Genome-Wide CRISPR Screening: Insights and Applications
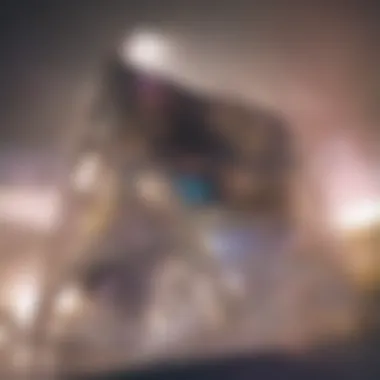
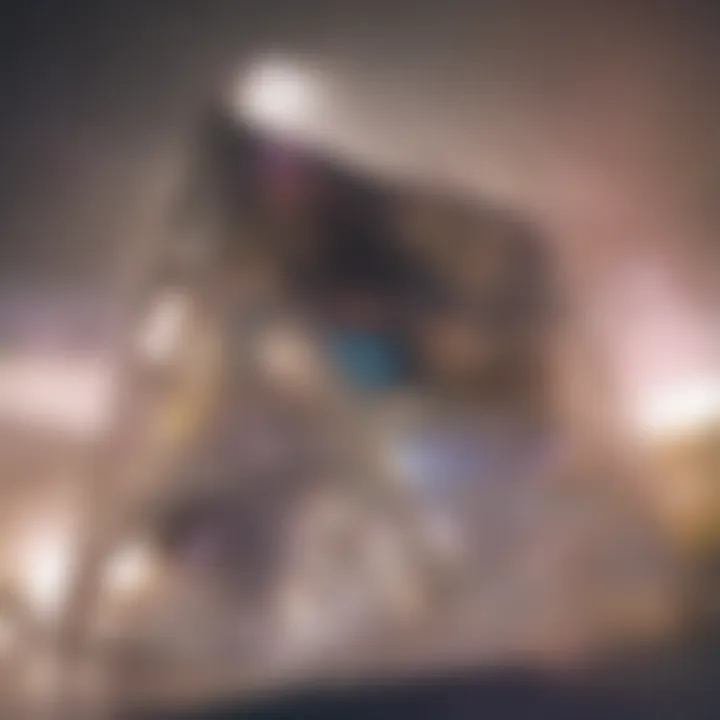
Intro
Genome-wide CRISPR screens represent a significant leap forward in genetic research and biotechnology. This method utilizes CRISPR technology to manipulate genes on a large scale, allowing researchers to identify and analyze functional elements within the genome. The process can reveal the roles of specific genes in biological pathways, disease processes, and therapeutic targets.
Understanding the intricacies of genome-wide CRISPR screens is essential for those involved in genetics, molecular biology, and related disciplines. This comprehensive exploration will provide insights into the methodologies, applications, and challenges associated with this powerful tool.
Article Overview
Purpose of the Article
The primary aim of this article is to dissect genome-wide CRISPR screens thoroughly. It will cover the methodologies used in these screens, the innovative technologies behind CRISPR, and their various applications across multiple scientific domains. By doing so, it highlights both the transformative nature of this technology and the fundamental changes it brings to genetic research.
Relevance to Multiple Disciplines
Genome-wide CRISPR screens hold significance not only in genetics but also in fields such as cancer research, drug development, and cell biology. Their utility spans various aspects of research and development, making them applicable to a wide array of scientific inquiries. This versatility is a cornerstone of the ongoing fascination with CRISPR technology.
Research Background
Historical Context
CRISPR technology has its roots in bacterial defense mechanisms. Initially identified in the late 1980s, its gene-editing capabilities were first harnessed in mammalian cells around 2012. Since then, it has rapidly evolved, paving the way for applications in genome-wide screens. These screens emerged as powerful approaches in basic and applied research, enabling systematic exploration of gene function on an unprecedented scale.
Key Concepts and Definitions
- CRISPR: Clustered Regularly Interspaced Short Palindromic Repeats, a system used by bacteria to defend against viruses, which has been repurposed for gene editing.
- Genome-Wide Screening: A method that tests multiple genes at once to discover their functions, interactions, and contributions to specific biological outcomes.
- Guide RNA (gRNA): A component of CRISPR that directs the Cas9 enzyme to a specific DNA sequence for editing.
These concepts form the backbone of genome-wide CRISPR screens, allowing scientists to undertake complex genetic experiments.
"Genome-wide CRISPR screens provide a way to systematically and comprehensively understand gene functions and interactions within the broader context of cellular networks."
"Genome-wide CRISPR screens provide a way to systematically and comprehensively understand gene functions and interactions within the broader context of cellular networks."
Prelims to Genome-Wide CRISPR Screens
Genome-wide CRISPR screens have emerged as a transformative tool in genetic research, offering unparalleled insights into gene function and interaction. This section delves into the significance of these screens within the broader context of genetic investigation. By facilitating the systematic analysis of gene roles, CRISPR technology not only enhances our understanding of cellular mechanisms but also opens avenues for therapeutic advancements.
Overview of CRISPR Technology
CRISPR, an acronym for Clustered Regularly Interspaced Short Palindromic Repeats, is a groundbreaking technology developed from a microbial immune system. Researchers have exploited this natural defense mechanism to create a precise editing tool for genomic DNA. The CRISPR-Cas9 system is the most recognized variant, wherein the Cas9 protein operates as molecular scissors, enabling targeted cuts in DNA facilitated by guide RNA (gRNA).
The versatility of CRISPR technology lies in its ability to modify genes at specified sequences, offering significant advantages over traditional genetic manipulation techniques. Its capacity for high-throughput applications demonstrates its potential impact on understanding complex biological systems and developing novel therapies. Recent advancements in CRISPR technology further emphasize its importance in genome-wide screening. This technology provides researchers with a reliable method to explore how genes contribute to various biological processes, thus driving forward the field of genomics.
Defining Genome-Wide Screening
Genome-wide screening refers to the comprehensive examination of genes across the entire genome to identify those that affect a particular phenotype or biological pathway. In the context of CRISPR, this involves designing libraries of gRNAs that target a wide range of genes. The objective is to systematically disrupt genes to observe resultant changes in cellular behavior or function.
This approach allows for the identification of essential genes associated with diseases such as cancer, neurodegeneration, and genetic disorders. By employing genome-wide CRISPR screens, researchers can prioritize gene targets for further exploration, providing a strategic advantage in therapeutic discovery. Moreover, this methodology underscores the necessity of a multidisciplinary approach, incorporating bioinformatics, molecular biology, and computational analysis, to fully harness the power of CRISPR technology.
Mechanisms of CRISPR
The mechanisms underlying CRISPR technology are essential for understanding its transformative role in genetic research. These processes are not only fundamental to how CRISPR functions, but they also highlight the adaptability and potential applications of this revolutionary technique. A comprehensive grasp of these mechanisms will enable researchers to tailor CRISPR systems to meet specific experimental needs.
CRISPR-Cas Systems
The CRISPR-Cas system is a sophisticated adaptive immune response in prokaryotes, allowing organisms to defend themselves against foreign genetic material. This system consists of two main components: the CRISPR arrays and Cas proteins. The CRISPR arrays are segments of DNA that contain short, repetitive sequences interspersed with snippets of viral DNA, known as protospacers. The Cas proteins are essential for recognizing these sequences and executing the necessary response when foreign elements invade.
The versatility of CRISPR-Cas systems, particularly the Cas9 protein, has made it a preferred tool for gene editing. Cas9 acts as molecular scissors, creating double-strand breaks in targeted DNA sequences. Following the modification, the cell's repair machinery intervenes, which can lead to either non-homologous end joining or homology-directed repair. This mechanism facilitates targeted gene knockout or insertion, allowing for precise modifications in an organism’s genome, which is advantageous in various applications including research and therapeutics.
Target Identification and Modification
Target identification and modification are critical steps in genome-wide CRISPR screening processes. Properly identifying the target genes is essential for successful experiments. A well-designed guide RNA (gRNA) is necessary to ensure specificity, minimizing off-target effects. This can be challenging due to the complex nature of genomes and the potential for unintended modifications.
Once targets are identified, the modification process begins. By introducing the gRNA along with the Cas enzymes into target cells, researchers can achieve precise edits. These modifications can include gene knockouts or insertions, depending on the desired outcomes of the research or therapeutic applications.
The focus on target identification and modification has significant implications. Understanding the best practices for designing gRNAs can vastly improve the success rate of CRISPR projects. In turn, this knowledge allows for advancements in areas such as disease modeling and drug development. By skillfully navigating these processes, scientists can harness the full power of CRISPR to interrogate gene functions and explore new therapeutic avenues.
"The efficiency and precision of CRISPR technology depend significantly on the accuracy of target identification and the quality of modifications implemented during experiments."
"The efficiency and precision of CRISPR technology depend significantly on the accuracy of target identification and the quality of modifications implemented during experiments."
As such, researchers must continuously refine their techniques in target identification and modification to maximize the benefit of genome-wide CRISPR screens. This ongoing progression is vital for pushing the boundaries of current genetic research.
Designing Genome-Wide CRISPR Screens
Designing genome-wide CRISPR screens is a critical aspect that impacts the success of genetic research and therapeutics. The design process determines the effectiveness and accuracy of the screening results. A well-structured design can uncover essential biological insights and assist in the development of targeted therapies. Several specific elements must be considered, including the choice of gRNA libraries, selection of appropriate cell lines, and the methodology of screening employed.
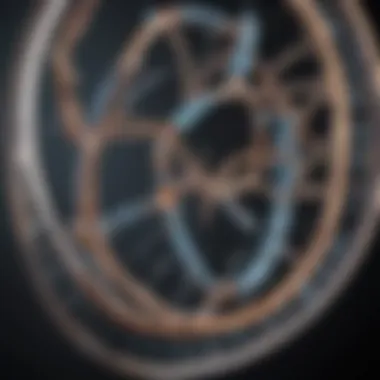
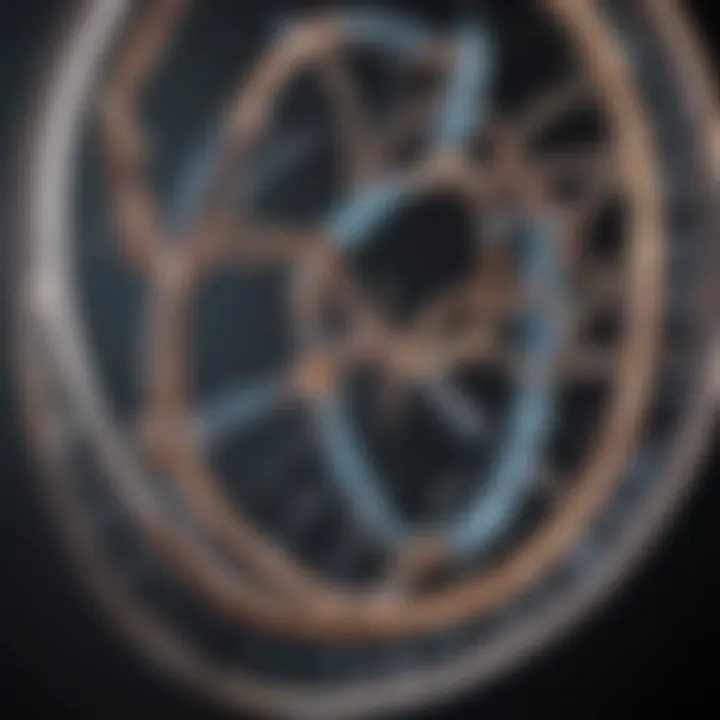
In this article, the benefits of thorough design will be emphasized. Appropriate design ensures a comprehensive coverage of the genome and improves the detection of relevant gene functions. Furthermore, careful design can mitigate challenges such as off-target effects and ensure reliable data collection. Ultimately, a meticulous approach to designing these screens can lead to significant advancements in various fields, such as cancer research and drug development.
gRNA Library Construction
The construction of gRNA libraries is foundational to genome-wide CRISPR screens. These libraries serve as the tools used to target specific genes across the genome. A crucial step in library construction is ensuring that the gRNAs are designed to provide dense coverage of the target genome without duplicacy. This requires an understanding of the genome's structure and the selection of guide sequences that minimize off-target effects.
Once the gRNA libraries are constructed, it is essential to validate their efficacy. This validation process ensures that the gRNAs effectively bind to their target genes and facilitate the desired gene editing. Through systematic testing and optimization, researchers can establish a library that maximizes efficiency in the screening process.
Screening Approaches
The choice of screening approach significantly influences the outcomes of CRISPR screens. Each approach offers distinct advantages and limitations.
Forward Genetics Approach
The forward genetics approach focuses on identifying genes responsible for a particular phenotype. This method is characterized by starting with a phenotypic observation and working backward to determine the underlying genetic factors. Its basic principle lies in the random integration of gRNAs into the genome, allowing for the investigation of genetic contributions to specific traits.
This approach is beneficial for exploratory studies because it is less hypothesis-driven. Researchers can discover unexpected genetic interactions and pathways. However, the forward genetics approach may have limitations, such as the need for extensive downstream validation to trace back from phenotype to genotype, which can be time-consuming.
Reverse Genetics Approach
In contrast, the reverse genetics approach begins with specific gene targets. Here, researchers aim to analyze the effects of knocking out or modifying already identified genes. This method utilizes pre-determined gRNAs designed against target sequences, making it a focused approach.
The key characteristic of the reverse genetics approach is its efficiency in studying known genes. It allows researchers to test hypotheses quickly and focus on genes of interest. One unique feature is that it can facilitate targeted modifications, providing clarity regarding the function of specific genes. However, this approach may miss broader genetic interactions that could be revealed by more exploratory methods, potentially limiting discoveries from unexpected areas.
"The choice of screening approach can dictate the findings of genome-wide CRISPR screens, emphasizing the need for careful consideration of the objectives of the research project."
"The choice of screening approach can dictate the findings of genome-wide CRISPR screens, emphasizing the need for careful consideration of the objectives of the research project."
Implementation of CRISPR Screens
The implementation of genome-wide CRISPR screens is a critical phase in harnessing the full potential of CRISPR technology for biological research. This stage involves several key factors that influence the success and accuracy of the screening process. First, selecting appropriate cell lines is essential. The choice of these cell lines can affect the representativeness of the screen results. Furthermore, efficient transduction methods are required to ensure that the gRNA libraries are effectively introduced into target cells. Lastly, robust data collection methodologies must be established to accurately capture and analyze the outcomes of the screens.
This section delves into these elements and highlights their significance in the broader context of genome-wide CRISPR applications. Understanding these specifics can aid researchers in optimizing their approaches and achieving meaningful results.
Cell Line Selection
Selecting the appropriate cell line is foundational for successful CRISPR screening. Various factors must be taken into account to make an informed decision. The biological context of the study, including the type of cancer or disease being investigated, can dictate the choice of cell line.
Some considerations include:
- Relevance: Is the cell line representative of the tissue or condition being studied?
- Growth Characteristics: Does the cell line have growth and survival rates that are conducive to screening?
- Genetic Background: Does it possess genetic mutations or features relevant to the research question?
The consequences of poor cell line selection can lead to misleading results. Thus, aligning the choice with the specific goals of the research is vital for drawing accurate conclusions from the screening outcomes.
Transduction Efficiency
Transduction efficiency is another critical component that determines the overall effectiveness of CRISPR screens. It refers to how well the gRNA is introduced into the target cells. Low transduction efficiency can result in insufficient representation of the gRNA library, leading to incomplete data and unreliable results. Key factors affecting transduction include:
- Viral Vectors: The choice of viral delivery system, such as lentivirus or adenovirus, impacts efficiency.
- MOI (Multiplicity of Infection): The ratio of infectious agents to cells can influence how many cells acquire a gRNA.
- Optimization of Protocols: Tailoring the transduction protocol to the specific cell type can yield better results.
Ensuring high transduction efficiency allows for a more comprehensive evaluation of gene function. By maximizing the number of cells that receive the CRISPR constructs, researchers can improve the depth of their analysis and identify critical gene interactions.
Data Collection Methodologies
The methodologies used for data collection during CRISPR screens play a significant role in the integrity and clarity of the findings. Implementing structured protocols for data capture is paramount. Different techniques may be employed depending on the objectives of the research. Some common methodologies include:
- High Throughput Sequencing: This allows for the comprehensive analysis of gRNA distribution across the cell population.
- Flow Cytometry: This enables the quantification of cells expressing certain markers, which can provide insights into the impact of specific gene edits.
- Cell Viability Assays: These tests help determine the functional consequences of CRISPR interventions on cell survival and proliferation.
The integration of these methodologies facilitates a robust analysis pipeline that helps distill complex datasets into actionable insights. Accurate data collection is vital for validating hypotheses and guiding subsequent experimental plans.
"A successful implementation of CRISPR screens hinges on meticulous attention to the nuances of cell line selection, transduction efficiency, and data collection methodologies. A slight oversight in any of these areas can cascade into significant challenges in interpreting results."
"A successful implementation of CRISPR screens hinges on meticulous attention to the nuances of cell line selection, transduction efficiency, and data collection methodologies. A slight oversight in any of these areas can cascade into significant challenges in interpreting results."
In summary, the successful implementation of genome-wide CRISPR screens requires a careful approach to various interdependent factors. Through diligent cell line selection, optimized transduction methods, and stringent data collection practices, researchers can leverage CRISPR technology to unlock new discoveries in genetics and beyond.
Analysis of CRISPR Screen Data
The analysis of CRISPR screen data is fundamental to understanding the results of any genome-wide CRISPR screening. After the execution of a screen, a vast amount of data is generated, which is essential for deriving meaningful biological insights. The significance of data analysis cannot be overstated; it helps researchers identify which genes are involved in a specific biological process and how they might interact with other genetic components. Consequently, this analysis is critical for connecting experimental outcomes to theoretical frameworks in genetics and molecular biology.
Careful data analysis allows researchers to filter noise and focus on significant signals from the background. Benefits of effective data analysis include improved hit identification, reduced errors in interpretation, and the ability to perform extensive validations. As genome-wide CRISPR screens gather substantial datasets, the complexity of data necessitates the use of advanced tools and methodologies.
Bioinformatics Tools
Bioinformatics tools play a pivotal role in the data analysis of CRISPR screens. These tools aid in processing and visualizing the massive datasets generated during experiments.
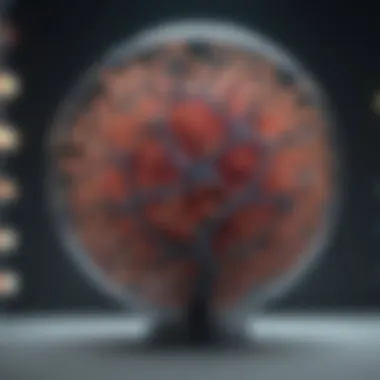
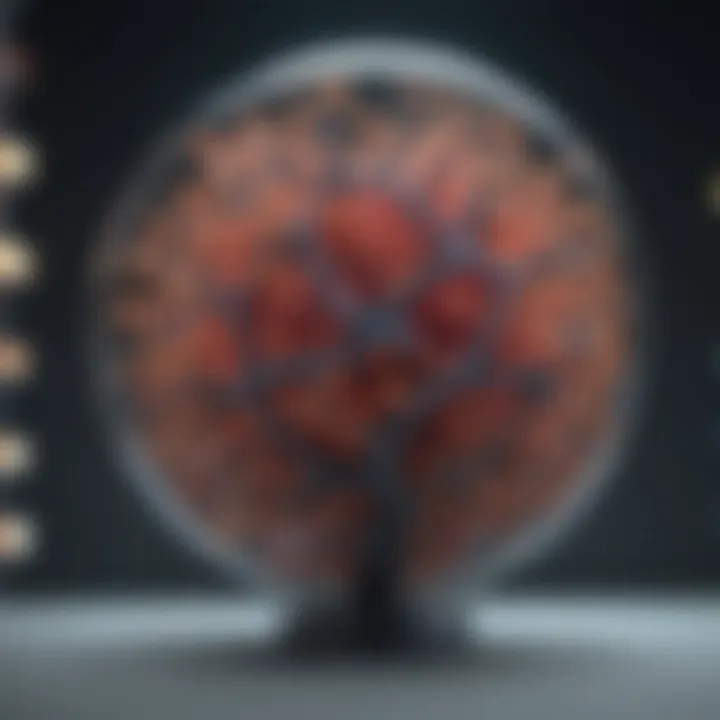
A few key bioinformatics tools include:
- MAGeCK: This software is designed for analyzing CRISPR screen data, providing functionalities to identify significant gene hits.
- CRISPRAnalyzeR: This tool helps researchers to analyze results from CRISPR screens through a comprehensive statistical framework.
- R and Bioconductor: For advanced users, R language provides extensive packages that allow high-throughput data analysis.
Moreover, data visualization is crucial. Visual representations help in pinpointing patterns and trends that may be overlooked in numerical formats. Heatmaps, scatter plots, and volcano plots are commonly used to depict results clearly and intuitively.
Statistical Approaches
Statistical approaches are essential to ensure that the findings from CRISPR screens are robust and reliable. Researchers often use various statistical models to address challenges such as batch effects and the inherent noise in biological data.
Common statistical methods include:
- Z-score Analysis: This method helps to identify outliers among the hit genes, allowing researchers to discern which results are statistically significant.
- False Discovery Rate (FDR) Control: This strategy mitigates the risk of false positives, which is crucial given the large datasets typical of genome-wide screens.
- Bayesian Approaches: These techniques can incorporate prior knowledge and improve the interpretation of results by modeling uncertainty.
By employing these methods, researchers can produce reliable conclusions from potentially overwhelming data, enhancing the validity of their findings.
Validation of Hits
Validation of hits is a crucial step following data analysis in CRISPR screens. It ensures that the identified gene targets genuinely affect the biological processes under study. Validation is often accomplished through secondary experiments, which could involve various techniques such as:
- Western Blotting: To confirm the protein levels of hit genes, providing information about gene expression.
- qPCR: Quantitative PCR allows the precise measurement of RNA levels, validating the involvement of specific genes in the observed phenotype.
- Functional Assays: These experiments assess the biological impact of the identified targets, validating their roles in the relevant pathways.
Ultimately, successful validation provides confidence in the findings and promotes further investigation into potential therapeutic applications or basic research avenues.
Applications of Genome-Wide CRISPR Screens
The applications of genome-wide CRISPR screens are vast and are transforming many fields of biological research. This section delves into pivotal applications that illustrate how these screens not only advance scientific understanding but also foster tangible innovations in medicine, drug development, and disease modeling. The integration of CRISPR technology facilitates precise gene editing and exploration of functional genomics, thereby addressing various challenges in contemporary biology.
Cancer Research
Cancer research stands at the forefront of CRISPR applications. Genome-wide CRISPR screenings enable the identification of essential genes that contribute to cancer cell proliferation, metastasis, and resistance to therapies. Researchers can systematically target genes across the genome to discover potential vulnerabilities in cancer cells. This method pinpoints genes that, when disrupted, result in reduced cell viability or altered responses to treatment. The insights gained from these screens aid in understanding tumor heterogeneity and uncover novel therapeutic targets. By using CRISPR to dissect complex signaling pathways, scientists can enhance the design of personalized cancer therapies tailored to individual patient profiles.
Drug Development
In drug development, genome-wide CRISPR screens streamline the process of discovering new drug targets. The ability to knock out or activate genes across the genome allows researchers to evaluate how specific genetic alterations impact drug sensitivity. Consequently, this informs the selection of compounds to test against particular cancer types. Moreover, CRISPR technology helps in creating more efficient pharmacogenomic models. By mapping the genetic factors that influence drug response, researchers can elucidate mechanisms of drug action and resistance, thus facilitating the development of more effective therapeutics.
Study of Gene Function
Understanding gene function is essential in genetics, molecular biology, and biomedicine. Genome-wide CRISPR screens provide a powerful platform to investigate the roles of thousands of genes systematically. By employing targeted gRNAs, researchers can classify genes based on their functions within specific biological processes. This exploration enhances gene annotation in databases, which is critical for both fundamental research and for understanding gene interactions within biological systems. Through these screens, it has become possible to identify previously unknown gene functions, thus adding depth to our understanding of cellular mechanisms.
Modeling Genetic Diseases
Modeling genetic diseases is another critical application of genome-wide CRISPR screens. By generating cellular models that reflect the mutations found in human genetic disorders, researchers can explore disease mechanisms at a molecular level. Such models serve as platforms for testing potential therapies. For example, in conditions like muscular dystrophy or cystic fibrosis, CRISPR screens can identify compensatory pathways that can be targeted therapeutically. This translates basic research into potential clinical applications, bridging the gap between bench and bedside.
The transformative potential of genome-wide CRISPR screens lies in their ability to democratize genetic research, making it accessible and applicable across various scientific domains.
The transformative potential of genome-wide CRISPR screens lies in their ability to democratize genetic research, making it accessible and applicable across various scientific domains.
In summary, the applications of genome-wide CRISPR screens reshape how researchers approach some of the most pressing questions in biology and medicine. From unraveling the complexities of cancer to discovering new drugs and elucidating gene functions, the versatility of CRISPR technology is pivotal in pushing the boundaries of what is known in the field.
Challenges in Genome-Wide CRISPR Screening
In the realm of genome-wide CRISPR screening, various challenges arise that can significantly impact the outcomes and interpretations of experiments. Addressing these challenges is crucial for researchers aiming to derive meaningful insights from their screens. Two significant challenges include off-target effects and the complexity of biological systems. Each of these factors plays a substantial role in influencing the reliability and effectiveness of CRISPR technologies in genetic research.
Off-target Effects
Off-target effects are unintentional modifications to the genome that occur when CRISPR components bind to sequences other than the intended target. This phenomenon can lead to misleading results, complicating data analysis and interpretation. The specificity of CRISPR systems is pivotal; thus, understanding the conditions that lead to off-target effects is essential for improving screening accuracy. A careful formulation of guide RNAs (gRNAs) is required to minimize these risks.
Researchers utilize several strategies to reduce off-target effects:
- Optimizing gRNA design: Employing algorithms that predict possible off-target sites is one effective approach. This helps in selecting gRNAs that are more likely to bind only at intended locations.
- Using high-fidelity Cas proteins: Variants such as Cas9 engineered for higher specificity can significantly reduce off-target modifications.
- Comprehensive validation: Performing deep sequencing can help identify unintended modifications, allowing researchers to confirm their findings and assess the off-target potential of the gRNAs used.
The management of off-target effects directly enhances the reliability of data obtained from CRISPR screening methodologies.
Complexity of Biological Systems
The intricate nature of biological systems presents another significant challenge in genome-wide CRISPR screening. Mammalian cells operate within vast and complex pathways where various molecular interactions occur simultaneously. The outcomes of CRISPR screens can alter based on the biological context in which they are performed. Understanding how environmental factors and cellular states affect gene expression is essential to accurately interpreting results.
To address this complexity, researchers must consider:
- Cell type selection: Using specific cell lines relevant to the study can yield more biologically meaningful results.
- System-level analysis: It may require an integrative approach, combining CRISPR technology with additional biological data to elucidate interactions more effectively.
- Replicate experiments: Conducting multiple independent assays helps to ensure that results are reproducible and not merely artifacts of a particular cellular context.
Ultimately, recognizing and overcoming these challenges enables researchers to better harness CRISPR technology for impactful discoveries in genetic research and therapeutic applications.
"The ability to accurately assess and mitigate challenges such as off-target effects and biological complexity will define the future success of CRISPR methodologies in genomics."
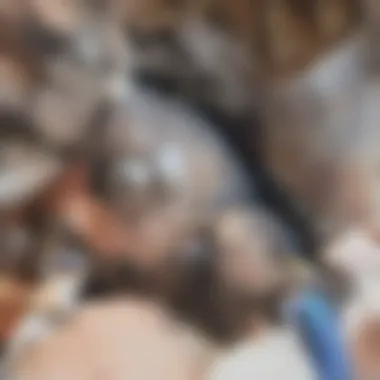
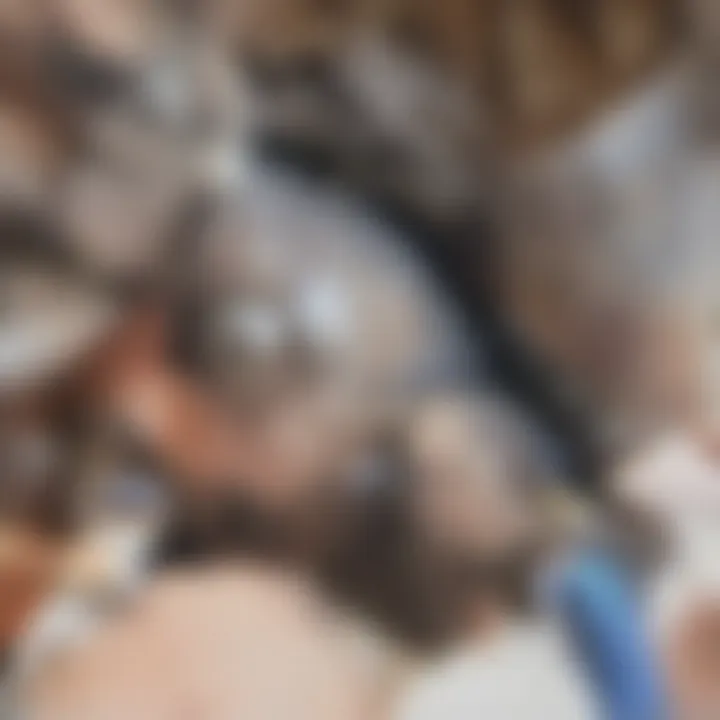
"The ability to accurately assess and mitigate challenges such as off-target effects and biological complexity will define the future success of CRISPR methodologies in genomics."
Future Directions in CRISPR Technology
The field of CRISPR technology continues to evolve rapidly, presenting new avenues of research and application. Understanding future directions is essential for scientists, researchers, and educators. As CRISPR technologies become more sophisticated, their potential extends beyond simple gene editing. Key areas of focus include enhancing specificity and expanding applications beyond genomics, which are vital for advancing both scientific understanding and therapeutic possibilities.
Enhancement of Specificity
One major consideration in CRISPR technology is the enhancement of specificity. Current CRISPR systems, while revolutionary, can still suffer from off-target effects. These unintended changes to the genome can lead to unpredictable consequences, which is particularly concerning in clinical applications.
Developing more refined gRNAs is one approach researchers are exploring to mitigate these risks. This involves optimizing sequence fidelity to ensure that CRISPR targets only intended genetic loci. Strategies such as high-fidelity Cas9 variants or engineered proteins like Cas12 have shown promise in improving specificity.
Moreover, incorporating computational models can assist in predicting off-target interactions, thus helping to tailor CRISPR’s precision. Advances in bioinformatics tools can help in designing and validating effective gRNAs with higher accuracy. More thorough validation and in vivo testing will also be essential to ensure that these advancements lead to safe and effective applications, especially in areas such as gene therapy and agriculture.
Expanding Applications Beyond Genomics
CRISPR technology’s potential extends well beyond genomic editing. One emerging area is its application in epigenetics. Researchers are beginning to explore how CRISPR can be utilized to regulate gene expression without altering DNA sequences. This field looks at modifying epigenetic marks, thereby influencing gene activity in more subtle ways, which might be crucial for treating complex diseases.
Another frontier is the use of CRISPR for synthetic biology applications. This includes creating new biological systems or pathways that can produce valuable compounds or novel therapeutics. For example, engineering microorganisms to manufacture pharmaceuticals or biofuels could revolutionize several industries.
Furthermore, CRISPR systems could be adapted for use in diagnostics and therapeutic interventions beyond gene editing, such as the development of CRISPR-based biosensors for disease detection. This versatility significantly changes the landscape of biotechnology, providing tools that are both powerful and adaptable.
The continued development of CRISPR technology not only provides significant advancements in genome editing but also opens doors to diverse applications that can reshape the future of medicine and biological research.
The continued development of CRISPR technology not only provides significant advancements in genome editing but also opens doors to diverse applications that can reshape the future of medicine and biological research.
Case Studies of Genome-Wide CRISPR Screens
Case studies serve as a pivotal element in understanding the practical applications of genome-wide CRISPR screens. They provide concrete examples that illustrate how this technology has been utilized in various scientific investigations. Through these examples, researchers can grasp the implementations, successes, and limitations faced in real-world scenarios.
These case studies also help to bridge the gap between theory and practice. They show not just the methodology but also how different research groups approach challenges, allowing for a deeper understanding of the innovation process in genetic research. Such insights are vital for students, researchers, and professionals looking to expand the scope of CRISPR technologies in their work.
Successful Implementations
Successful implementations of CRISPR screens have demonstrated the technology’s potential to revolutionize genetic research. One notable case involved the use of CRISPR screens to uncover new gene functions related to human disease. In this study, researchers employed a genome-wide approach to systematically knock out genes in human cell lines. This enabled them to determine the role of specific genes in disease pathways.
Here are key elements of successful implementations:
- Targeted Screening: The design of specific guide RNAs allowed for focused screening, reducing off-target effects and improving results accuracy.
- Validation: Subsequent validation of identified hits through secondary assays gave reliability to the findings.
- Interdisciplinary Collaboration: Collaboration between geneticists, bioinformaticians, and clinical researchers led to diverse perspectives that enhanced the study’s robustness.
Such effective strategies have led to significant discoveries, including new potential drug targets for various cancers and genetic disorders.
Comparative Studies
Comparative studies using genome-wide CRISPR screens play a crucial role in developing a nuanced understanding of its applications. By contrasting different CRISPR screening strategies, researchers can delineate the strengths and weaknesses inherent in each approach. For instance, comparisons between forward and reverse genetics screens provide valuable insights.
Here are common comparisons made in studies:
- Efficiency: Studies often highlight the efficiency of different libraries used in screening. Some libraries may lead to higher hit rates but might also contribute to increased noise in the data.
- Specificity: Comparative studies analyze how different gRNA designs impact specificity and off-target effects.
- Applicability: Researchers often evaluate which CRISPR approach is most appropriate for specific diseases or biological questions, guiding future research personnel in their experimental design.
"Understanding comparative results enhances the design of subsequent experiments, tailoring the CRISPR screen to specific research needs."
"Understanding comparative results enhances the design of subsequent experiments, tailoring the CRISPR screen to specific research needs."
Such analyses yield critical insights into the operational and theoretical frameworks of CRISPR technology, ultimately enriching the field of genetic research.
Ethical Considerations in CRISPR Research
The rapid advancement of genome editing technology, especially CRISPR, raises numerous ethical questions. These considerations are critical for researchers, policy makers, and the public alike. Ethical discussions can ensure that scientific progress aligns with societal values and norms. In the context of CRISPR, the implications of gene editing can be far-reaching, influencing not only individual health but also future generations.
Gene Editing Implications
Gene editing using CRISPR has vast potential to amend genetic disorders. However, it also brings challenges. For instance, the possibility of creating unintended genetic changes poses risks. These off-target effects can alter genes in ways that are currently unpredictable. This unpredictability could lead to adverse health outcomes, raising concerns about safety and consent.
Furthermore, germline editing—where modifications are passed to offspring—raises profound ethical issues. Decisions made today may shape the genetic profiles of future generations without their consent. The ethical dilemma here revolves around autonomy and the rights of individuals yet to be born.
A few key implications include:
- Safety Risks: The potential for unintended consequences in gene editing.
- Heritable Changes: Changes that persist through generations raise questions about the consequences of these modifications.
- Access and Inequality: There is a concern that advanced treatments may become accessible only to a wealthier segment of society, leading to ethical socio-economic divides.
The ability to edit the human genome calls for ethical scrutiny to minimize harm while maximizing benefits.
The ability to edit the human genome calls for ethical scrutiny to minimize harm while maximizing benefits.
Policy and Regulation
Policies governing CRISPR research are still in development across the globe. Regulatory frameworks must evolve to address the unique challenges posed by gene editing. Engaging with scientists, ethicists, and the public is essential for creating effective guidelines.
Key considerations for policy and regulation include:
- Research Oversight: Ensuring that all research involving CRISPR is closely monitored for safety and ethical compliance.
- Public Engagement: Educating the public about CRISPR's implications and encouraging dialogue. This can help in aligning research with societal expectations.
- International Standards: Considering cooperation across borders to establish a consistent approach to gene editing. Many countries may face divergent views on ethical issues surrounding CRISPR technology.
In summary, the ethical dimensions of CRISPR research must be given substantial attention. Addressing the implications of gene editing and establishing thoughtful policy can foster responsible innovation and public trust.