture relies on several distinct technologies. Understanding these can provide insight into how they function and contribute to energy systems. The primary technologies discussed here include absorption, adsorption, and membrane separation. Each of these methods has its specific advantages and challenges.
Absorption
Absorption involves dissolving carbon dioxide in a solvent, usually a liquid. This method is considered standard in carbon capture systems due to its efficiency. The key characteristic of absorption is its capacity to handle large volumes of gas effectively. Its widespread use in industries is a testament to its reliability and success.
One notable feature of absorption is its ability to be tailored for specific gases, enhancing its overall efficacy. Among its advantages, absorption systems can achieve high capture rates under appropriate conditions. However, there are disadvantages as well. The process requires significant amounts of energy to regenerate the solvent for reuse, which can compromise overall energy efficiency.
Adsorption
Adsorption works on a different principle. It captures carbon dioxide by allowing it to adhere to the surface of solid materials, known as adsorbents. The primary feature of adsorption is the potential for low energy requirements compared to absorption methods. This makes it an appealing option for various applications.
A benefit of adsorption is its versatility; it can be utilized in both small and large-scale operations. However, adsorption can sometimes be less efficient in capturing low concentrations of carbon dioxide. The overall system design needs to be optimized carefully to address this challenge.
Membrane Separation
Membrane separation utilizes selective permeability to separate carbon dioxide from other gases. This method has been gaining traction due to its compact design and energy efficiency. The key aspect of membrane separation is its continuous operation, which can contribute to better performance over time.
Membrane technology has the unique characteristic of modular design, which allows it to be easily integrated into existing systems. The advantages include reduced footprint and operational flexibility. Nevertheless, the technology faces challenges, such as membrane degradation over time and the need for regular maintenance.
Integration of Carbon Capture with Fuel Cells
Integrating carbon capture with fuel cell systems can enhance both technologies' performance. By capturing emissions directly from fuel cell processes, significant reductions in greenhouse gases can be achieved. This integration helps in creating a more sustainable energy future. The combination of these technologies allows for cleaner energy production with a smaller environmental impact. Collaboration across industries will be vital to develop practices that maximize the benefits of this integration.
Effective carbon capture is not just a technology; it's an essential strategy in the fight against climate change.
Effective carbon capture is not just a technology; it's an essential strategy in the fight against climate change.
Synergistic Benefits of Fuel Cell Carbon Capture
The integration of fuel cells and carbon capture technologies serves multiple critical functions in today’s energy landscape. It is essential to understand the synergistic benefits that this integration provides. In a world increasingly focused on sustainability, these technologies can significantly transform energy production, carbon management, and economic stability. This section examines these benefits in detail.
Enhanced Energy Efficiency
Fuel cells offer a highly efficient method of converting fuel directly into electricity while producing minimal waste. When paired with carbon capture systems, the overall efficiency can improve further. The combined operation ensures that not only is energy generated with less loss, but harmful emissions are also reduced significantly.
Fuel cell systems have an efficiency range of 40% to 60%, which can rise dramatically when combined with heat recovery systems. By capturing waste heat, fuel cells can attain efficiencies of up to 90%. This results in a lower carbon footprint and better utilization of resources. Such enhanced efficiency is crucial in making the case for a transition towards cleaner energy.
Reduction of Greenhouse Gas Emissions
The environmental impact of blending fuel cells with carbon capture technologies cannot be understated. Traditional power generation methods, such as coal-fired plants, contribute significantly to greenhouse gas emissions. However, utilizing fuel cells not only reduces the emissions at the source but also effectively captures remaining carbon dioxide before it reaches the atmosphere.
Through carbon capture, facilities can potentially reduce emissions by as much as 90%. This reduction is instrumental in combating climate change and adhering to international obligations aimed at lowering atmospheric carbon levels.
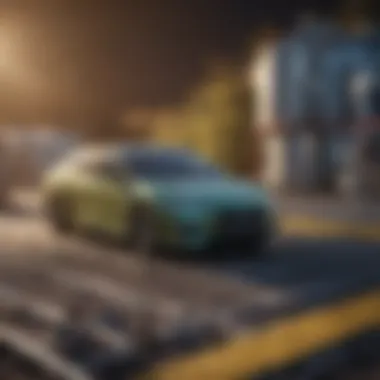
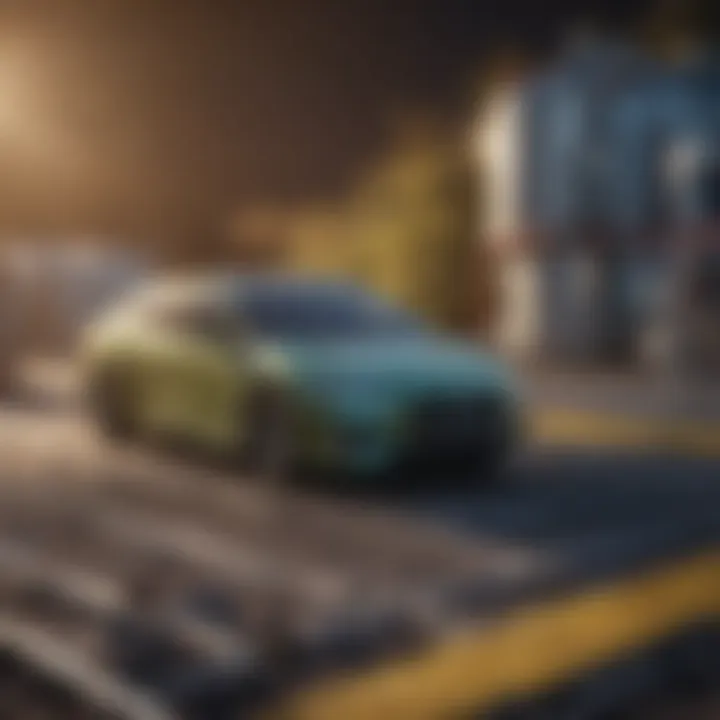
"Integrating carbon capture with fuel cells presents a vital opportunity to cut down emissions and lead the way towards a carbon-neutral future."
"Integrating carbon capture with fuel cells presents a vital opportunity to cut down emissions and lead the way towards a carbon-neutral future."
Economic Viability and Job Creation
The economic implications of fuel cell carbon capture technology are also noteworthy. As industries begin to adopt these technologies, they can expect to see a decrease in costs related to carbon emissions pricing and potential tax incentives for lowering emissions.
Moreover, the growth of this sector can foster job creation in several areas, such as research and development, manufacturing, and maintenance of these systems. New jobs will arise in high-demand fields. This transition supports not just an eco-friendly approach but also encourages economic growth by creating a market for green technologies.
Current Research and Innovations
The integration of fuel cells and carbon capture systems is increasingly essential in addressing climate change. Current research in these fields aims to unlock new potentials, enhance efficiency, and reduce costs. Innovations in fuel cell technologies can lead to improved energy output and reliability. Similarly, advancements in carbon capture methods can facilitate more effective CO2 reduction.
Recent Advancements in Fuel Cell Technology
Fuel cell technology has seen significant progress in recent years. Researchers focus on improving the durability and efficiency of fuel cells, particularly Proton Exchange Membrane Fuel Cells (PEMFCs) and Solid Oxide Fuel Cells (SOFCs). New materials and designs for electrodes and membranes are under investigation to optimize performance.
For example, the development of nanoscale catalysts shows promise for increasing reaction speeds and reducing dependence on precious metals like platinum. Enhanced thermal management systems also help maintain optimal operating temperatures, thereby improving overall efficiency. Furthermore, advances in hybrid systems, which combine fuel cells with renewable sources, are paving the way for sustainable energy solutions.
Emerging Carbon Capture Methods
In the realm of carbon capture, new techniques are emerging, enhancing the feasibility of capturing CO2 effectively. One notable approach is the implementation of new absorbing materials that exhibit higher selectivity and lower energy consumption. These materials can capture carbon from industrial processes more efficiently.
Another area of innovation is direct air capture technology. This method involves creating systems that can draw CO2 directly from the atmosphere. As the technology advances, it may contribute significantly to atmospheric CO2 reduction alongside traditional carbon capture methods.
"The synergy between advancements in fuel cells and emerging carbon capture technologies offers a dual approach towards our sustainability goals."
"The synergy between advancements in fuel cells and emerging carbon capture technologies offers a dual approach towards our sustainability goals."
In summary, ongoing research and innovations in fuel cells and carbon capture technology can address critical environmental challenges. Continued investment and development in these areas are crucial for effective climate change mitigation.
Challenges in Implementation
The integration of fuel cells and carbon capture technologies represents a promising step toward sustainable energy systems. However, several challenges hinder the widespread implementation of these systems. Addressing these challenges is crucial for realizing the full potential of fuel cell carbon capture solutions. The combined intricacies of technical limitations, economic barriers, and regulatory challenges must be carefully examined to facilitate progress in this field.
Technical Limitations
Understanding the limitations of current fuel cell and carbon capture technologies is essential for advancing their integration. One major technical limitation is the efficiency of carbon capture systems when used with fuel cells. Existing capture technologies may not operate optimally at the varying temperatures and pressures found in fuel cell applications.
Another limitation is the durability and lifespan of fuel cells. Fuel cells can degrade over time due to factors such as impurities in the fuel or operational stress. This degradation can impact the overall efficiency and performance of the system. Moreover, many carbon capture methods require significant energy input, which can reduce the overall energy efficiency of fuel cells if not managed properly.
Economic Barriers
Economic factors also pose significant challenges to the implementation of fuel cell carbon capture technologies. The initial investment required for developing and deploying these systems is substantial. For many industries, particularly smaller companies, the high capital cost can deter investment.
Additionally, the operation and maintenance costs of these systems can be a barrier. While the promise of lower emissions and energy efficiency is appealing, the economic returns may not be immediately evident. This situation prompts the need for incentives and funding from governmental or private entities to encourage investment. Furthermore, market fluctuations can affect the cost-effectiveness of fuel cells and capture technologies, adding to the uncertainty of economic viability.
Regulatory and Policy Issues
The regulatory landscape plays a vital role in shaping the development and deployment of fuel cell carbon capture systems. Diverse regulations across jurisdictions create uncertainty for businesses. Without clear guidelines, companies may hesitate to invest in new technologies due to concerns over compliance and future regulatory changes.
Policy frameworks are often slow to adapt to rapid technological advancements. In some cases, existing laws may not adequately reward or support the integration of fuel cells and carbon capture systems. For example, emissions trading systems and tax incentives may lack mechanisms specifically designed for these technologies.
The success of these technologies hinges on overcoming technical limitations, addressing economic barriers, and navigating the regulatory landscape effectively.
The success of these technologies hinges on overcoming technical limitations, addressing economic barriers, and navigating the regulatory landscape effectively.
Only through collaborative efforts can these challenges be effectively addressed, paving the way for a sustainable energy future.
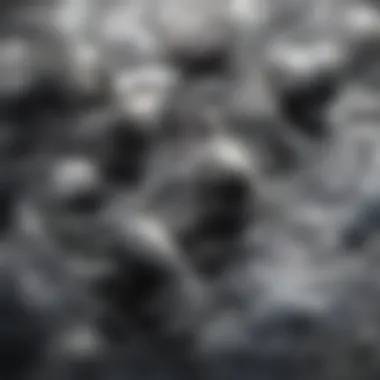
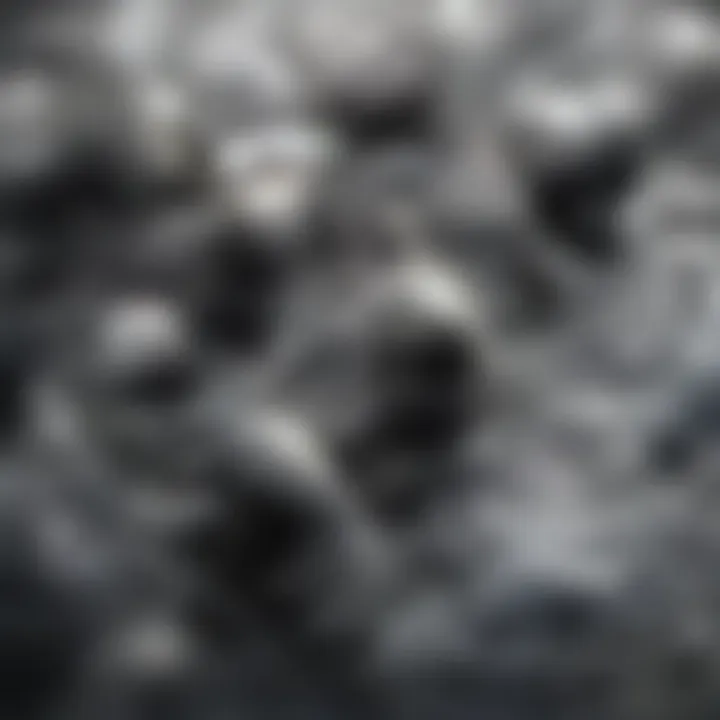
Case Studies of Fuel Cell Carbon Capture Applications
The application of fuel cell carbon capture technologies proves critical for implementing solutions to climate change across various sectors. These case studies provide tangible examples of how these technologies function in real-world scenarios. They highlight the importance of adaptation to local contexts while demonstrating the benefits associated with integrating both fuel cells and carbon capture systems.
Industrial Applications
Industries are among the largest contributors to greenhouse gas emissions. Consequently, they hold significant potential for impact when it comes to fuel cell carbon capture implementation. For example, the cement manufacturing sector is examining how it can incorporate fuel cells with carbon capture. In these processes, fuel cells can generate electricity while capturing carbon dioxide emitted during the cement production phase.
Benefits of this integration include:
- Energy Efficiency: Fuel cells operate at a higher energy efficiency than standard combustion methods. This efficiency can lower the overall energy demand from industries.
- Reduced Emissions: By capturing carbon dioxide directly at the source, industries can drastically cut emissions without needing extensive overhauls of their existing infrastructure.
- Cost Savings: Many industries may face economic pressure to reduce their carbon footprint. The combination of fuel cells with carbon capture can offer pathways to financial savings over time while complying with stricter regulations.
Transportation Sector Innovations
In the transportation sector, fuel cells can revolutionize the way we approach travel and freight. Case studies from companies like Hydrogenics illustrate how fuel cells paired with carbon capture can lower emissions significantly in vehicles and shipping.
For example, heavy-duty trucks powered by hydrogen fuel cells emit only water vapor. However, when these vehicles are paired with efficient carbon capture technology, they can mitigate the minor emissions they do produce in operation. Such innovations present a dual approach: reducing emissions while providing clean energy for the vehicles themselves.
Key points include:
- Decarbonization of Transportation: As governments push for cleaner public transport, fuel cells can provide a sustainable alternative. This move is crucial considering the transport sector is a major contributor to pollution.
- Infrastructure Development: Investing in hydrogen refueling stations alongside carbon capture facilities allows for a more integrated energy system, making it easier for consumers to transition to hydrogen-powered vehicles.
- Data Collection and Analysis: These case studies also allow researchers to gather vital data on efficiency and overall performance, pointing to areas needing improvement.
"Integrating fuel cells with carbon capture systems offers one of the strategic methodologies to mitigate climate impact while enhancing energy efficiency in powerful industrial and transportation applications."
"Integrating fuel cells with carbon capture systems offers one of the strategic methodologies to mitigate climate impact while enhancing energy efficiency in powerful industrial and transportation applications."
Overall, these examples underlie the need for continued collaboration between stakeholders in various sectors. The case studies present ample opportunities to refine technology and align market needs with sustainability goals.
Future Prospects and Directions
The integration of fuel cells with carbon capture technologies presents a compelling avenue in the quest for sustainable energy solutions. As concerns about climate change escalate, understanding the future prospects and directions of these technologies is vital. Their combined potential could significantly influence energy production and greenhouse gas reductions.
Potential for Large-Scale Implementation
For fuel cell carbon capture to be effective on a larger scale, several factors come into play. First is the scalability of the technologies involved. Fuel cells must demonstrate the ability to provide stable and ample energy supply while integrating carbon capture systems.
Secondly, developing robust infrastructure is essential. This includes enhancing transportation systems for hydrogen and implementing facilities for carbon dioxide storage and utilization. Moreover, optimizing the efficiency of both fuel cells and CO2 capture mechanisms will maximize economic and environmental benefits.
Several industries can benefit from large-scale deployment. For instance, the power sector could reduce emissions dramatically by retrofitting existing plants with both technologies. Additionally, sectors such as transportation can transition to cleaner fuels, powered by fuel cells using captured carbon.
Research and Development Priorities
To unlock the full potential of fuel cell carbon capture, focused research and development efforts are necessary. Key priorities include:
- Innovating Materials: Researchers should work to identify more effective materials for fuel cells and carbon capture systems that enhance performance and durability.
- Cost-Reduction Strategies: Development must prioritize lowering the costs associated with both technologies. This may include exploring new manufacturing processes or alternative materials that are less expensive.
- Integrated System Design: There is a need for holistic designs that consider the interaction between fuel cells and carbon capture components. This can lead to improved efficiencies and lower overall costs.
"The successful integration of fuel cells with carbon capture technologies could redefine our approaches to reducing carbon emissions across various industries."
"The successful integration of fuel cells with carbon capture technologies could redefine our approaches to reducing carbon emissions across various industries."
Epilogue
The integration of fuel cells and carbon capture technologies is an essential topic in today's discussions about climate change and energy sustainability. The benefits of these technologies are profound, impacting not just emissions reduction but also energy efficiency, economic growth, and social responsibility.
Summarizing Key Takeaways
- Reduction in Carbon Emissions: Fuel cell technology offers a cleaner alternative to traditional fossil fuels. By integrating with carbon capture systems, it can help trap significant amounts of CO2, thus lowering the overall greenhouse gas emissions from energy production.
- Enhanced Energy Efficiency: Fuel cells convert hydrogen and oxygen into electricity with higher efficiency than combustion methods. When coupled with carbon capture, the efficiency gains can lead to better resource use and lower operational costs.
- Synergistic Advantages: The combination of these technologies provides dual benefits. Not only do they improve energy production, but they also help transition society towards sustainable energy practices.
- Economic Potential: Investing in fuel cell carbon capture can stimulate economic growth through job creation in research, manufacturing, and installation sectors.
- Research and Innovation: Ongoing research is vital for overcoming current technological barriers and improving the effectiveness of carbon capture processes.
Call for Collaborative Efforts
Addressing climate change and energy demands will require coordinated efforts across industries, governments, and research institutions. Collaborative projects can harness diverse skill sets and resources, driving innovation in fuel cell carbon capture technologies. It is crucial to:
- Encourage Public-Private Partnerships: Government incentives are necessary to promote investments in this area.
- Foster Academic Collaborations: Universities and research institutions must work together to advance knowledge and develop new carbon capture methods.
- Engage the Community: Public awareness campaigns can enhance understanding of the technologies and their benefits, leading to greater support.
In summary, the potential of fuel cell carbon capture is vast and merits further exploration and commitment. Collaborative efforts can ensure that these technologies become a cornerstone of sustainable energy solutions.