Comprehensive Guide to DNA Extraction Techniques and Protocols
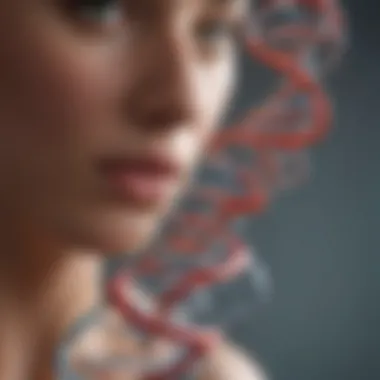
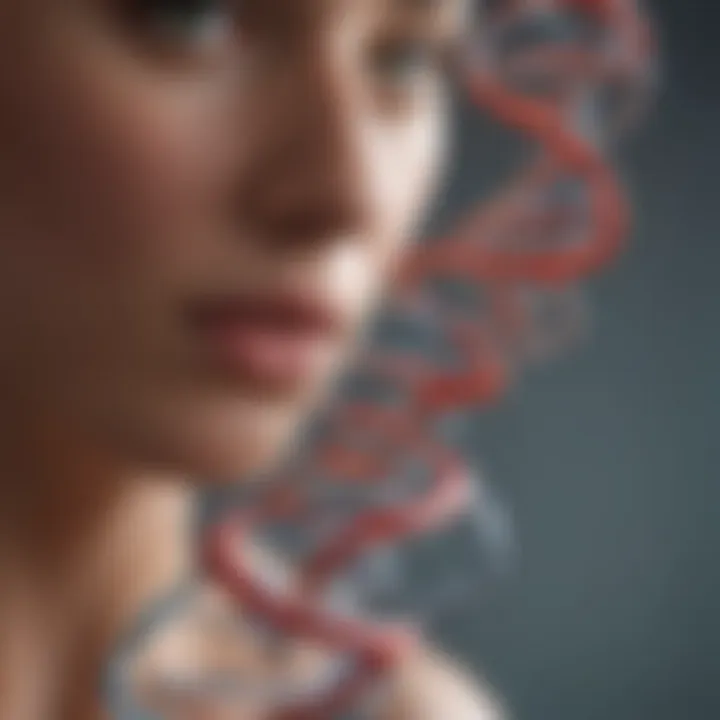
Article Overview
Purpose of the Article
This article aims to shed light on the nuances of DNA extraction, providing a thorough examination of various techniques and protocols. It seeks to bridge the gap between theoretical understanding and practical application, which is crucial for researchers working in diverse fields, from genetics to forensic science. By engaging with the underlying principles of DNA extraction, this guide intends to empower both seasoned scientists and eager learners with the insights necessary for successful execution.
Relevance to Multiple Disciplines
DNA extraction is not merely a technical procedure; it’s a fundamental skill that underpins multiple scientific disciplines. The ability to isolate DNA has far-reaching implications in areas such as:
- Biotechnology: Innovations in genetic engineering rely heavily on effective DNA extraction methods.
- Medicine: Understanding genetic disorders often hinges on the ability to analyze extracted DNA.
- Forensics: Criminal investigations utilize DNA extraction for identifying suspects.
- Evolutionary Biology: Studies of genetic variation can only begin with reliable extraction techniques.
Given this multifaceted relevance, the techniques discussed herein are critical for various applications, making this guide crucial for a broad audience including students, researchers, educators, and professionals engaged in science.
Research Background
Historical Context
The journey of DNA extraction started back in the mid-20th century, a time when scientists were plowing new fields in molecular biology. The first notable method was developed in 1950 by Friedrich Miescher, who isolated nuclein from white blood cells. Fast forward to the 1980s, when PCR (Polymerase Chain Reaction) became the game changer, allowing for the amplification of extracted DNA. This advancement led to a plethora of techniques, each tailored for specific types of materials, whether that be plant tissues, animal cells, or even environmental samples.
Key Concepts and Definitions
To appreciate the intricacies of DNA extraction, it’s essential to understand some foundational terms:
- Nucleic Acids: The molecules that carry genetic information, including both DNA and RNA.
- Lysis: The process of breaking down cell membranes to release DNA into solution.
- Precipitation: A step where DNA is separated from proteins and other cellular debris, usually using alcohol.
- Purification: The final stage where the DNA is isolated from any contaminants for further analysis.
Through clarity in these concepts, we can appreciate the methods we will explore in further sections—each trusty tool for extracting the genetic foundations of life.
Prolusion to DNA Extraction
DNA extraction serves as a cornerstone of molecular biology, playing a vital role in various scientific disciplines. This introductory section sets the stage to understand what DNA extraction entails—that is, the process of isolating this fundamental molecule from cells. DNA, or deoxyribonucleic acid, is the hereditary material in almost all living organisms; therefore, understanding its extraction is crucial for a plethora of applications including genetic engineering, diagnostics, and forensic science.
In a world where the study of genetics is becoming increasingly central, knowing the ins and outs of DNA extraction equips researchers and students alike with essential skills. Effective extraction techniques not only allow us to glean information about genetic makeup, but also contribute to advances in biotechnology, helping to tackle challenges in health, agriculture, and environmental science.
Fundamental Concepts
At its core, DNA extraction involves breaking down cellular structures to liberate the DNA. Understanding the basic principles behind this process is crucial for successful extraction. Three fundamental steps characterize most DNA extraction methods:
- Cell Lysis: This involves disrupting the cell membrane to release the DNA. Various agents like detergents or physical methods can be used to achieve this.
- Precipitation: Following lysis, alcohol such as ethanol or isopropanol is used to precipitate the DNA, effectively separating it from the other cellular components.
- Purification: The final step involves removing any remaining contaminants to ensure the DNA is suitable for downstream applications.
An understanding of these steps provides a solid foundation for further exploration of specific extraction methods.
Importance in Molecular Biology
The significance of DNA extraction cannot be overstated. It acts as the gateway to understanding genomic information and functions. Here are a few reasons why DNA extraction is crucial in molecular biology:
- Research Applications: In genetic research, extracting DNA from various organisms allows scientists to analyze genetic variations, study disease mechanisms, and conduct evolutionary studies.
- Clinical Diagnostics: The ability to extract DNA is indispensable in diagnosing conditions and determining the genetic basis of diseases, thus facilitating personalized medicine.
- Forensic Science: In the field of forensics, DNA extraction leads to profiling that aids in solving criminal cases and identifying victims based on biological evidence.
As molecular biology continues to evolve, mastering the art and science of DNA extraction remains pertinent, ensuring advancements that bridge various scientific fields.
Types of DNA Extraction Methods
Understanding the various types of DNA extraction methods is foundational for any work involving genetic material. Each method has unique nuances that cater to different applications, sample types, and desired purity levels. Furthermore, the choice of extraction technique can significantly affect the subsequent analysis, making this section essential for researchers and science enthusiasts alike. The effectiveness, efficiency, and overall success of DNA extraction depend heavily on the chosen method, hence understanding these types is invaluable.
Organic Extraction
Organic extraction, known for its time-tested approach, primarily utilizes organic solvents. This method remains a mainstay due to its ability to yield high-quality DNA, making it a favored choice in many laboratories.
Chemical Agents
Chemical agents like phenol chloroform have a proven track record in facilitating organic extraction. One notable characteristic of chemical agents is their ability to efficiently separate DNA from proteins and other contaminants. This property makes them quite popular in the context of this guide. However, it is crucial to handle these chemicals with care due to their toxicity and potential health risks. The benefit of using chemical agents lies in their efficiency, allowing researchers to achieve high purity levels, although they require meticulous handling and waste disposal.
Application in Genomics
In terms of genomics, the application of organic extraction techniques hasn’t waned. This method provides a robust solution for isolating high-quality genomic DNA from various biological samples such as tissues and blood. Its ability to reliably yield DNA suitable for downstream applications like PCR or sequencing is a leading characteristic. Some may view this method as cumbersome compared to others, but the reliability and quality it offers often outweighs the inconvenience, making it a fundamental technique in genomics.
Silica-Based Methods
Silica-based methods have surged in popularity due to their simplicity and effectiveness, utilizing silica membranes to bind DNA during the extraction process.
Mechanism of Action
The mechanism of action involves the binding of DNA to silica in the presence of high concentrations of chaotropic salts. This allows for the selective precipitation of DNA while other substances can be washed away. The straightforward nature of this method makes it a beneficial choice for many in laboratories. The unique feature here is its rapidity; it generally provides quick results without the need for toxic chemicals. However, for novice users, inconsistency in salt concentrations could lead to varying yields, which can be a drawback if precision is paramount.
Efficiency and Purity
When considering efficiency and purity, silica-based methods are often commended for their high repeatability in providing clean DNA extracts. This makes them particularly attractive for high-throughput settings such as clinical diagnostics or research environments. However, while they generally lead to high-purity DNA, some specific sample types may not yield the expected results, thereby necessitating careful consideration.
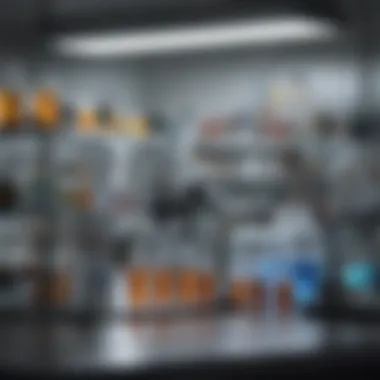
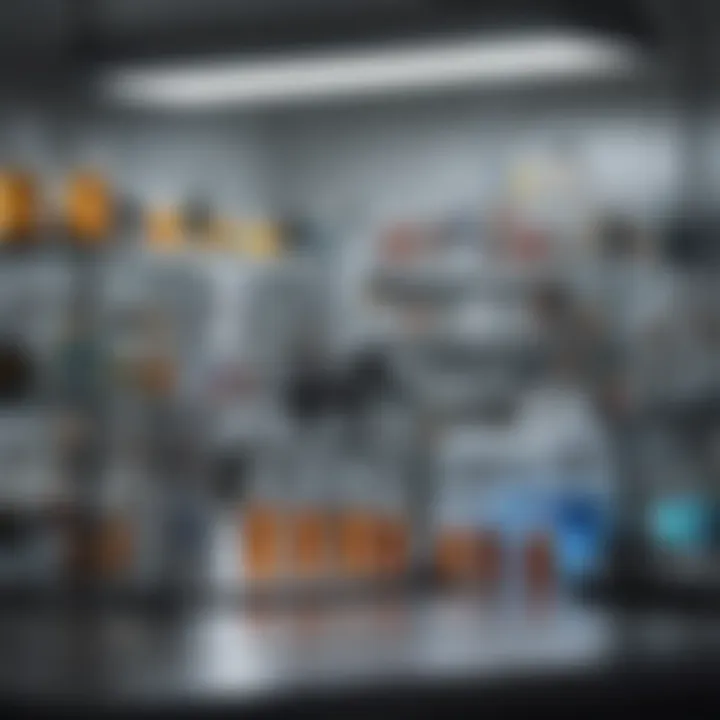
Magnetic Bead Extraction
Magnetic bead extraction has carving out a niche in modern laboratories, characterized by its ease and versatility in handling various sample types.
Principles of Magnetism
The principles of magnetism come into play through the use of beads coated with DNA-binding agents. This enables the DNA to be isolated from its environment simply by applying a magnetic field. One core characteristic of this method is its adaptability; it can be applied across various biological specimens — from cells to even more challenging sources. The primary advantage is that it minimizes cross-contamination risks due to the closed nature of the process, although it can be somewhat more expensive than traditional methods.
Ease of Use
The ease of use factor cannot be understated when it comes to magnetic bead extraction. It allows for rapid processing, often requiring minimal training for personnel. This is particularly crucial in high-volume labs where efficiency is key. However, some may find that the initial investment in equipment could be a hurdle in adopting this method compared to others that are more straightforward and cheaper.
Automated DNA Extraction
With the ever-increasing need for efficiency, automated DNA extraction methods have gained traction, embodying the future of DNA extraction technologies.
Advantages of Automation
The advantages of automation are manifold; it allows for consistency across large numbers of samples, reducing human error. Automation also brings speed to the table, which is a game changer for labs with high sample throughput. Though the upfront costs can be high when investing in these automated systems, the benefit comes in the form of time saved and standardized results that can elevate the quality of research outputs.
Sample Throughput
In terms of sample throughput, automated systems excel, particularly in environments where a large volume of samples needs to be processed quickly and reliably. By minimizing manual intervention, these systems enhance the overall efficiency of the laboratory workflow. On the flip side, the reliance on machines may introduce some complications if malfunctions occur; maintenance becomes crucial to avoid dipping into the productivity of the laboratory.
Detailed Protocols for DNA Extraction
In the realm of genetic research, having well-defined protocols for DNA extraction is crucial. These protocols serve as a roadmap, guiding researchers through the often complex landscape of isolating DNA from various sources. A well-executed extraction can ensure high yields and purity of the DNA, which can directly influence downstream applications in genetic analysis, cloning, and sequencing.
Despite the similarities across various extraction methods, the specifics of each protocol can have significant impacts on overall outcomes. This necessitates a comprehensive understanding of the steps involved, as well as considerations unique to each method.
Sample Collection and Preparation
Before diving into the technicalities, the foundation of any successful DNA extraction begins with sample collection. It’s not just about grabbing whatever is on hand; the choice of sample can dictate the quality and integrity of the extracted DNA. Depending on the source, whether it's human, plant, or bacterial cells, different collection methods might be necessary. For instance, human samples might come from blood or saliva, while plant DNA might involve leaf or root tissues.
Moreover, the way samples are stored impacts their viability for extraction. Using sterile tools and avoiding contamination is vital. Any foreign DNA can compromise the results, leading to misleading conclusions. After collection, samples often require processing—homogenization or grinding—especially in cases of tough or fibrous materials. This pre-extraction step is key in ensuring that the cells are adequately prepared for lysis, allowing for maximum yield.
Step-by-Step Protocols
Cell Lysis
Cell lysis is the first significant step in DNA extraction, where the cell membranes are disrupted to release the DNA. This process is essential, as it sets the stage for the subsequent steps. The critical characteristic of cell lysis is its ability to effectively break open various cell types, including both delicate and robust cells.
What makes cell lysis a popular choice in protocols is the variety of lysis buffers available. Depending on what kind of cells you are working with, one might opt for a detergent-based buffer for animal cells or a more robust mechanical method for plant cells. Each method comes with its advantages, such as speed and efficiency, yet carries its downsides; for example, excessive agitation may shear the DNA.
Precipitation of DNA
Once cells are lysed, the next step involves the precipitation of DNA from the cellular debris. This is often accomplished using alcohol—ethanol or isopropanol—mixed with salt. The key aspect of this step is that DNA is selectively soluble in alcohol, while proteins and other impurities precipitate out.
The beauty of this process lies in its simplicity and efficiency. By carefully controlling the ratios of alcohol to solution, researchers can achieve a high yield of DNA. However, this step is not without its quirks; if the temperature is not cold enough, the DNA may not precipitate adequately, resulting in lower yields. The precipitation of DNA can easily be viewed as a rite of passage in the extraction process, where the once invisible genetic material becomes tangible.
Washing and Resuspension
After precipitation, washing the DNA with cold alcohol is crucial to removing residual impurities. This process of washing helps increase the purity of the extracted DNA, which is beneficial for downstream applications. The characteristic aspect of washing is ensuring that any leftover salts are thoroughly removed. Without this step, residual contaminants can lead to issues with subsequent experiments, such as PCR misfires or sequencing errors.
The resuspension of DNA in a suitable buffer completes the extraction process. Choosing the right resuspension buffer can influence the stability of the DNA and, consequently, its usability in further applications. It’s worth noting that the delay in resuspension can result in DNA degradation, so timing is of the essence.
Quality Control of Extracted DNA
With the DNA extracted, the next segment involves ensuring its quality. Quality control is vital, as the integrity and concentration of the DNA can significantly affect experimental outcomes.
Spectrophotometric Analysis
One of the primary methods for assessing DNA quality is via spectrophotometric analysis. This technique relies on measuring absorbance at specific wavelengths. The common characteristic of spectrophotometric analysis is its ability to provide a quick and relatively easy way to assess purity by comparing ratios at 260 nm and 280 nm. A ratio of around 1.8 is generally accepted as a marker of high purity.
Advantages of this method are plentiful—it's fast, cost-effective, and requires minimal sample preparation. However, it does have a drawback, as the presence of contaminants can sometimes lead to misleading results, not to forget its inability to quantify the integrity of the DNA, only its purity.
Agarose Gel Electrophoresis
Agarose gel electrophoresis follows spectrophotometric analysis as another method for quality control, offering a visual representation of DNA integrity. This method involves applying an electric current to a gel containing the DNA, allowing it to migrate based on size.
The uniqueness of agarose gel electrophoresis lies in its capacity to visualize DNA, separating fragments and elucidating the overall quality. It is especially useful for checking whether extracted DNA has been sheared or degraded during the process. Its advantage lies in the ability to provide detailed insights into the sample health. Adding a disadvantage, though, it requires more time and handling than spectrophotometric analysis.
Ultimately, both spectrophotometric analysis and agarose gel electrophoresis can complement each other in granting a complete picture of the extracted DNA's quality.
Ultimately, both spectrophotometric analysis and agarose gel electrophoresis can complement each other in granting a complete picture of the extracted DNA's quality.
Considerations and Best Practices
In the intricate world of DNA extraction, understanding the nuances of best practices is essential for achieving accurate and reliable results. This section delves into various considerations that scientists and researchers should keep in mind when conducting DNA extraction, emphasizing how these elements contribute to successful experimental outcomes.
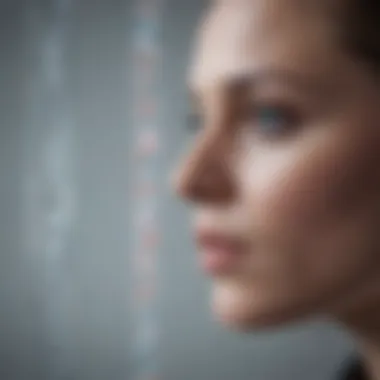
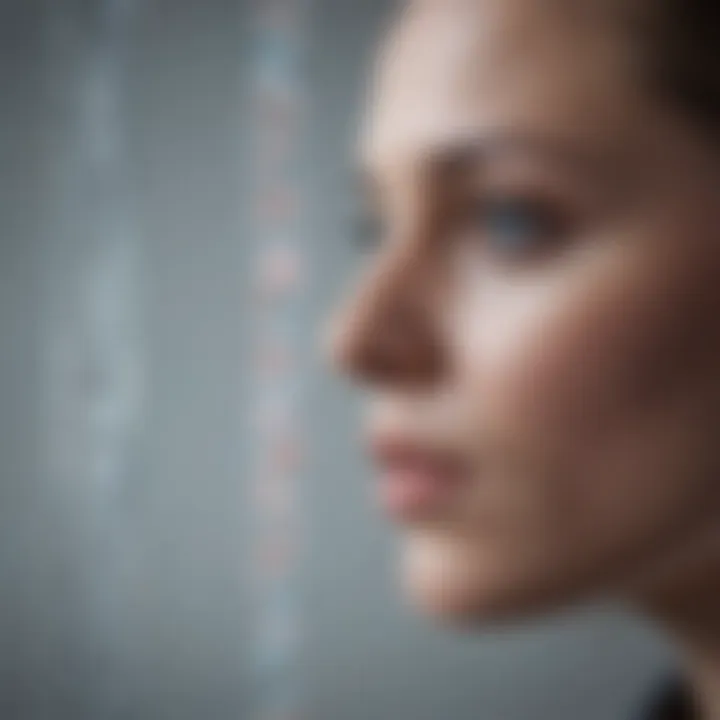
Common Contamination Issues
Contamination is a persistent challenge in DNA extraction, and it can severely compromise the integrity of the extracted genetic material. Common contamination issues can arise from various sources, such as laboratory equipment, reagents, or even environmental factors. For instance, improper handling of samples can introduce unwanted DNA, leading to skewed results or false positives.
To mitigate these risks, consider the following strategies:
- Use of sterile technique: Always work in a clean environment. Wear gloves, and use disposable pipette tips to avoid transferring contaminants.
- Regular cleaning: Ensure that DNA extraction surfaces and tools are regularly decontaminated with appropriate solutions like bleach or ethanol.
- Sample storage: Store samples at the correct temperatures promptly after collection to prevent degradation or contamination.
By maintaining rigorous standards throughout the extraction process, researchers can significantly reduce the likelihood of contamination, ensuring that their findings remain valid and reliable.
Troubleshooting Extraction Challenges
Issues during DNA extraction are not uncommon, and knowing how to troubleshoot effectively can save time and resources. Let's look at two prevalent challenges faced by researchers: low yield and degraded samples.
Low Yield
Low yield refers to the situation where the amount of DNA extracted is considerably less than anticipated. This aspect is particularly disheartening, especially when researchers have invested time and effort into sample preparation. The common causes of low yield can include poor cell lysis, inefficient precipitation, or the degradation of samples prior to extraction.
Understanding the key characteristic of low yield is crucial. It often indicates flaws in the extraction protocol or issues related to sample quality itself. Moreover, it can influence downstream applications, leading to inconclusive results or requiring repeat experiments.
To address low yield, researchers can:
- Reassess the cell lysis method. Choosing a more efficient lysis buffer can enhance the extraction process.
- Optimize precipitation conditions. Adjusting the concentrations of alcohol and salt can sometimes yield better results.
- Ensure samples are fresh and properly stored before extraction.
While low yield is a drawback, its identification can direct researchers to optimize protocols, potentially improving future outcomes.
Degraded Samples
Degraded samples pose another significant problem in DNA extraction. When DNA is damaged or fragmented, it can hinder analyses, leading to inaccurate results. The integrity of collected samples can be affected during collection, transport, or storage. Understanding how to handle degraded samples is vital.
The primary issue with degraded samples is that they can yield lower-quality DNA, complicating applications such as PCR amplification or sequencing. However, researchers can adopt certain practices:
- Prompt processing: Extract DNA from samples as soon as possible to minimize degradation risks.
- Utilize stabilizing agents: Incorporating solutions that protect DNA, such as lysis buffers designed for preservation, can aid in maintaining sample integrity.
- Assess quality before use: Using spectrophotometry or gel electrophoresis can help identify the quality of the extracted DNA.
Addressing the problem of degraded samples can ultimately allow researchers to salvage valuable findings, emphasizing the importance of diligence throughout the extraction process.
"Effective troubleshooting and adherence to best practices can significantly elevate the reliability of DNA extraction outcomes."
"Effective troubleshooting and adherence to best practices can significantly elevate the reliability of DNA extraction outcomes."
By paying close attention to contamination risks and honing skills in troubleshooting challenges such as low yield and degraded samples, researchers can enhance the reliability and validity of their DNA extraction efforts.
Safety and Ethical Considerations
In DNA extraction, the safety and ethical dimensions hold paramount significance, framing the very foundation of how this complex process is approached and managed. Ensuring that all practices adhere to stringent safety measures not only protects researchers but also preserves the integrity of the samples being handled. Furthermore, ethical considerations entwined with genetic research emphasize transparency, integrity, and responsibility towards both human and non-human subjects. This balance between safety protocols and ethical practices is crucial for maintaining public trust and advancing scientific exploration.
Handling Biological Materials
When dealing with biological materials, one must tread carefully. The risk associated with exposure to infectious agents, especially when extracting DNA from clinical or environmental samples, cannot be underestimated. Safety gear such as gloves, lab coats, and goggles is non-negotiable. Proper training in biohazard protocols is essential, as even a single misstep could lead to contamination or, worse, exposure to harmful pathogens.
Implementing standard operating procedures (SOPs) is key to ensuring that all personnel are aware of potential hazards and how to mitigate them. Here are some best practices for handling biological materials:
- Use specified containment procedures to prevent accidental exposure.
- Decontaminate work surfaces and equipment before and after experiments.
- Dispose of waste properly to avoid any environmental impact.
By following these guidelines, not only is safety ensured, but the quality of the DNA extraction is also maintained, leading to more reliable research outcomes.
Ethics in Genetic Research
Delving into the realm of ethics in genetic research raises crucial questions about human rights, societal implications, and the boundaries of scientific inquiry. Researchers must navigate a landscape laden with ethical dilemmas, particularly concerning the use of genetic materials from living or deceased individuals.
Informed Consent
Informed consent serves as the bedrock of ethical research practices. It requires that individuals provide clear agreement to participate after being fully briefed about the research's purpose, procedures, risks, and benefits. This transparency fosters trust and empowers subjects, allowing them to make educated choices about their genetic information. Key characteristics of informed consent include:
- Clarity: Participants should understand what they're consenting to without any confusion.
- Voluntariness: Consent must be given freely, without undue pressure.
- Right to withdraw: Subjects should have the ability to withdraw their consent at any time without repercussions.
A unique feature of informed consent is the safeguarding it offers against exploitation. Collecting DNA samples without appropriate consent not only violates ethical standards but can lead to legal ramifications for researchers. While the benefit of informed consent is clear—protecting individual rights and promoting ethical research—it can also be time-consuming in complex studies where participant engagement might be harder to achieve.
Data Privacy
Data privacy is another significant pillar in the ethical landscape of genetic research. As researchers work with genetic information, they must ensure that personal data remains confidential and secure. The DNA extracted from samples can reveal sensitive information, making it imperative to have strong data protection measures in place.
Key characteristics of data privacy include:
- Anonymization: Personal identifiers must be removed to protect participants' identities.
- Limited access: Only authorized personnel should be able to access genetic data.
The unique feature of data privacy is its dual role as a protective barrier for participants and a regulatory requirement for researchers. While stringent data privacy measures maintain public confidence, they can limit the scope of research if data can't be shared among collaborators. Balancing ethical considerations with research needs is an ongoing challenge in this dynamic field.
In genetic research, safety and ethical considerations are not just compliance measures, but vital components that shape scientific integrity and community trust.
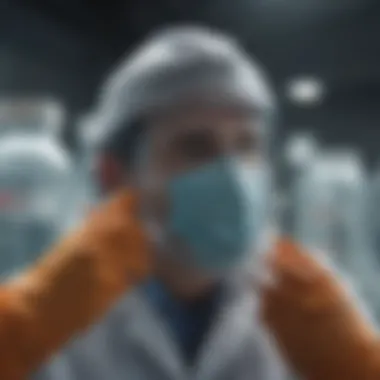
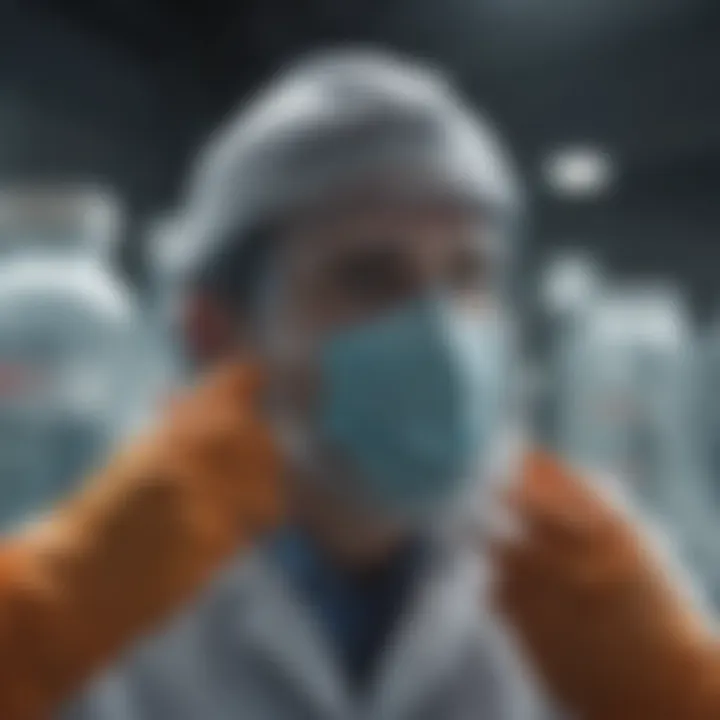
In genetic research, safety and ethical considerations are not just compliance measures, but vital components that shape scientific integrity and community trust.
Applications of DNA Extraction
Understanding the applications of DNA extraction is crucial, as it bridges the gap between theoretical knowledge and practical application. Extracted DNA serves as a pivotal tool across various fields, advancing research and driving innovations that can significantly impact society.
Genetic Research and Biotechnology
In genetic research, DNA extraction is the bedrock upon which studies are constructed. For instance, researchers employ extracted DNA to study genetic variations linked to diseases, paving the way for potential therapies and interventions. By isolating specific regions of DNA, scientists can compare sequences among different populations or species, revealing insights into evolution, genetic drift, and adaptation. Biotechnology further benefits from these extractions, as they enable the creation of genetically modified organisms (GMOs) designed for improved crop yields or disease resistance. The ability to manipulate genetic material expands the horizons for agricultural practices and food security.
Clinical Diagnostics
The role of DNA extraction in clinical diagnostics cannot be overstated. Healthcare professionals utilize this technique to diagnose genetic disorders, infections, and cancer. For example, extracted DNA allows for the identification of pathogen sequences, helping clinicians develop targeted treatment approaches. The precision of genetic testing enhances patient care by providing personalized medicine options, guiding treatment plans that cater to individual genetic profiles. Moreover, the rise of non-invasive prenatal testing, where fetal DNA is extracted from maternal blood, exemplifies how this application can lead to early diagnosis and informed decision-making.
Forensic Science
Forensic science represents a fascinating domain where DNA extraction plays a critical role in criminal justice. Investigators use extracted DNA to solve crimes, identify victims, and exonerate the innocent. This application often involves two prominent aspects:
DNA Profiling
DNA profiling, also known as genetic fingerprinting, has emerged as a cornerstone in forensic investigations. What sets DNA profiling apart is its ability to generate unique genetic profiles from tiny biological samples, such as hair or blood. This high specificity makes it an incredibly powerful technique for linking suspects to crime scenes or identifying unknown individuals. However, while DNA profiling is immensely eficaz, it also comes with ethical considerations regarding data privacy and potential misuse.
Case Studies
Case studies in forensic science provide real-world examples of how DNA extraction and profiling can lead to justice. For instance, the use of DNA evidence in high-profile cases has not only solved long-standing mysteries but has also changed judicial outcomes. A key characteristic of these case studies is that they highlight the transitional journey from investigation to conviction. While they serve to emphasize the benefits of DNA evidence, they also illustrate the limitations, such as contamination risks or reliance on the quality of the extracted sample.
"Forensic DNA analysis stands as a beacon of hope in the fight for justice, encapsulating both the promise of scientific advancement and the necessity for ethical oversight."
"Forensic DNA analysis stands as a beacon of hope in the fight for justice, encapsulating both the promise of scientific advancement and the necessity for ethical oversight."
As DNA extraction continues to evolve, its applications span an ever-expanding horizon—from genetic research to clinical applications and forensic investigations, underpinning the essence of its importance in modern science.
Future Directions in DNA Extraction Techniques
The landscape of DNA extraction is continually evolving, driven by advances in technology, a deeper understanding of genetic material, and the ever-growing needs of various fields. Understanding these directions not only aids researchers in obtaining better results but also encourages innovative applications across disciplines. The importance of focusing on future directions cannot be overstated; as DNA methodologies improve, they promise increased efficiency, accuracy, and applicability in diverse laboratory environments.
Innovations in Extraction Methods
As we inch further into the 21st century, novel extraction methods are emerging that challenge the traditional paradigms. Look at enzymatic extraction techniques for instance; these methods leverage specific enzymes to break down cellular components without the need for harsh chemicals. This approach has garnered attention, particularly in human sample manipulations, where purity is paramount. By adopting this kind of tactic, labs can not only improve the quality of DNA obtained but also minimize potential contaminants that can interfere with downstream applications.
Another innovation worth mentioning is the introduction of smartphone-based extraction kits. These portable kits are engineered to facilitate on-site sample processing in remote areas, where access to sophisticated lab facilities is limited. Imagine extracting DNA from wildlife specimens or agricultural samples right in the field with a device in your pocket. The implications for biodiversity studies, epidemiology, and forensic science are enormous.
- Benefits of Innovations:
- Enhanced efficiency in sample processing
- Increased purity of extracted DNA
- Accessibility for field settings
Despite these advancements, it's vital to consider the challenges that come with them, such as reliability in various environmental conditions and standardization across different sample types. Researchers must weigh these factors against the potential for improved methodologies.
Interdisciplinary Approaches
The future of DNA extraction is not just in refining existing methods, but also in the collaboration between multiple fields. Take the synergy between bioinformatics and molecular biology; data analytics can significantly enhance the extraction process by optimizing protocols based on real-time analysis of DNA quality. For instance, machine learning algorithms may assist in predicting which extraction techniques will yield the best results based on specific sample characteristics.
This trend is not confined to the lab. Public health initiatives are also leveraging cross-disciplinary frameworks. In disease outbreak investigations, DNA extraction from various biological samples can reveal insights about pathogen evolution. Here, microbiology and epidemiology intersect, driving new approaches for monitoring and managing public health.
Additionally, environmental sciences are taking giant leaps. Techniques like DNA barcoding are being integrated into biodiversity assessments. This method allows researchers to identify species from environmental samples using extracted DNA, providing crucial information about ecosystem health.
"Interdisciplinary collaboration is not just advantageous; it is essential for paving the way for future discoveries in DNA extraction and application."
"Interdisciplinary collaboration is not just advantageous; it is essential for paving the way for future discoveries in DNA extraction and application."
The benefits of interdisciplinary approaches extend beyond mere advances in methodology; they foster innovation, opening doors to uncharted territories, which could redefine how we understand and utilize genetic material.
In summary, the future directions in DNA extraction techniques herald a transformative period marked by innovative extraction methods and interdisciplinary collaboration. Engaging with new technologies while fostering partnerships across fields will ensure a thriving landscape that adapts to emerging scientific challenges and societal needs.
Epilogue and Key Takeaways
In wrapping up this deep dive into the realm of DNA extraction, it becomes clear how crucial this field is in molecular biology and its various applications. The techniques covered not only reflect the evolution of scientific practices but also its necessity in genetic research, diagnostics, and forensic science. Understanding these methods isn’t just about knowing how to extract DNA; it's about grasping the very essence of genetic material and its implications.
Summary of Techniques
A brief revisit to the techniques discussed throughout the article reveals their individual merits:
- Organic Extraction: Relies heavily on solvents to break down cellular components, allowing for effective DNA retrieval. Its applications span from academia to industry, particularly in genomic studies.
- Silica-Based Methods: A favorite for many labs due to their efficiency and high purity yields. The technique utilizes silica to selectively bind DNA, which can significantly streamline the extraction process, making it a mainstay in many labs.
- Magnetic Bead Extraction: A modern approach focusing on ease and speed, magnetic bead methods leverage magnetism for a quick DNA isolation, fitting for high-throughput scenarios.
- Automated DNA Extraction: As labs grow, automation is key. These systems boost sample throughput and reduce human errors, a critical factor in precise science.
Each of these methods offers distinct advantages, catering to different needs and contexts in the scientific landscape.
Implications for Future Research
Looking ahead, the implications of advanced DNA extraction techniques cannot be overstated. The emerging technologies promise not only to enhance the efficiency of current methods but also to radically shift how we approach genetic research. For instance, the integration of nanotechnology into extraction processes presents exciting opportunities for improved specificity and yield.
Additionally, interdisciplinary approaches, incorporating elements from fields such as bioinformatics and material sciences, could yield new extraction strategies. Such strategies could further propel research into personalized medicine, a burgeoning field that holds the potential to tailor medical treatments based on individual genetic profiles.
"The future is not something we enter. The future is something we create." - Leonard I. Sweet
"The future is not something we enter. The future is something we create." - Leonard I. Sweet