Hydrogen from Biomass: Technologies and Benefits
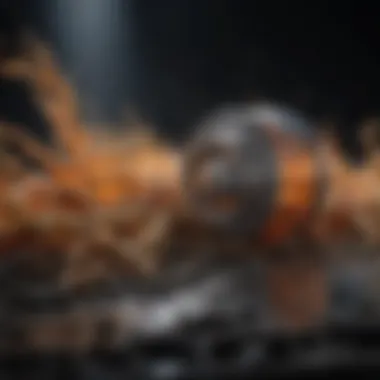

Article Overview
The production of hydrogen from biomass represents a significant advancement in the pursuit of sustainable energy solutions. This article aims to dissect the mechanisms, technologies, and applications related to biomass hydrogen production. By examining these facets, we aim to illustrate not only the technical processes involved but also the broader implications for energy systems.
Purpose of the Article
The primary purpose of this article is to provide an insightful exploration into the complex processes and technologies associated with hydrogen production from biomass. It seeks to inform stakeholders in various fields about the potential of this technology as a viable source of renewable energy, emphasizing environmental sustainability and economic viability. In doing so, the article will highlight current research and case studies that demonstrate real-world applications and outcomes.
Relevance to Multiple Disciplines
Hydrogen production from biomass is pertinent to several fields, including environmental science, chemistry, engineering, and energy policy. As global energy needs grow, professionals in these areas must understand how biomass can contribute to energy systems and mitigate climate change impacts. By integrating knowledge from these disciplines, we can better appreciate the nuances of biomass-to-hydrogen conversions and their implications for future developments in renewable energy.
Research Background
Historical Context
The concept of converting biomass to energy is not new; it has its roots in ancient practices of burning organic materials for heat and light. However, the modern scientific approach to biomass conversion has evolved significantly, especially in the last few decades. As concerns about fossil fuel dependency and greenhouse gas emissions grew, research intensified in developing efficient methods for biomass conversion to hydrogen. Recent advancements in technology have further accelerated this process, making it a focus area for researchers worldwide.
Key Concepts and Definitions
To better understand the processes involved in hydrogen production from biomass, it is essential to clarify some key concepts:
- Biomass: Organic material derived from plants and animals, used as a renewable energy source.
- Biochemical Conversion: Processes that use biological methods, such as fermentation, to convert biomass into hydrogen.
- Thermochemical Conversion: Heat-driven processes, including gasification and pyrolysis, that break down biomass into hydrogen-rich gases.
- Hydrogen Production: The generation of hydrogen gas, which can be utilized as a clean energy carrier.
These definitions set the stage for a deeper exploration of the mechanisms and technologies that follow. It is within these frameworks that the challenges and benefits of biomass hydrogen production will be assessed.
Foreword to Biomass and Hydrogen Production
The intersection of biomass and hydrogen production presents a promising avenue toward achieving sustainable energy goals. Biomass serves as a renewable feedstock, offering potential to mitigate carbon emissions while providing clean energy sources. Understanding this relationship is crucial, as it illuminates pathways for cleaner fuel alternatives in diverse applications such as transportation, industry, and power generation.
Defining Biomass as a Feedstock
Biomass comprises organic materials such as plant matter, agricultural waste, and residues from forestry. These sources can be converted into hydrogen through various processes that harness their energy content.
One significant property of biomass is its ability to sequester carbon during its growth phase, making it a carbon-neutral option at the time of combustion or processing. Notably, lignocellulosic biomass, which includes materials like straw, wood chips, and sugarcane bagasse, represents a rich arena for hydrogen production. The challenge lies in the complex structural composition of these materials, demanding advanced technologies for efficient conversion into hydrogen.
This feedstock is not only abundant in nature but also diverse in availability. The suitability for hydrogen extraction often depends on regional agricultural practices and waste management systems. Integrating biomass into hydrogen production could lead to reduced reliance on fossil fuels, providing a circular economy where waste is repurposed effectively.
The Role of Hydrogen in Sustainable Energy
Hydrogen is frequently heralded as a clean energy carrier. Its role becomes increasingly vital as the world faces the pressing issues of climate change and energy security. The clean combustion of hydrogen results in water vapor as the only byproduct, a stark contrast to the greenhouse gas emissions from traditional fossil fuels.
Moreover, hydrogen can serve multiple purposes across sectors. For example, it can be utilized in fuel cells for vehicles, in industrial processes like ammonia production, and as a potential energy storage medium. A robust hydrogen economy hinges on widespread production and distribution capabilities, and biomass-derived hydrogen presents a realistic solution to supply these demands.
The use of hydrogen from biomass not only enhances energy systems but also promotes enhanced sustainability. It aligns with global strategies aimed at transitioning to cleaner solutions, particularly as renewable energy sources expand. Thus, the discussions surrounding hydrogen production from biomass must encompass both its environmental benefits and its applicative versatility in future energy frameworks.
Biomass Types and Their Suitability for Hydrogen Production
Hydrogen production from biomass relies heavily on the type of biomass used. Each type has unique characteristics that determine its efficiency and suitability for conversion to hydrogen. The relevance of this section lies in understanding how different biomass types impact yield, process conditions, and overall feasibility for sustainable hydrogen production. By analyzing lignocellulosic biomass, algal biomass, and waste biomass, we can identify their benefits and challenges in hydrogen generation.
Lignocellulosic Biomass
Lignocellulosic biomass includes materials such as wood, straw, and agricultural residues. It is abundantly available and represents a significant resource for hydrogen production. This type of biomass is made predominantly of cellulose, hemicellulose, and lignin. Each component plays a role in how biomass can be processed into hydrogen.
- Cellulose is a carbohydrate that can be converted into glucose through hydrolysis. It is a primary sugar that can be further fermented into hydrogen.
- Hemicellulose provides a lower energy yield but is more readily hydrolysable.
- Lignin, while more challenging to break down, can contribute to the energy content and enhance overall biomass properties.
The challenge with lignocellulosic biomass is its recalcitrant nature. Efficient methods, such as pretreatment, are necessary to enhance accessibility for enzymatic hydrolysis. These pretreatments can involve physical, chemical, or biological approaches to improve the yield of fermentable sugars.
Algal Biomass
Algal biomass stands out due to its rapid growth rates and high lipid content. Algae can be cultivated in a variety of environments, including saltwater, freshwater, and even wastewater. The two main categories of algae considered for hydrogen production are microalgae and macroalgae.
- Microalgae often produce significant amounts of oil and carbohydrates that can be converted into hydrogen. Some species can generate hydrogen through photofermentation.
- Macroalgae or seaweeds, on the other hand, are predominantly composed of polysaccharides like agar and alginate. They can be processed to extract sugars for fermentation or can undergo anaerobic digestion to generate biogas, which contains hydrogen.
The main benefit of algal biomass is its ability to absorb CO2 and produce oxygen, positively impacting the environment. However, large-scale cultivation and harvesting present logistical challenges that need addressed.
Waste Biomass
Waste biomass refers to organic waste materials from industrial, agricultural, and municipal sources. Examples include food waste, animal manure, and plant residues from processing.
The advantages of using waste biomass for hydrogen production include:
- Waste reduction: Valorization of waste materials reduces landfill contributions and releases of greenhouse gases.
- Cost-effectiveness: Waste is often available at low or no cost, making it an economically attractive option for hydrogen production.
However, the heterogeneous nature of waste biomass poses challenges. Variability in composition can affect process efficiency. Moreover, the presence of contaminants may necessitate preprocessing. This includes sorting and separating undesirable materials to ensure high-quality feedstock.
The successful integration of diverse biomass types in hydrogen production will require innovative processes and technologies tailored to specific feedstock properties.
The successful integration of diverse biomass types in hydrogen production will require innovative processes and technologies tailored to specific feedstock properties.
In summary, the distinct characteristics of lignocellulosic, algal, and waste biomass dictate their suitability for hydrogen production. Understanding these differences is crucial in optimizing processes and improving yields.
Biochemical Methods for Hydrogen Production
Biochemical methods for hydrogen production represent a cornerstone in the field of renewable energy. These processes leverage biological systems to convert biomass into hydrogen gas. This approach is pivotal, as it not only aids in producing renewable energy but also aligns with global sustainability goals. The use of microorganisms in these methods opens doors to diverse biomass feeds that can yield hydrogen efficiently.
The benefits associated with biochemical methods include lower energy inputs compared to thermochemical processes, and the potential to utilize various types of biomass, which makes it a versatile choice. These factors make biochemical methods a significant area of focus in the exploration of sustainable hydrogen production.
Fermentation Process
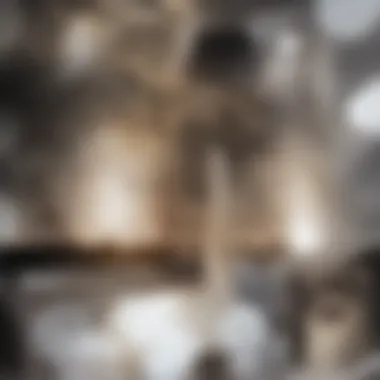

Fermentation is one of the primary biochemical processes used to generate hydrogen. It involves the breakdown of organic matter by microorganisms in anaerobic environments. During this process, organic compounds are converted into hydrogen, carbon dioxide, and other metabolites.
The fermentation process can occur through several pathways, depending on the types of feedstock and microorganisms used. For instance, hydrogen-producing bacteria like Clostridium can efficiently convert glucose or other sugars into hydrogen. In addition to being effective, this method can harness waste biomass such as agricultural residues, effectively addressing waste management issues while producing energy.
In terms of optimizing yield, several factors come into play.
- pH Levels: Maintaining an optimal pH is crucial for microbial activity.
- Temperature: Temperature control is necessary for maximizing hydrogen production.
- Nutrient Availability: The presence of nutrients can significantly influence microbial growth and hydrogen yield.
Dark Fermentation vs. Photofermentation
When discussing fermentation for hydrogen production, two notable processes emerge: dark fermentation and photofermentation.
- Dark Fermentation: This process occurs in the absence of light, with microorganisms degrading organic substrates to produce hydrogen. It is characterized by its efficiency in utilizing a variety of feedstocks. Since it does not require light, this method can be implemented in a range of conditions, making it widely applicable.
- Photofermentation: In contrast, photofermentation uses light energy in addition to organic substrates. Photosynthetic bacteria perform this process, converting light into chemical energy to produce hydrogen. This method often provides higher yields due to the dual role of light and substrates in the hydrogen production process.
Both types of fermentation have distinct advantages and challenges. Dark fermentation is typically simpler to run in various settings. However, photofermentation offers the possibility of higher hydrogen yields, although it is more dependent on environmental light conditions.
Using both methods in a complementary manner could optimize hydrogen production and utilization of available resources.
Using both methods in a complementary manner could optimize hydrogen production and utilization of available resources.
Ultimately, understanding these biochemical methods and their nuances can drive advancements in sustainable hydrogen production, contribute to a circular economy, and mitigate environmental impact.
Thermochemical Processes in Hydrogen Generation
Thermochemical processes represent a pivotal method in generating hydrogen from biomass. These processes leverage heat and chemical reactions to convert organic materials into usable hydrogen fuel. The significance of thermochemical methods lies in their ability to handle a wide variety of biomass types, including solid and liquid feedstocks, thus enhancing the flexibility of hydrogen production.
The thermochemical processes discussed here mainly include gasification and pyrolysis, each with unique characteristics and advantages. Understanding these methods is essential for optimizing hydrogen yields and improving the overall efficiency of production systems. Additionally, these processes can help integrate biomass into existing energy infrastructures effectively, making them a crucial focus in the shift towards renewable energy sources.
Gasification
Gasification is a crucial thermochemical process that converts biomass into syngas, primarily composed of hydrogen and carbon monoxide. The process typically occurs under high temperatures (around 700 to 1,000 degrees Celsius) in an oxygen-limited environment. This careful management of the oxidation state is vital as it influences the composition of the resultant gas.
The advantages of gasification are numerous:
- High Hydrogen Yield: Gasification generally results in a higher yield of hydrogen compared to other processes.
- Versatile Feedstock: It can utilize various biomass sources, including agricultural residues, forestry waste, and even municipal solid waste.
- Energy Recovery: The syngas produced can be further processed to generate electricity or other chemicals.
However, gasification does present challenges. Contamination and tar formation are common issues that can complicate downstream processing. Thus, refining methods must be employed to clean the syngas for further applications. Recent advancements in gasification technologies focus on improving efficiency and mitigating these challenges.
Pyrolysis
Pyrolysis involves the thermal decomposition of biomass in the absence of oxygen, leading to the formation of bio-oil, char, and syngas, which contains hydrogen. Typically occurring at temperatures between 300 and 900 degrees Celsius, the process produces high yields of bio-oil, which can be upgraded to fuel or chemicals.
The key features of pyrolysis include:
- Production of Bio-Oil: One significant advantage is the generation of bio-oil, a liquid form of energy which can be processed further.
- Flexible Operation: The process can be performed in batch or continuous systems, allowing for scalability based on local biomass availability.
- Co-Product Generation: Besides hydrogen, pyrolysis yields solid char, which can serve as a soil amendment or activated carbon.
Despite these benefits, pyrolysis has limitations, particularly regarding the quality of bio-oil. The oil can contain impurities that complicate its use as fuel. Recent studies have focused on improving oil quality and refining techniques to make it a more viable product.
Overall, thermochemical processes like gasification and pyrolysis present significant opportunities for generating sustainable hydrogen from biomass. As advancements continue in these areas, they hold promise for future hydrogen production frameworks.
Overall, thermochemical processes like gasification and pyrolysis present significant opportunities for generating sustainable hydrogen from biomass. As advancements continue in these areas, they hold promise for future hydrogen production frameworks.
Hydrogen Production Technologies: Current State
Understanding hydrogen production technologies is essential in comprehending the larger picture of biomass conversion into hydrogen. This section highlights the cutting-edge techniques and technological applications that currently dominate in the field of hydrogen production. Knowledge of these technologies allows for better assessment of their efficiency, scalability, and economic viability, all critical factors in making biomass hydrogen production a feasible energy solution.
Column and Batch Reactors
Column reactors and batch reactors serve distinct purposes in hydrogen production technology. Column reactors operate continuously, allowing for a constant flow of biomass to be processed. This setup is favorable for large-scale operations, as it maximizes throughput and minimizes idle time. The continuous flow promotes efficient use of resources, leading to higher productivity in hydrogen output.
On the other hand, batch reactors are suitable for smaller operations or when the biomass feedstock is varied. They allow for careful monitoring of reaction conditions and are adaptable to different types of biomass. This flexibility can lead to optimized production processes tailored to specific feedstock characteristics.
Both reactor designs have their advantages, which means selecting the right type depends on the project goals, biomass varieties, and desired production rates. Understanding these reactors allows researchers and industry participants to innovate in process design and enhance hydrogen yields effectively.
Pilot Plant Developments
The evolution of pilot plants has played a crucial role in advancing hydrogen production technologies from biomass. These facilities bridge the gap between laboratory research and full-scale industrial applications. They serve as testing grounds for new processes and technologies, enabling scientists and engineers to gather real-world data on efficiency, costs, and operational challenges.
Pilot plants often focus on demonstrating the scalability of specific processes, whether biochemical or thermochemical. They provide insights into the viability of these methods in a commercial setting. For instance, projects may explore the effectiveness of various catalysts in gasification or the optimum conditions for fermentation processes.
As more pilot projects transpire globally, the collective findings contribute immensely to the body of knowledge. They help identify best practices, technological shortcomings, and potential market applications. This iterative learning process fosters innovation and drives investment in hydrogen production technologies.
"Pilot plants are essential for validating research and demonstrating feasibility before commercialization. Their insights guide future investments and projects, enhancing overall success in the biomass hydrogen sector."
"Pilot plants are essential for validating research and demonstrating feasibility before commercialization. Their insights guide future investments and projects, enhancing overall success in the biomass hydrogen sector."
In summary, the current state of hydrogen production technologies encompasses a variety of reactor designs and pilot projects, each contributing unique advantages and insights. Understanding these components highlights the ongoing developments and their implications for making hydrogen a sustainable energy source derived from biomass.
Performance Metrics for Biomass-to-Hydrogen Processes
Understanding the performance metrics for biomass-to-hydrogen processes is crucial for assessing the efficiency and feasibility of hydrogen production. By evaluating these metrics, researchers and industry professionals can identify optimal pathways for converting biomass into hydrogen. Accurate performance metrics allow for the comparison of different technologies, promoting advancements in efficiency and reductions in cost. This section will explore the significant aspects related to hydrogen yield analysis and energy efficiency indicators.
Hydrogen Yield Analysis
Hydrogen yield analysis focuses on quantifying the amount of hydrogen produced per unit of biomass. This measure is essential to determine the viability of various biomass conversion methods. The yield can differ significantly based on the type of biomass used and the specific conversion technology employed.
To assess hydrogen yield effectively, the following key factors should be considered:
- Type of Biomass: Lignocellulosic materials often yield different quantities of hydrogen compared to algal or waste biomass. The composition of the biomass directly influences its hydrogen potential.
- Conversion Method: Whether through thermochemical or biochemical means, the method chosen can drastically affect the quantity of hydrogen produced.
- Operational Conditions: Parameters such as temperature, pressure, and the presence of catalysts can enhance or diminish hydrogen production.
Evaluating hydrogen yield not only aids in understanding current technologies but also identifies prospects for efficiency improvements and innovations.
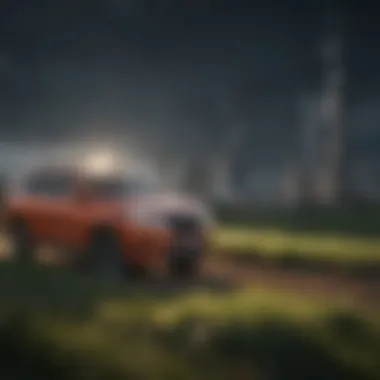
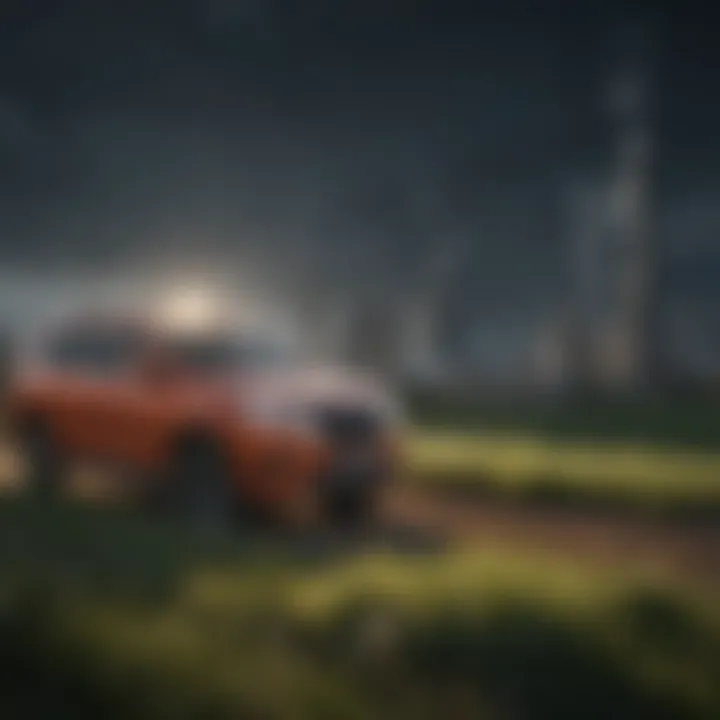
Energy Efficiency Indicators
Energy efficiency indicators are metrics that outline how effectively energy inputs convert into hydrogen outputs within biomass conversion processes. Understanding these indicators is crucial for organizations looking to optimize their processes and reduce environmental impact. Some vital elements to consider include:
- Input Energy Requirements: Measuring how much energy is needed to convert biomass to hydrogen, including pre-treatment processes, is vital. Lower input energy signifies higher efficiency.
- Conversion Efficiency: This indicator reflects the proportion of input energy that becomes hydrogen. A higher conversion efficiency indicates a more effective process.
- Overall System Efficiency: This metric encompasses not just the conversion efficiency but also factors in energy losses during transportation and storage.
"Understanding both hydrogen yield and energy efficiency indicators is key to realizing a sustainable and economically viable pathway for biomass-to-hydrogen production."
"Understanding both hydrogen yield and energy efficiency indicators is key to realizing a sustainable and economically viable pathway for biomass-to-hydrogen production."
Environmental Implications of Hydrogen from Biomass
The production of hydrogen from biomass holds great promise for sustainable energy solutions. As we explore this subject, it is key to consider various environmental implications. This topic delves into not just the advantages of biomass as a feedstock for hydrogen, but also the broader ecological impacts of adopting such technologies.
Lifecycle Analysis Considerations
Lifecycle analysis (LCA) is an essential methodology for assessing the environmental effects of biomass-to-hydrogen pathways. The process involves evaluating every stage, from biomass cultivation and harvesting through to hydrogen production and end-use. Each phase has associated input-output assessments that include energy consumption, greenhouse gas emissions, and resource utilization.
Key considerations in LCA for biomass hydrogen production include:
- Cultivation Impact: The method of biomass cultivation significantly affects the environment. Sustainable farming practices can mitigate soil degradation and enhance biodiversity.
- Transport Emissions: The distance biomass must travel to processing facilities can contribute to transportation emissions. Efficient logistics strategies can help minimize this.
- Production Processes: The technology used for hydrogen extraction, whether biochemical or thermochemical, can greatly influence overall energy efficiency and emissions.
- Waste Management: By-products from hydrogen production processes must be managed to prevent environmental harm.
Understanding these elements allows researchers and industry professionals to identify areas for improvement, leading to more sustainable practices in hydrogen production.
Greenhouse Gas Emission Reduction Potential
Hydrogen produced from biomass presents a unique opportunity for significant reductions in greenhouse gas emissions. Compared to conventional fossil fuels, biomass is considered a carbon-neutral resource when managed sustainably. The underlying principle is that the carbon dioxide emitted during hydrogen combustion is approximately equivalent to what the biomass absorbed during its growth.
In practical terms, some benefits include:
- Lower Carbon Footprint: Utilizing biomass, such as agricultural waste or dedicated energy crops, helps to lower the carbon intensity of energy systems.
- Negating Landfill Emissions: Organic waste used for hydrogen production can reduce methane emissions associated with landfilling. When biodegradable materials decompose without oxygen, they release methane, a potent greenhouse gas. Converting this waste into hydrogen diminishes such emissions.
- Enhanced Energy Systems: When integrated into existing energy portfolios, biomass-derived hydrogen can complement renewable energy sources by providing a stable and dispatchable energy supply.
"Hydrogen from biomass can create a pathway to a circular economy, where waste is minimized and energy is harnessed sustainably."
"Hydrogen from biomass can create a pathway to a circular economy, where waste is minimized and energy is harnessed sustainably."
Economic Perspectives on Biomass Hydrogen Production
Understanding the economic aspects of biomass hydrogen production is key for policymakers, researchers, and industry leaders. The production of hydrogen from biomass not only presents a potential for environmental sustainability but also opens doors to new market opportunities. As the world shifts toward more renewable energy sources, analyzing the economic viability of these technologies becomes increasingly crucial.
Cost-effective production techniques will determine how widely biomass hydrogen can be adopted. Various techniques, from fermentation to gasification, have different economic implications. Efficiency, scale, and the price of feedstock directly impact the overall cost. Investment in research to improve these processes can lead to breakthroughs that lower production costs, making hydrogen a more attractive option in the energy market.
Cost Analysis of Production Techniques
A thorough cost analysis of hydrogen production techniques is essential for understanding their feasibility. Several techniques exist, each with its own financial profile:
- Fermentation: This method relies on biological processes. Although initial costs may be lower due to simpler setups, the efficiency of hydrogen yield can vary. As a result, the economic return might depend on the type of biomass used.
- Gasification: This technique involves converting biomass into syngas at high temperatures. The costs here include not just operational expenses but also the infrastructure needed. While gasification can produce higher hydrogen yields, the capital cost may deter new projects.
- Pyrolysis: Similar to gasification but operates in the absence of oxygen. This method has low initial capital costs and can efficiently convert various biomass types. However, the selling price of hydrogen often remains challenging due to market standards.
Each of these methods has trade-offs in terms of capital and operational expenditures. A detailed review and comparison can point to which techniques might be more economically feasible under varying market conditions.
Market Potential and Demand Scenarios
The outlook for hydrogen produced from biomass reveals a growing market potential. As countries work toward sustainable energy goals, the demand for clean fuel is expected to rise.
Several factors contribute to this scenario:
- Government Incentives: Many nations are creating policies to support renewable energy projects, including biomass hydrogen. This can enhance profitability for new projects.
- Technological Improvements: Advancements in production methods could drive down costs, making biomass hydrogen competitive with other energy sources like natural gas.
- Public Awareness: Increasing public concern about carbon emissions is pushing demand for cleaner energy. Biomass hydrogen could satisfy this market requirement.
Though the market is promising, challenges remain. Competition from established fossil fuels and the need for infrastructure to support hydrogen delivery could affect demand projections. Nevertheless, developing robust market scenarios can help stakeholders prepare for future trends in the biomass hydrogen sector.
"The economic viability of biomass hydrogen production depends heavily on technological advancements and supportive policy frameworks."
"The economic viability of biomass hydrogen production depends heavily on technological advancements and supportive policy frameworks."
By addressing these economic perspectives, stakeholders can better evaluate the potential for biomass-derived hydrogen in future energy markets.
Challenges in Scaling Biomass Hydrogen Production
Scaling biomass hydrogen production is crucial to realizing hydrogen's potential as a sustainable energy source. Various factors present significant obstacles in this endeavor. Understanding these challenges is essential for researchers, industry professionals, and policy-makers aiming to advance hydrogen production technologies.
One of the primary issues is the accessibility of feedstock. Reliable and consistent feedstock supply is vital for large-scale hydrogen generation. The type and source of biomass impact the overall feasibility and efficiency of production processes. Access to lignocellulosic biomass, such as agricultural residues or dedicated energy crops, can vary by region, affecting logistics and costs. Additionally, competition for biomass from other industries, such as food production, raises concerns about long-term sustainability and economic viability. When biomass is scarce or expensive to obtain, it complicates the scaling of hydrogen production.
Feedstock Accessibility Issues
Feedstock accessibility issues encompass several elements. The first element involves the geographical limitations of biomass resources. Some regions may have an abundance of specific biomass types, while others may rely on imports, making logistics complex and costly. Proximity to biomass resources influences operational efficiency and economic feasibility, reinforcing regional disparities in hydrogen production capabilities.
Moreover, seasonality of biomass availability presents another challenge. Biomass crops may only be harvested during specific seasons, leading to supply fluctuations that can disrupt hydrogen production timelines. This variability makes it essential to develop storage solutions to maintain consistent hydrogen production throughout the year.
Finally, collaboration among different sectors — agriculture, energy, and waste management — is vital. Interdisciplinary partnerships can facilitate improved access to feedstocks by promoting effective management of resources. This may include utilizing agricultural residues and organic waste for hydrogen production, contributing to a circular economy model. To streamline biomass accessibility, policy frameworks that incentivize the use of waste and residual biomass should be explored.
Technological Barriers and Innovations Needed
Technological barriers present another significant challenge to scaling hydrogen production from biomass. Several processes for converting biomass to hydrogen, such as gasification and pyrolysis, require advanced technology. The complexity of these technologies can hinder development and implementation.
The efficiency of existing technologies poses an obstacle. While some methods provide a sustainable route for hydrogen production, they may still require optimization in terms of yield and energy consumption. Furthermore, existing technologies might not readily adapt to various biomass feedstocks, limiting their applicability.
To overcome these challenges, investments in research and development are paramount. Continued innovation can lead to the creation of new catalytic processes or improved reactor designs that enhance hydrogen yield. This could involve exploring novel materials for reactors or improving control systems for better energy efficiency.
Moreover, fostering collaborations between academia and industry can accelerate the transition of research from theory to practice. Pilot projects can serve as testing grounds for innovative technologies, providing valuable data to inform scale-up processes.
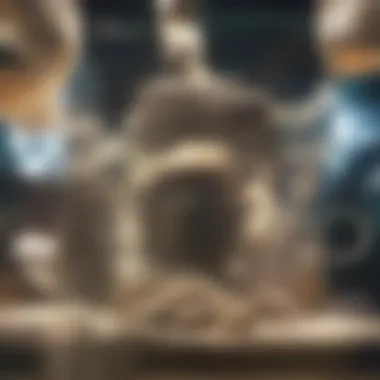
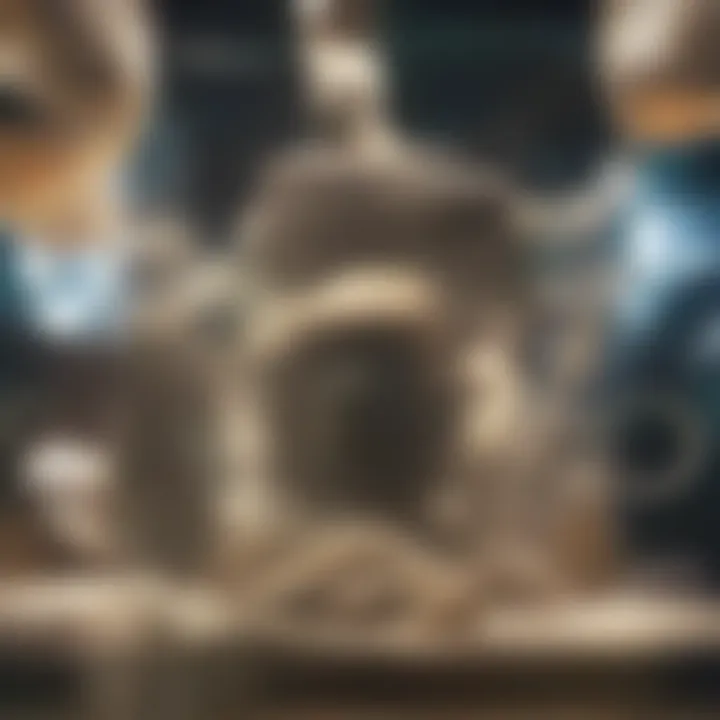
In addition, clearer regulatory frameworks are necessary to support innovation in hydrogen technologies. Policymakers must recognize the importance of enabling environment that encourages research funding and commercialization of new biomass-to-hydrogen technologies.
In summary, effective strategies that address feedstock accessibility and technological barriers are vital for scaling biomass hydrogen production. Overcoming these challenges is key to unlocking the full potential of hydrogen sourced from biomass.
Global Research and Development Initiatives
The global effort to harness hydrogen from biomass represents a significant stride in promoting sustainable energy practices. This section elucidates the vital role of research and development initiatives that span across borders, uniting scientists, engineers, and policy-makers. Understanding these collaborative undertakings is crucial to advancing technology, enhancing efficiency, and ultimately realizing the full potential of biomass as a hydrogen feedstock.
The importance of international collaboration cannot be overstated in this field. Many countries are beginning to realize that addressing energy challenges requires collective action. As hydrogen production technologies rapidly evolve, sharing knowledge and resources among nations helps to tackle common obstacles effectively. Projects that involve multi-national partnerships enable stakeholders to leverage diverse expertise, fostering innovation that can lead to breakthrough advancements in biomass hydrogen production.
Research initiatives also play a pivotal role in developing methodologies that can improve the efficiency of existing processes. This includes optimizing fermentation techniques or enhancing gasification methods that are fundamental to hydrogen generation. Furthermore, these initiatives can lead to the establishment of standardized metrics for performance evaluation across various biomass conversion technologies.
International Collaborative Efforts
International collaborative efforts are seen in various forms, including joint research projects, funding programs, and multinational conferences dedicated to biomass hydrogen production. Organizations such as the International Renewable Energy Agency (IRENA) actively promote collaboration among member countries.
"Collaboration is key to accelerating hydrogen production innovations and achieving sustainability goals globally."
"Collaboration is key to accelerating hydrogen production innovations and achieving sustainability goals globally."
Several significant partnerships have emerged, focusing on areas like:
- Shared research funding: Governments and institutions pooling resources to support transformative projects.
- Knowledge exchange programs: Initiatives allowing researchers to work across borders, sharing insights and fostering innovation.
- Conferences and workshops: Events that bring together leading experts to discuss recent findings and advancements in biomass hydrogen technologies.
This global approach not only helps address scientific and technological challenges but also aligns with broader climate and sustainability commitments set forth by many nations.
Case Studies in Biomass Hydrogen Projects
Examining various case studies can provide significant insights into the methodologies and results achieved through joint efforts in hydrogen production. These projects showcase innovative solutions and highlight best practices that can be replicated elsewhere.
- Sweden’s Biogas Program: This initiative exemplifies how organic waste can be converted into hydrogen-rich biogas. Through collaboration between local governments, universities, and businesses, Sweden has increased its hydrogen production capacity, demonstrating a model of circular economy in practice.
- Germany's Biomass Fuel Cells: Germany has initiated multiple projects focusing on integrating biomass with fuel cell technology. Collaborative efforts among research institutes and industry leaders aim to streamline technologies for more efficient hydrogen generation, while also exploring market deployment.
- US Department of Energy Projects: The U.S. has several national laboratory programs under its Department of Energy that emphasize biomass-to-hydrogen pathways. They foster collaborations with universities and tech companies to innovate in conversion technologies.
These case studies demonstrate that successful global research and development initiatives in hydrogen production from biomass can lead to technological advancement as well as scalable solutions that benefit energy needs and environmental targets.
Future Outlook for Hydrogen from Biomass
The future outlook for hydrogen production from biomass is critical for energy diversification and sustainability. As the world seeks to transition away from fossil fuels, the potential of utilizing biomass for hydrogen generation offers a promising avenue. This section will explore technological advancements and integration strategies that could enhance hydrogen production processes, thereby reducing fossil fuel dependence and promoting cleaner energy sources.
Technological Innovations on the Horizon
Recent research reveals numerous technological innovations poised to revolutionize hydrogen production from biomass. One significant area is the development of more efficient fermentation microorganisms. Such microorganisms can significantly increase hydrogen production rates and yields.
Key Innovations Include:
- Genetic Engineering: Researchers are focusing on genetically modifying strains of bacteria to enhance hydrogen production. This can lead to higher yields with less time and resources used.
- Advanced Gasification Techniques: Refinements in gasification methods have been a game changer. New reactors that optimize feedstock transformation could lead to higher hydrogen output.
- Nanotechnology: The application of nanomaterials in catalysis has shown great promise. They can enhance reaction rates and reduce energy input in thermochemical processes, making hydrogen production more feasible.
- Process Integration: Innovations in integrating different biomass conversion processes can streamline operations and minimize waste, thus optimizing hydrogen yield throughout various stages.
These technological strides will likely enable economies of scale, making biomass-derived hydrogen competitive with traditional hydrogen sources.
Integration with Existing Energy Systems
Another essential aspect of the future outlook involves the integration of hydrogen into current energy systems. This will be pivotal for maximizing the benefits of hydrogen as an energy carrier. With the increasing development of hydrogen infrastructure, several strategies are emerging.
Considerations for Integration:
- Blending Hydrogen with Natural Gas: This method allows for a gradual introduction of hydrogen into existing gas networks, aiding in the reduction of carbon intensity. While this does not entirely eliminate the use of fossil fuels, it can significantly lower greenhouse gas emissions.
- Hydrogen as a Storage Medium: Hydrogen can serve as an effective energy storage solution for fluctuating renewable energy sources such as wind and solar. This aids in balancing supply and demand without the energy loss associated with conventional storage solutions.
- Fuel Cell Technology: The integration of hydrogen fuel cells in transportation and stationary applications holds great potential. Advancement in fuel cell technology could lead to more efficient and widespread adoption.
- Collaborative Research Initiatives: Partnerships between government agencies, academia, and industry can lead to more robust solutions that consider regional energy needs. Such collaborations could facilitate smoother integration of hydrogen into the existing energy landscape.
Policy Frameworks Affecting Biomass Hydrogen Production
The production of hydrogen from biomass is not only influenced by technological advancements but also by policy frameworks that support or hinder its development. Effective policies can create an environment where biomass hydrogen production can flourish. These frameworks address regulatory considerations and incentives aimed at promoting sustainable practices.
Regulatory Considerations
Understanding regulatory considerations is crucial for the growth of biomass hydrogen production. Various governments implement regulations that dictate how biomass can be sourced, processed, and converted into hydrogen. These regulations may include:
- Environmental Protection Laws: These laws ensure that biomass sourcing and production practices do not lead to significant environmental degradation. Strict regulations can safeguard biodiversity while promoting sustainable sourcing.
- Bioenergy Policies: Many countries have specific bioenergy policies that outline acceptable practices for biomass generation. Compliance with these regulations is essential for producers aiming to enter the hydrogen market.
- Safety Standards: The production process involves several safety risks. Regulatory bodies often impose standards to protect workers and the environment from potential hazards associated with biomass processing and hydrogen production.
Failing to adhere to these regulations can lead to significant penalties. Therefore, a clear understanding of the regulatory landscape is crucial for stakeholders engaged in biomass hydrogen production.
Incentives for Sustainable Practices
Incentives play a key role in encouraging the adoption of biomass hydrogen production. By providing financial or regulatory benefits, governments can stimulate interest and investment in this sector. Some notable incentives include:
- Subsidies and Grants: Financial support can reduce the initial capital needed for setting up biomass-to-hydrogen facilities. This is particularly important for startups and smaller companies.
- Tax Breaks or Credits: Offering tax incentives for companies engaging in sustainable biomass practices can enhance their profitability while encouraging further investment in renewable energy.
- Research Funding: Allocating funds for research can spur innovation in biomass-to-hydrogen technologies. This leads to improved efficiency and lower costs, making hydrogen production more competitive.
"Sustainable incentives not only drive investment but also align economic growth with environmental responsibility."
"Sustainable incentives not only drive investment but also align economic growth with environmental responsibility."
In summary, policy frameworks are vital for the development of biomass hydrogen production. Regulatory considerations ensure safe and sustainable practices, while incentives encourage investment and innovation. A robust policy environment can significantly influence the viability and growth of this promising energy source.
Epilogue
In this article, we explored the multifaceted aspects of hydrogen production from biomass. The conversion of biomass into hydrogen is not only a promising avenue for sustainable energy but also holds significant environmental and economic benefits. Understanding the various mechanisms involved in this process highlights the importance of biomass as a renewable feedstock.
Recapitulation of Findings
The production of hydrogen from biomass can be approached through both biochemical and thermochemical processes. We examined two primary methods: fermentation, involving the breakdown of organic material by microorganisms, and thermochemical processes like gasification and pyrolysis of woody and residual biomass. Critical performance metrics, such as hydrogen yield and energy efficiency, play a vital role in evaluating the viability of these processes. Additionally, the environmental implications, including greenhouse gas reductions and lifecycle analysis considerations, were discussed, illustrating the sustainable potential of utilizing biomass for hydrogen production.
Final Thoughts on Future Developments
Looking ahead, the future of hydrogen production from biomass appears promising yet requires further attention to several factors. Innovations in technology will be crucial for enhancing the efficiency of conversion processes. Additionally, integration with existing energy systems can facilitate smoother transitions to sustainable practices. A coordinated effort involving regulatory frameworks and incentives for adopting green technologies will also support the growth of this industry. Continued research and international collaboration will remain imperative in overcoming existing challenges.
"The transition to sustainable energy sources hinges on our ability to innovate and integrate renewable practices into our daily lives."
"The transition to sustainable energy sources hinges on our ability to innovate and integrate renewable practices into our daily lives."
The potential of hydrogen from biomass could play a key role in achieving a more sustainable energy future.