Identifying Genetic Material: Techniques and Impact
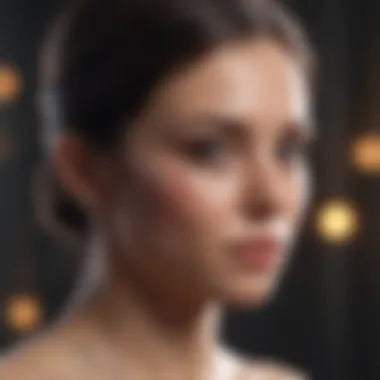
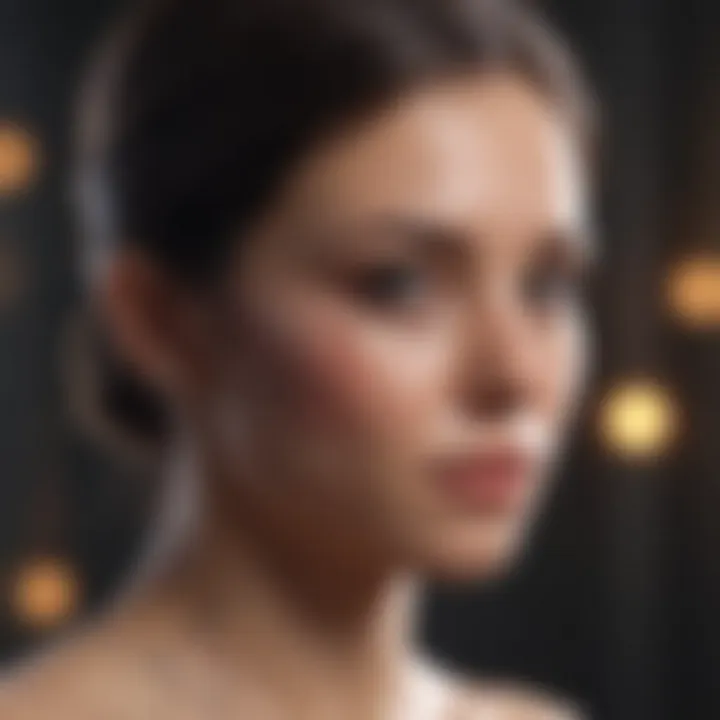
Article Overview
Purpose of the Article
In the vast and rapidly evolving field of genetics, the quest to identify specific genes is like searching for a needle in a haystack. This article aims to illuminate the various methodologies used for pinpointing these genetic gems, explaining their significance across multiple domains from medicine to agriculture. Understanding genetic material and how to locate specific genes is not just academic; it carries profound implications for advancements that can enhance food production, cure diseases, and even restore endangered ecosystems.
Relevance to Multiple Disciplines
The ability to identify genes in genetic material transcends disciplinary boundaries. In medicine, for instance, gene identification plays a pivotal role in developing personalized treatment plans. With the knowledge of an individual's genetic makeup, healthcare providers can tailor interventions that align more closely with a patient’s unique genetic profile. Similarly, in agriculture, discovering genes related to drought resistance or pest resilience can guide the breeding of crops that are better suited to withstand climate change. Furthermore, in environmental science, gene identification aids in assessing biodiversity, enabling strategies for conservation and understanding ecological networks.
Research Background
Historical Context
The journey to understanding genetic material is steeped in history. It began in the mid-19th century with Gregor Mendel's foundational work on inheritance, which laid the groundwork for classical genetics. Fast forward to the 20th century, the discovery of the DNA double helix by James Watson and Francis Crick marked a significant milestone. From this point, the race was on; researchers began to develop an arsenal of methods to unravel the complexities of genetic material, moving from rudimentary techniques to sophisticated technologies that utilize the power of bioinformatics.
Key Concepts and Definitions
To better grasp the methodologies discussed further in the article, some key concepts are crucial to define:
- DNA (Deoxyribonucleic Acid): The molecule that carries the genetic instructions for life.
- Gene: A segment of DNA that contains the coded instructions for making a particular protein.
- Genetic Mapping: The process of determining the location and chemical sequence of genes on a chromosome.
- Bioinformatics: The use of computer technology to manage and analyze biological data, especially in genomics.
"Identifying genetic material is like piecing together a puzzle, where each piece has its unique place in the grand picture of life."
"Identifying genetic material is like piecing together a puzzle, where each piece has its unique place in the grand picture of life."
In summary, understanding how to identify genetic material is a multifaceted endeavor. The implications stretch far and wide, impacting everything from health outcomes to environmental sustainability. As we delve deeper into the various methodologies, we will uncover the nuances involved in this fascinating journey of discovery.
Prelude to Genetic Identification
Genetic identification stands as a cornerstone in biological sciences, a field as intricate as it is crucial for numerous applications. Through understanding genetic material, researchers can pinpoint not just individuals but also uncover the underlying truths of evolution, diseases, and traits present in both flora and fauna.
Recognizing the significance of genes in biology can offer profound insights. Genes serve as instructions encoded in the very fabric of life, guiding processes responsible for development, function, and reproduction. In essence, identifying these segments of genetic material allows scientists to decode these biological blueprints, revealing the symphony of life unfolding within every organism.
The Importance of Genes in Biology
Genes are paramount in biology, serving as the basic units of heredity. The mechanisms through which they function dictate a range of biological phenomena.
- Heredity: Genes are responsible for the transmission of traits from parents to offspring. An in-depth understanding of how specific genes influence traits is crucial for everything from agriculture to medicine.
- Disease Mechanisms: Identifying genes linked to diseases permits targeted research that can lead to effective treatments. For example, pinpointing BRCA1 and BRCA2 genes is key in understanding breast cancer susceptibility, paving the way for preventative measures and individualized care.
- Evolutionary Biology: By studying genetic variation, scientists can make inferences about evolutionary processes, adaptation, and biodiversity. This understanding can help in conservation efforts and in combating the effects of climate change on species.
Thus, genes carry substantial implications not just for individual organisms but for entire ecosystems.
Historical Context of Gene Discovery
Looking at the historical context of gene discovery reveals how far science has come in understanding genetics. The mid-19th century was marked by Gregor Mendel, who laid down the foundations of genetics through his experiments with pea plants. His work, albeit unnoticed at the time, would eventually shape modern genetics.
- The Mendelian Revolution: Mendel introduced concepts of dominant and recessive traits, establishing a framework that would later contribute to the formulation of the laws of inheritance.
- Structural Discoveries: Fast forward to the 20th century; the discovery of the DNA double helix by James Watson and Francis Crick in 1953 marked a significant leap. It was not just about inheritance anymore—understanding the molecular structure of DNA opened the floodgates to a whole new realm of genetic research.
- The Human Genome Project: The late 20th century brought initiatives like the Human Genome Project, a collaborative effort aiming to wholly map the human genome. This endeavor highlighted the importance of genetic identification, revealing not just our similarities but vital differences that underpin health and disease.
The historical trajectory of gene discovery is a testament to humanity's relentless quest for knowledge in the realm of genetics, illuminating pathways that have transformative implications in modern science.
Understanding Genome Structure
Understanding the structure of the genome is fundamental when it comes to identifying genetic material. An intricate arrangement of DNA, the genome's architecture plays a pivotal role in how genetic information is processed, expressed, and inherited. When researchers delve into the complexities of the genome, they gain insights that are applicable in various scientific realms, from elucidating genetic disorders to enhancing agricultural traits.
The value of having a firm grasp on genome structure lies in the way it informs methodologies for gene identification. For instance, comprehending how chromosomes are organized leads directly to more effective mapping techniques. It allows scientists to pinpoint regions of interest with precision, ultimately yielding better outcomes when investigating specific genes that might be responsible for diseases or valuable characteristics in crops.
Chromosomes and Their Organization
Chromosomes are like the filing cabinets of genetic information. When we think about chromosomes, we’re dealing with neatly packaged strands of DNA, containing both genes and regulatory elements. Each species has a characteristic number of chromosomes, and they vary widely – for example, humans possess 46 chromosomes arranged in 23 pairs, while fruit flies have only 8.
Understanding how chromosomes are organized sheds light on several key points:
- Chromosome Structure: In a way, chromosomes can be viewed as physical manifestations of genetic inheritance. Their structure—composed of chromatin, a mix of DNA and protein—creates both spatial and functional organization. This architecture is critical in allowing the DNA to be compacted yet accessible for transcription and replication.
- Gene Density: Different regions of chromosomes have different levels of gene density. Some areas house numerous genes tightly packed together, while others might be gene-poor. This heterogeneity influences how genetic information is activated in response to cellular needs.
- Centromeres and Telomeres: These are vital components of chromosomes. Centromeres play a critical role during cell division, ensuring that each daughter cell receives the correct number of chromosomes. Telomeres, on the other hand, cap the ends of chromosomes and protect them from degradation, which is crucial for maintaining genome integrity.
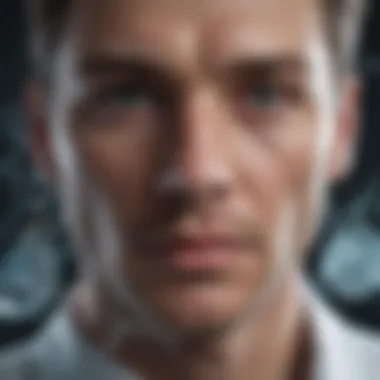
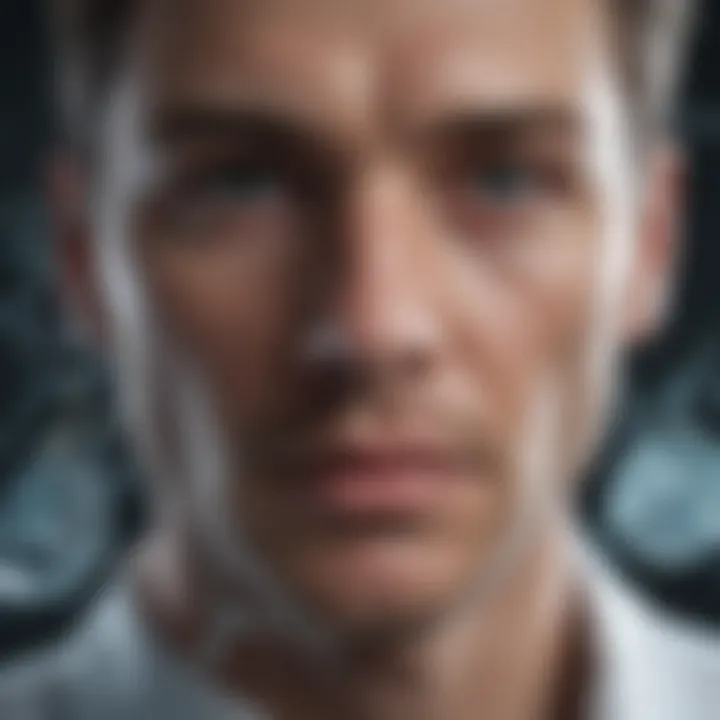
Understanding chromosome organization is also fundamental in modern genetic research. This allows for targeted approaches when investigating genetic disorders or developing biotechnological applications.
DNA Sequence and Genetic Code
At the core of genetics lies the DNA sequence — the precise order of nucleotides (adenine, thymine, cytosine, and guanine) that defines genetic information. This sequence not only determines the traits of organisms but also encodes proteins that perform a vast array of functions within the body.
The significance of the DNA sequence and the genetic code can hardly be overstated:
- Coding vs. Non-coding Regions: Not all DNA sequences code for proteins. Approximately 98% of human DNA does not code for proteins and includes regulatory elements that control gene expression. Identifying both coding and non-coding regions is crucial for understanding the complex web of genetic regulation.
- Mutations and Variability: Small changes in the DNA sequence can lead to mutations, potentially resulting in phenotypic variations. Understanding how these variations occur and their implications on health and disease is a central theme in genetics. For instance, a single nucleotide polymorphism (SNP) can affect an individual's risk for certain diseases.
- Genetic Code: The genetic code itself is a language made up of triplet combinations of nucleotides that dictate which amino acids are assembled to form proteins. Understanding this code is essential, as it lays the groundwork for advancements in gene therapy and biopharmaceuticals.
In summary, both the organization of chromosomes and the understanding of DNA sequences are essential for advances in genetics, helping researchers to map out the intricate connections between genes, traits, and biological functions.
"Understanding genome structure is not just an academic exercise; it shapes the future of agriculture, medicine, and environmental sustainability."
"Understanding genome structure is not just an academic exercise; it shapes the future of agriculture, medicine, and environmental sustainability."
By examining these fundamental elements, we can better appreciate the tools and approaches we have at our disposal for identifying genetic material and addressing the implications that accompany such discoveries.
Classical Techniques for Gene Finding
Classical techniques for gene finding are foundational methods that continue to play a critical role in genetics research. Despite the emergence of modern technologies, understanding these classical approaches allows researchers to appreciate the historical context and the evolution of genetic identification. These methods are not merely relics of the past; they provide vital insights into the mechanisms of heredity and the complex interplay of genes in organisms.
Moreover, classical techniques such as genetic mapping and phenotypic correlation studies have paved the way for the development of advanced methodologies. They also remind us of the importance of rigorous experimentation and observation in science. While the accuracy and efficiency of these techniques might not match the newest technologies, they offer a level of simplicity and accessibility that continues to be valuable in specific contexts.
Genetic Mapping Fundamentals
Genetic mapping serves as a cornerstone in the quest to identify and locate genes. This technique provides a visual representation of the genetic architecture of an organism. It illustrates how genes are situated on chromosomes, revealing their proximity to one another, which can hint at their collective function. Two major components of genetic mapping are linkage analysis and recombination frequencies, each with unique significance.
Linkage Analysis
Linkage analysis is crucial for understanding the genetic relationships within families or specific populations. A key characteristic of this technique is its ability to identify genes that are inherited together more often than would be expected by chance. This feature makes linkage analysis a beneficial choice for locating genes associated with particular traits or diseases.
In practice, it works by analyzing the co-segregation of traits in family studies, allowing researchers to map the position of a gene relative to known markers. One unique feature of linkage analysis is its reliance on observable phenotypes, making it a powerful tool for studying inherited diseases. However, a drawback is that it may require large families or specific populations and can sometimes lead to ambiguous results due to recombination events.
Recombination Frequencies
Recombination frequencies, on the other hand, give insight into the probability that two genes will be inherited together. This measurement is taken between two loci on a chromosome and reflects their physical distance apart. The key characteristic of recombination frequencies is that they help geneticists understand the genetic map of organisms by estimating distances based on the frequency of recombined offspring.
Using this method, scientists can create detailed genetic maps, which are essential for gene identification. A unique aspect of recombination frequencies is their quantifiable nature, allowing researchers to assign a numerical value to genetic distances. However, one advantage/disadvantage is that high recombination rates can complicate linkage predictions, leading to difficulties in establishing precise genetic maps.
Phenotypic Correlation Studies
Phenotypic correlation studies focus on the relationship between observable characteristics and underlying genetic factors. By examining how various phenotypes co-vary, researchers can identify potential genetic connections that may not be immediately obvious. This method skillfully marries observation with genetic analysis, enriching the understanding of gene function and its influence on traits.
These studies provide substantial insights into how traits are expressed and influenced by genetic variations, offering investigators clues about the underlying genetic mechanisms. By linking phenotypic data to genotype information, researchers can establish connections that help paint a clearer picture of gene-environment interactions, a subject of growing importance in the realm of genetics and genomics.
Modern Techniques in Gene Identification
Modern techniques in gene identification have reshaped how researchers approach the vast and complex world of genetic material. Unlike the classical methods that often relied on slower, more manual processes, these contemporary strategies harness technology to expedite and enhance accuracy in gene discovery. Innovating these methodologies can lead to breakthroughs in understanding genetic diseases, improving agricultural traits, and navigating environmental challenges. As the landscape of genetics evolves, so do the techniques that allow scientists to delve deeply into the intricacies of genomes.
Next-Generation Sequencing Technologies
Next-generation sequencing (NGS) technologies stand at the forefront of modern gene identification, offering unprecedented speed and scale in analyzing genetic sequences. Among the notable methods, Whole Genome Sequencing and Targeted Sequencing each play critical roles.
Whole Genome Sequencing
Whole Genome Sequencing (WGS) involves determining the complete DNA sequence of an organism’s genome at a single time. This exhaustive approach is valuable because it captures both coding regions and non-coding sequences, presenting a holistic view of the genetic material. One key characteristic of WGS is its ability to reveal variations across entire genomes, allowing researchers to pinpoint genetic anomalies associated with diseases.
Why choose WGS? Primarily, it's a popular choice due to its comprehensive nature. By providing a complete genetic blueprint, it becomes easier for scientists to explore genetic complexities and variations that might otherwise go unnoticed. A unique aspect of WGS is its applicability in personalized medicine, giving insights into how individual genetic landscapes can influence treatment responses.
However, WGS does come with its own set of challenges. The vast amount of data generated demands robust computational tools for analysis, which can be resource-intensive. Moreover, interpreting the non-coding regions of the genome can be confusing, as their functions are still not entirely understood. Despite these hurdles, the advantages often outweigh the disadvantages, making WGS a central player in gene identification methodologies.
Targeted Sequencing
Targeted Sequencing, in contrast, focuses on specific regions of interest within the genome. This technique is adept at examining selected gene sets that are known to be linked to certain diseases or traits. The advantage of targeting specific regions lies in the cost-effectiveness and speed—researchers can obtain actionable data without wading through the entire genome.
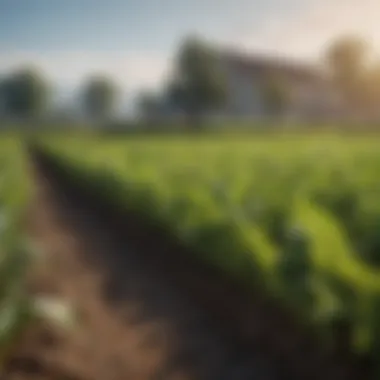
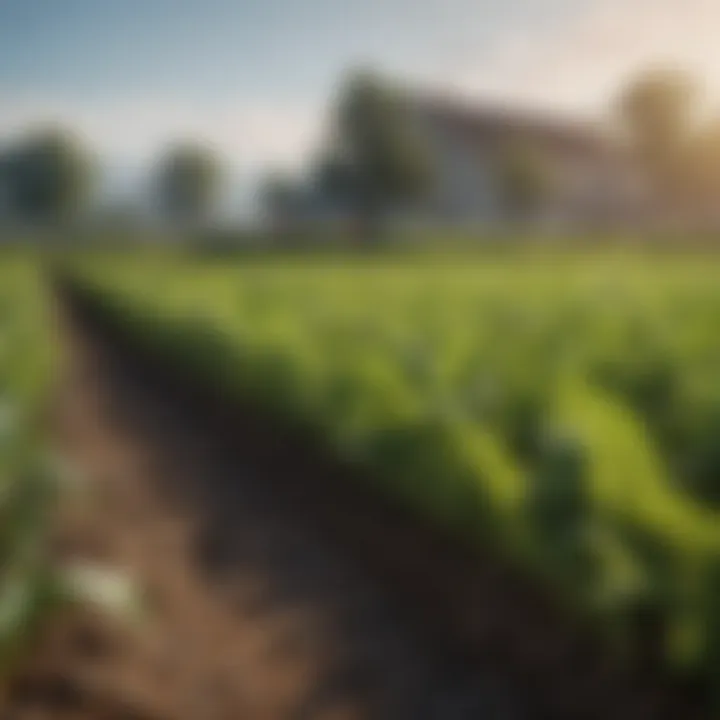
The key characteristic of Targeted Sequencing is its efficiency; by narrowing down to areas of interest, it reduces the amount of sequencing required. This makes it beneficial for studies aimed at understanding particular genetic disorders or traits without the need for the comprehensive scope of WGS.
While Targeted Sequencing offers lower costs and faster results, it can suffer from some limitations. For example, important genetic variations outside the targeted areas may be overlooked, that could provide further insights into the genetic basis of diseases. Still, when used strategically, Targeted Sequencing becomes a powerful tool in the genetic research toolbox.
CRISPR and Gene Editing
CRISPR technology has revolutionized gene editing and, consequently, the field of gene identification. It stands out due to its accuracy, efficiency, and flexibility. CRISPR allows scientists not just to identify genes, but to modify them with precision, enabling a deeper understanding of gene functions and interactions. Its implications are profound—ranging from correcting genetic mutations that cause diseases to enhancing crop resilience against climate change. The potential applications are vast, but ethical considerations must always be at the forefront to mitigate risks associated with gene editing technologies.
Bioinformatics in Gene Discovery
Bioinformatics plays a vital role in gene discovery by merging biology with computer science to analyze large datasets. The methodologically robust nature of bioinformatics can help unravel the complexities of genetic information. With the deluge of data from various gene sequencing technologies, bioinformatics is becoming an indispensable tool for researchers. Advances in computational techniques, statistics, and machine learning have elevated the accuracy and efficiency of identifying genetic material.
Data Analysis Techniques
Genome Annotation
Genome annotation is the process of identifying the locations of genes and coding regions in a genome. This step is crucial because it transforms raw nucleotide sequences into functional insights. One key characteristic of genome annotation is its integration of biological data with computer algorithms, allowing for assessment of gene function based on where they are positioned within the genome.
The benefit of this method is clear—accurate annotations help to predict gene function, which in turn can inform drug discovery or disease identification. For example, accurately annotated genomes facilitate targeted research in genetic disorders and contribute to personalized medicine approaches.
However, one unique feature of genome annotation is the reliance on existing databases for functional assignments, which can be a double-edged sword. While leveraging cross-species data does enhance annotation quality, it may also introduce biases if the reference genome is from a genetically distant organism. Thus, while genome annotation is fairly popular for its utility, researchers must tread carefully to account for these limitations.
Comparative Genomics
Comparative genomics takes a broader perspective, examining the similarities and differences in gene content between different organisms. This technique sheds light on evolutionary relationships and can unveil conserved genes that are crucial for fundamental biological processes. A key characteristic here is its ability to draw insights from multiple genomes, which can help identify functionally important genetic elements that are conserved across species.
The primary advantage of comparative genomics lies in its potential to pinpoint homologous genes, helping scientists make informed hypotheses about gene function based on evolutionary conservation. Such analysis is extremely beneficial, particularly in understanding genetic diseases: if a gene is found to be conserved across species, it likely plays a significant role in vital physiological processes that can impact health.
Yet, the unique feature of comparative genomics also brings its challenges. The interpretation of comparative data may be complicated by genetic drift and varying selective pressures faced by different species, which can obscure the true function of a gene. Overall, while the advantages of comparative genomics are considerable, they must be weighed against these complex evolutionary dynamics.
Software Tools for Gene Prediction
The proliferation of software tools dedicated to gene prediction has revolutionized how genetic material is identified and characterized. These tools employ sophisticated algorithms and statistical models to predict gene locations, sequences, and functions. In today's landscape, utilizing the appropriate software can significantly speed up the research process and enhance precision.
Gene prediction software can vary widely in terms of capabilities and focus areas. For instance, some focus on specific organisms while others are designed for a wide range of species. The effective use of these tools depends on a researcher’s familiarity with their functionalities and their suitability for particular projects. Notably, combining multiple tools can often yield more reliable predictions, as each has its own strengths and weaknesses. This multidimensional approach is beneficial, providing a comprehensive strategy for gene identification and characterization.
"The fusion of computational power with biological insights forms the backbone of modern genetic discovery, dramatically reshaping our understanding of life's blueprint."
"The fusion of computational power with biological insights forms the backbone of modern genetic discovery, dramatically reshaping our understanding of life's blueprint."
As bioinformatics continues to evolve, it's becoming clear that its role in gene discovery will only grow more significant, making it an exciting frontier for researchers eager to delve deeper into the complexities of genetics.
Applications of Gene Finding
Identifying specific genes carries significant weight in several fields, and it paints a picture of how these discoveries are woven into the fabric of societal advancements. The applications span across medicine, agriculture, and environmental considerations, making the subject of gene finding not merely an academic pursuit, but a tangible part of everyday life.
Biomedicine and Genetic Disorders
In the realm of biomedicine, the identification of genes associated with genetic disorders is a game changer. By pinpointing the genetic underpinnings of diseases such as cystic fibrosis or Huntington's, researchers can develop targeted therapies that go beyond general treatments. For instance, knowledge of specific mutations allowed scientists to create drugs tailored to help manage symptoms rather than just mask them.
Moreover, gene finding fuels the burgeoning field of personalized medicine. Treatment plans can now be customized based on genetic profiles. Such advancements increase the likelihood of treatment success while minimizing side effects, ultimately revolutionizing patient care. Integrating genetic testing into routine healthcare could potentially alter how we approach diagnostics.
"Understanding the genetic basis of diseases unlocks doors that were previously unseen."
"Understanding the genetic basis of diseases unlocks doors that were previously unseen."
Agricultural Biotechnology
The significance of gene finding in agriculture stems from its ability to enhance crop yields and resistance to pests. By identifying genes that confer drought resistance, for example, breeders can introduce these traits into existing crops. This is not just about better harvests; it's about food security in an era of climate change. Farmers are particularly interested in cultivating crops that can withstand harsh conditions, ensuring sustainability through innovative methodologies.
Moreover, gene finding can lead to the creation of biofortified crops that are nutritionally superior. One exhilarating example is the development of Golden Rice, which has been engineered to contain higher levels of vitamin A. This is a crucial advancement, especially in regions where deficiencies in essential nutrients are rampant. Such efforts in agricultural biotechnology illustrate how gene identification translates into real-world benefits.
Environmental Applications
The environment benefits from gene identification in surprisingly intricate ways, particularly through conservation efforts. Scientists can identify genetic variations within endangered species, aiding in the development of strategies aimed at preserving biodiversity. These methods also assist in assessing the resilience of species to changing climates.
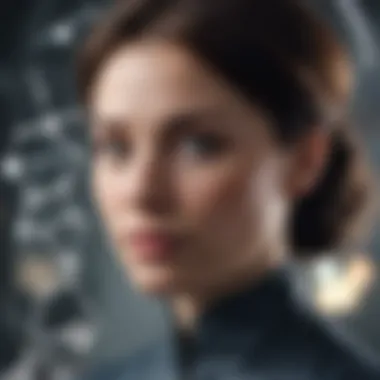
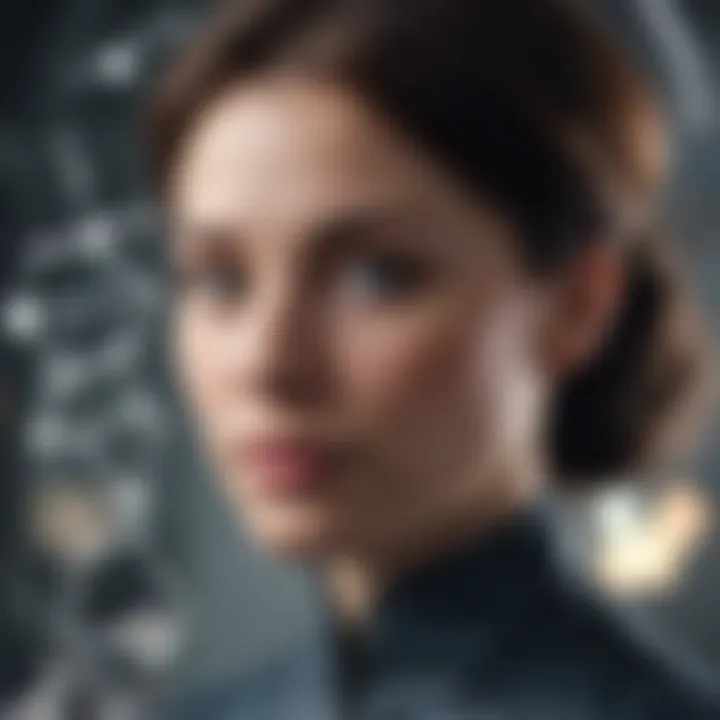
Furthermore, gene finding plays a pivotal role in bioremediation. Certain microorganisms have been found capable of degrading pollutants, and understanding their genetic make-up allows for the enhancement of these properties. For example, by identifying and manipulating genes that enable bacteria to break down oil spills, environmental scientists can develop more effective cleanup strategies. The implications of these discoveries are profound, not only rejuvenating ecosystems but also fostering a more sustainable approach to managing human impact on the planet.
In summary, the applications of gene finding are far-reaching and multifaceted. As science continuously advances, the impact of accurately identifying and manipulating genetic material emerges as a critical tool for addressing some of the United Nations' Sustainable Development Goals, particularly those centered on health, food security, and environmental sustainability.
Challenges in Gene Identification
Identifying genetic material is no walk in the park; it is rather akin to navigating a labyrinth without a map. There are myriad challenges that researchers face as they quest to pinpoint specific genes. Understanding these challenges is crucial not just for advancing scientific knowledge but also for applying that knowledge in fields like medicine, agriculture, and environmental science.
One of the core challenges that arise in gene identification stems from the inherent complexity of the genome. Gene regions can vary dramatically in size and organization, and the sheer volume of data generated by modern sequencing technologies can be overwhelming. This often leads researchers to confront issues of accuracy and efficiency in data interpretation. Moreover, genes don't function in isolation. They interact with each other and with their environment, further complicating the identification process.
Complexity of the Genome
The human genome alone contains about three billion base pairs that house roughly 20,000-25,000 genes, each with unique sequences and functions. The structure is not only intricate but also varied, including coding regions, introns, and regulatory elements.
- Non-coding DNA: A considerable portion of the genome does not code for proteins. Understanding the role of this non-coding DNA, often termed "junk DNA," represents a daunting task. Some of it may have regulatory functions or even play roles in diseases.
- Gene families: Many genes are part of families that have evolved through duplication events. Distinguishing one gene from another within these families can pose a challenge when fast sequencing techniques churn out vast amounts of similar sequences.
- Alternative splicing: This phenomenon enables a single gene to produce multiple products, complicating the mapping of gene functions.
Overall, the tumultuous landscape of genomic complexity necessitates advanced methodologies in data collection and analysis.
Ethical Considerations in Genetic Research
The dilemmas surrounding gene identification extend beyond scientific hurdles; ethical considerations loom large as well. How do researchers navigate the moral minefield of manipulating genetic material? Researchers often grapple with several ethical concerns:
- Consent and privacy: Obtaining informed consent can be quite challenging, especially when working with genomics that involve sensitive health data. Safeguarding privacy remains paramount.
- Genetic discrimination: Accidental misuse of genetic information may result in discrimination regarding insurance or job opportunities. This reality poses a significant ethical concern that could harm individuals and communities.
- Biotechnology applications: While the benefits of gene identification might bolster agriculture, it raises questions about genetically modified organisms (GMOs) and their effects on biodiversity and health, making it hard to chart a clear path forward.
The intersection of ethics and genetics prompts vital questions about how to responsibly manage the potential applications of gene discovery.
The intersection of ethics and genetics prompts vital questions about how to responsibly manage the potential applications of gene discovery.
Navigating these challenges requires a blend of scientific rigor and ethical mindfulness. It's essential that as we advance our understanding of genetics, we also cultivate frameworks that prioritize the well-being and rights of individuals and communities involved.
As we venture into the future of genetic research, acknowledging these challenges will pave the way for responsible and innovative breakthroughs.
Future Directions in Gene Finding
The field of gene identification is undergoing rapid transformation fueled by new technologies and interdisciplinary collaborations. As scientists and researchers continue to decode the intricate roles genes play in development and disease, it's imperative to explore what lies ahead. Future directions in gene finding not only promise new methodologies but also heightened understanding of genetic material, which could lead to significant breakthroughs across various sectors.
Innovations in Technology
One cannot ignore the pivotal role that technological advancements play in gene discovery. A few significant innovations stand out, transforming how researchers approach genetic identification:
- Artificial Intelligence (AI): AI algorithms can analyze vast amounts of genomic data more rapidly and accurately than traditional methods. For instance, machine learning techniques are being employed to predict gene locations and functions based on existing data. The ability to recognize patterns that may elude even seasoned geneticists might redefine future research methodologies.
- Single-Cell Sequencing: Instead of analyzing bulk DNA, single-cell sequencing allows researchers to examine the genetic material of individual cells. This innovation yields insights into cellular diversity within tissues, highlighting how differences at the cellular level can affect overall gene expression. This granular approach can lead to the discovery of novel gene functions that simply cannot be observed at a population level.
- Synthetic Biology: This emerging field combines biology and engineering, allowing scientists to design and construct new biological parts, devices, and systems. With synthetic biology, gene identification can evolve into creating entirely new biological pathways, making it possible to analyze gene function in unprecedented ways.
"Technological progress in gene identification bridges gaps between theoretical research and practical applications, sparking a revolution in biomedicine and beyond."
"Technological progress in gene identification bridges gaps between theoretical research and practical applications, sparking a revolution in biomedicine and beyond."
Interdisciplinary Approaches to Genetics
Today's scientific challenges require an integrative approach, marrying knowledge from various fields. The future of gene finding will increasingly lean on these interdisciplinary strategies:
- Collaboration with Data Science: With the explosion of genetic data, collaboration between genetics and data science is crucial. Analyses that combine statistical methods from mathematics and biology can yield deeper insights. As geneticists work closely with data scientists, the chances of developing sophisticated models that predict gene behavior will increase exponentially.
- Ethics and Social Implications: Just as technology advances, so do the ethical considerations surrounding gene identification. An interdisciplinary approach that includes bioethics will ensure that the implications of genetic research are fully considered. Understanding how genetic information impacts individuals and societies will be vital as new discoveries emerge.
- Integration with Environmental Science: The relationship between genetics and environmental factors is becoming clearer. Research that considers genetic material alongside environmental variables will advance our knowledge of adaptations and evolutionary processes. This understanding is essential for fields like agriculture and conservation, where gene-finding efforts could inform practices that support biodiversity and sustainability.
As the landscape of gene identification evolves, these future directions underscore a need for ongoing adaptation and collaboration across various disciplines. Researchers will not just be identifying genes but also unraveling their roles within complex networks and systems, ultimately making strides in fields like personalized medicine, crop resilience, and environmental stewardship.
Epilogue
The concluding segment of this article serves to wrap up the extensive exploration into the realm of genetic identification methodologies. The importance of understanding these methods cannot be understated, as they lay the groundwork for both innovative research and practical applications in various domains. Identifying genetic material not only contributes to scientific knowledge but also drives tangible advancements in medicine, agriculture, and environmental stewardship. The future of genetics hinges on the evolution of these methodologies, requiring continuous refinement and adaptation to emerging challenges.
The Ongoing Journey of Gene Discovery
The pursuit of uncovering the intricacies of gene function is an ongoing journey. As science progresses, new technologies and methodologies present themselves as pivotal tools for researchers embarking on this path. Here are some noteworthy elements regarding this journey:
- Generational Innovations: Each decade brings forward cutting-edge technologies. From early genetic mapping to today’s transformative CRISPR techniques, these innovations underscore a larger trend where continuously evolving methodologies shape our understanding of genetics.
- Global Collaboration: The scope of gene discovery spans continents, involving international collaborations that enhance knowledge-sharing and resource pooling. This collective effort often leads to breakthroughs that wouldn't be achievable in isolation.
- Public Impact: The implications of genetic discovery extend beyond laboratories. The identification of genes linked to diseases holds the promise of tailored therapies, aiding in the treatment of genetic disorders. Similarly, efforts in agricultural biotechnology can lead to improved crop resilience, which is crucial amidst climate change challenges.
- Ethical Considerations: As we tread further into genetic frontiers, ethical considerations remain paramount. The implications of gene editing and data privacy cannot be overlooked, necessitating robust discussions within the scientific community and beyond.
In sum, the ongoing quest for genetic discovery is a testament to human curiosity and ingenuity. The challenges faced today will likely forge new pathways and expand understanding, proving that the journey of genetic identification is far from complete. In this regard, both students and professionals are encouraged to embrace this dynamic field as it continues to unfold, shaping our future in profound ways.
"The art and science of gene discovery is woven with curiosity, ethics, and unyielding pursuit of knowledge."
"The art and science of gene discovery is woven with curiosity, ethics, and unyielding pursuit of knowledge."
In closing, as we reflect on the methodologies and implications discussed, it becomes evident that the future of genetics is both promising and complex. It invites informed dialogue among all stakeholders in the field.