Innovations in Electron Microscopy: A New Era
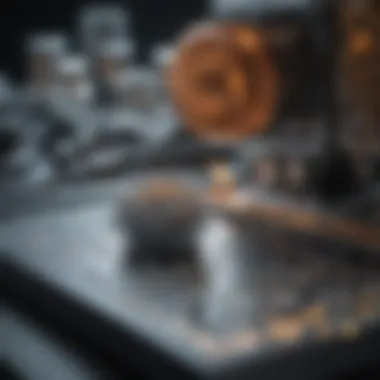
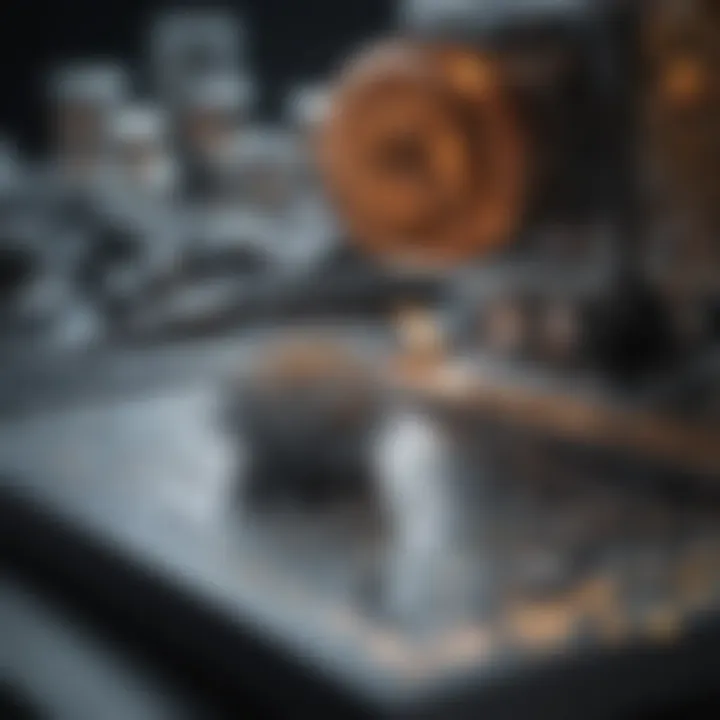
Intro
The interest in modern electron microscopy has surged in recent years. This is driven by the demand for high-resolution imaging capabilities across a variety of scientific fields. The innovations in this area are not just technical upgrades; they promise to impact biology, materials science, and nanotechnology, among others. By understanding these developments, researchers can harness their potential effectively.
Article Overview
Purpose of the Article
The aim of this article is to explore the innovations behind new electron microscopy technologies. By examining their operational principles, we can understand how they differ significantly from older models. This discussion will unveil important advancements such as cryo-electron microscopy and scanning transmission electron microscopy. Their implications for basic and applied sciences are crucial for modern research practices.
Relevance to Multiple Disciplines
These advancements are critically useful in several scientific disciplines:
- Biology: New electron microscopes allow detailed visualization of cellular structures and dynamics.
- Materials Science: High-resolution imaging assists in determining material properties and structures at the nanoscale.
- Nanotechnology: Observing nanoscale phenomena is essential for developing new technologies.
Such technologies span a wide range of applications, making them vital for not only researchers but also educators and professionals in various fields.
Research Background
Historical Context
Electron microscopy has a storied history stretching back to its inception in the early 20th century. Early models were limited in resolution and required significant user expertise. As technology progressed, the introduction of transmission and scanning electron microscopes marked significant milestones. Today, the focus is on enhancing resolution and enabling new methods of imaging.
Key Concepts and Definitions
To better understand the innovations in electron microscopy, it is important to clarify a few concepts:
- Cryo-Electron Microscopy (cryo-EM): A technique that allows researchers to observe specimens at cryogenic temperatures, preserving their natural state.
- Scanning Transmission Electron Microscopy (STEM): Combines features of both scanning and transmission electron microscopy to provide high-resolution images and information about the material.
These definitions set the stage for a deeper exploration of the innovations in electron microscopy technology, which will be discussed in further sections.
Prologue to Electron Microscopy
Electron microscopy plays a crucial role in modern scientific research. It serves as a powerful tool that allows scientists to visualize structures at the nanoscale level with precision and detail. This level of imaging is essential in various fields such as biology, material science, and nanotechnology. With the advancements in electron microscopy technology, researchers can explore complex biological systems, analyze nanomaterials, and characterize material defects more effectively than ever before.
Historical Context
The origins of electron microscopy can be traced back to the early 20th century. The development of the first transmission electron microscope by Ernst Ruska and Max Knoll in 1931 marked a significant milestone. This invention allowed for higher magnifications than traditional optical microscopes, which rely on visible light. Since then, advancements have led to various types of electron microscopes, including scanning electron microscopes, and more recently, cryo-electron microscopy techniques. Each innovation has contributed to a deeper understanding of materials and biological processes, pushing the boundaries of what can be visualized.
The historical context of electron microscopy is not only about technological evolution. It reflects the growing demands for detailed analysis in scientific applications. Researchers needed tools that could meet the challenges posed by complex samples. As such, the development of these microscopes has been driven by the need for precision in imaging, enabling studies in areas like medicine, materials research, and nanotechnology.
Principles of Electron Microscopy
Electron microscopy operates on fundamental principles that differ significantly from light microscopy. It uses beams of electrons instead of light to illuminate specimens. When electrons strike a sample, they interact with the atoms in the material, creating various signals that can be captured and analyzed.
The key principles include:
- Electron Beam Generation: Electrons are emitted from a heated filament and focused into a coherent beam through electromagnetic lenses.
- Interaction with Sample: The electrons penetrate or scatter off the specimen, depending on the type of microscope used. This interaction forms the basis for obtaining images of internal structures.
- Image Formation: Detectors capture the signals, which are then processed to generate high-resolution images. This process allows for the visualization of intricate details at much higher resolutions than optical methods.
Overall, understanding these principles enhances the appreciation of how electron microscopes function and their potential applications. Emphasizing these capabilities helps to elucidate the innovations made in recent years, supporting advancements in scientific research. For a detailed study, scholars may refer to resources such as Wikipedia .
Electron microscopy provides insights that are often unattainable through other means, revolutionizing the way we study materials and biological phenomena.
Electron microscopy provides insights that are often unattainable through other means, revolutionizing the way we study materials and biological phenomena.
Types of Electron Microscopes
Electron microscopy has evolved considerably since its inception, resulting in various types of microscopes tailored to specific applications and requirements. Understanding the types of electron microscopes is essential for choosing the right one for a given research task. The following three main types offer unique capabilities and advantages that cater to diverse scientific fields.
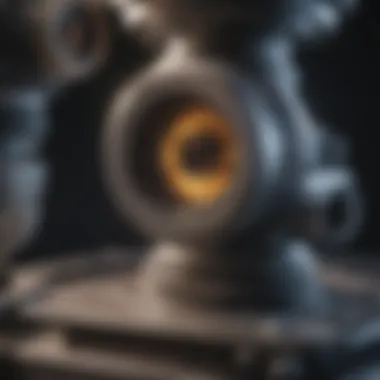
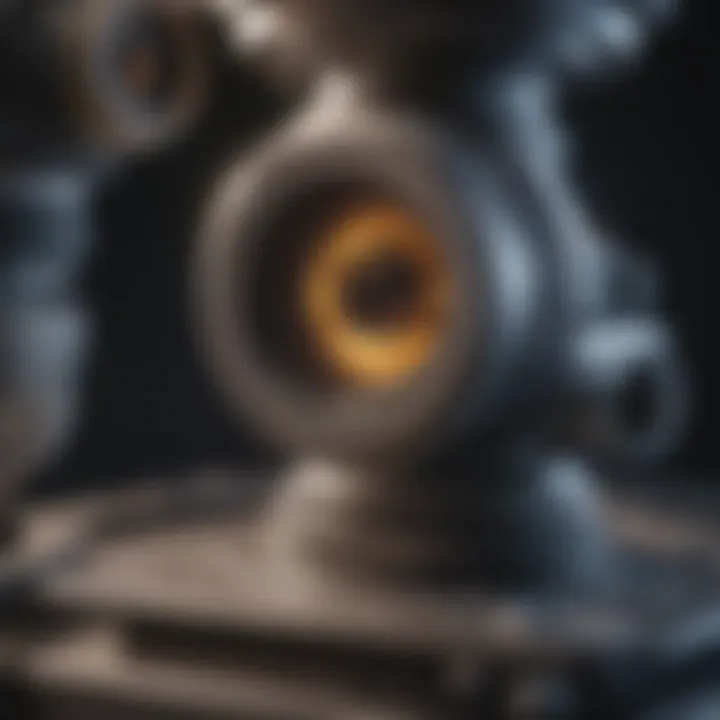
Transmission Electron Microscope (TEM)
The Transmission Electron Microscope, or TEM, is a pivotal instrument for researchers who need to observe the internal structures of specimens at an atomic level. TEM works by transmitting a beam of electrons through a thin sample. As electrons pass through, they scatter, creating an image that reveals intricate details about the sample's morphology and composition.
This modality is particularly beneficial in fields such as nanotechnology and materials science, where analyzing fine structures and defects is critical. The resolution of TEM can reach up to 0.1 nanometers, allowing researchers to visualize nano-scale features effectively.
Some important considerations for using TEM include the need for ultra-thin specimen preparation and the relatively complex operation compared to other electron microscopes. However, the depth of information gained justifies these requirements.
Scanning Electron Microscope (SEM)
The Scanning Electron Microscope, known as SEM, provides a different approach compared to TEM. SEM uses a focused beam of electrons that scans over the surface of a sample. When the electrons interact with the atoms of the sample, various signals are emitted, which are then collected to form a detailed three-dimensional image of the surface.
SEM is favored for its ability to image a wide range of materials with high depth of field, giving a more realistic view of specimen surfaces. The typical resolution of SEM is around 1 nanometer, making it suitable for studying larger structures, such as biological specimens and materials.
This type is simplified in comparison to TEM when it comes to specimen preparation, often allowing samples to retain their natural state and composition. These qualities make SEM an indispensable tool in many research areas, including biology, forensics, and materials science.
Scanning Transmission Electron Microscope (STEM)
The Scanning Transmission Electron Microscope, STEM, combines the principles of both TEM and SEM. It allows researchers to obtain both high-resolution images and quantitative data by scanning a sample with a finely focused beam of electrons while simultaneously transmitting them. This dual approach enables the examination of both surface features and internal compositions at an atomic level.
STEM is particularly suitable for applications where both surface and inner data are crucial, such as in nanomaterials research and advanced material characterization. The modality provides enhanced imaging resolution while enabling elemental analysis through techniques like atomic-resolution imaging and electron energy loss spectroscopy.
Challenges associated with STEM include the complexity of operation and the requirements for high-quality electron sources. These factors may limit its use in some laboratories, yet the comprehensive insights it provides are invaluable.
"Different types of electron microscopes serve distinct roles in advancing scientific research. Selecting the right one is vital for extracting the maximum information from materials and specimens."
"Different types of electron microscopes serve distinct roles in advancing scientific research. Selecting the right one is vital for extracting the maximum information from materials and specimens."
Understanding the nuances and applications of each type of electron microscope plays a critical role in advancing research across various disciplines. Their capabilities empower scientists to push the boundaries of discovery and innovation, enabling detailed investigations that were not possible with traditional optical techniques.
Advancements in New Electron Microscopes
The field of electron microscopy has witnessed remarkable advancements in recent years, fundamentally altering how researchers engage with and interpret the microcosm. These innovations have expanded the boundaries of imaging capabilities, providing unprecedented power for both biological and material science studies. The importance of these advancements can be summed up in several key elements:
- Increased Resolution: New electron microscopes are adept at providing high-resolution images that unveil intricate details previously hidden from view. This is crucial for biological research, where understanding molecular structures can lead to groundbreaking discoveries in health and disease.
- Enhanced Techniques: Techniques such as cryo-electron microscopy and 3D electron tomography have emerged. These allow scientists to observe samples in a near-native state, paving the way for authentic insights into dynamic processes.
- Broader Applicability: Innovations have made electron microscopy applicable across diverse fields. It is not just a tool for physicists and biologists; engineers and material scientists are now leveraging these technologies for various applications.
High-Resolution Imaging
High-resolution imaging is at the forefront of electron microscopy advancements. Modern electron microscopes can achieve resolutions down to the atomic scale. This level of detail enables scientists to explore the architecture of cells, proteins, and materials like never before. The increase in resolution stems from improved electron sources and advanced detector technology, which significantly enhances the signal-to-noise ratio in imaging.
Moreover, high-resolution imaging can reveal subtle changes in structure that correlate with function. In drug development, for instance, this ability to visualize a target at unprecedented resolution aids in rational drug design. Researchers can observe how drug candidates interact with biological macromolecules at a molecular level, ensuring greater efficacy.
Cryo-Electron Microscopy Techniques
Cryo-electron microscopy is a key innovation that revolutionizes how samples are prepared and observed. Unlike traditional methods that typically require heavy sample preparation, cryo-EM allows samples to be frozen quickly, preserving their native state. This technique is especially beneficial for studying complex biological structures such as viruses, proteins, and cellular components in their functional forms.
The implications of cryo-EM are vast. For instance, the recent resolution of virus structures has accelerated vaccine development. The unprecedented details obtained through cryo-EM provide vital insights into how viral proteins behave, informing both therapeutic strategies and vaccine design. As this technique continues to develop, its potential applications will likely expand even further.
3D Electron Tomography
3D electron tomography is another notable advancement. This technique offers the ability to reconstruct three-dimensional images from two-dimensional projections, enabling researchers to visualize intricate structures in three dimensions. It is particularly applicable in studying cellular organization, providing insights into spatial relationships within cells.
This advancement is not just a novelty; it can lead to substantive scientific breakthroughs. Consider the implications for nanotechnology, where understanding the 3D arrangement of nanomaterials is critical to improving their properties. By implementing 3D electron tomography, researchers can analyze how various nanostructures behave when subjected to different environments, refining and optimizing material properties for specific applications.
"The advancements in new electron microscope technologies are not merely incremental; they represent a seismic shift that redefines our understanding of the microscopic world."
"The advancements in new electron microscope technologies are not merely incremental; they represent a seismic shift that redefines our understanding of the microscopic world."
In summary, the advancements in new electron microscopes have elevated the potential for scientific discovery across disciplines. From the precision of high-resolution imaging to the authenticity brought by cryo-EM and the spatial insights from 3D tomography, these innovations are critical for the future of research.
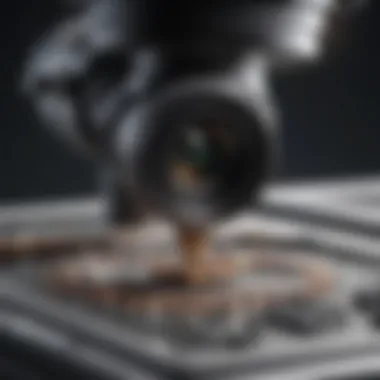
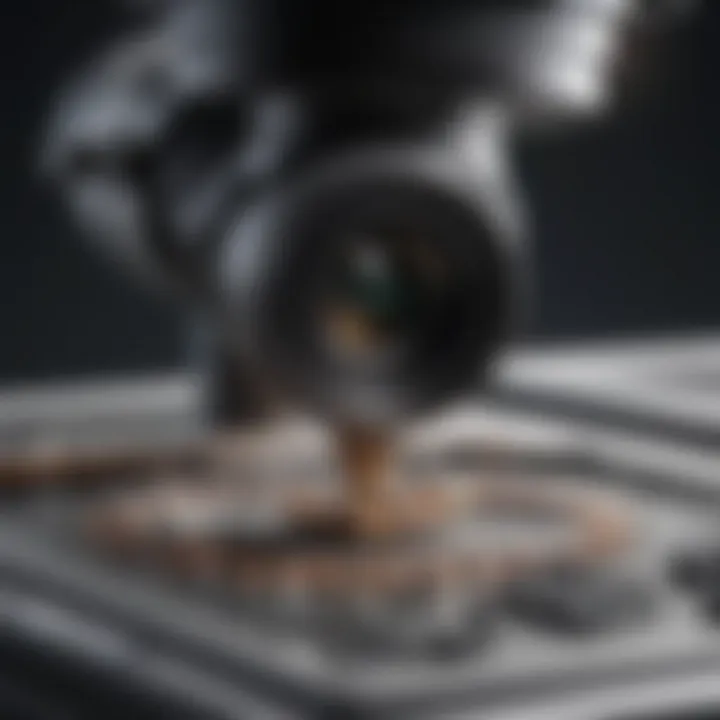
Applications in Biological Research
The field of biological research has been significantly enhanced by the innovations found in modern electron microscopy. This technology allows for unprecedented insight into biological structures at the nanometer scale. Understanding the intricate details of cellular architecture, molecular interactions, and dynamic processes is crucial for researchers. The applications of electron microscopy span across various sub-disciplines in biology, offering specific benefits that aid in advancing the scientific understanding of life.
Structural Biology
Structural biology is essential for elucidating the three-dimensional arrangements of biological macromolecules. Modern electron microscopy has made it possible to visualize complex structures like proteins and nucleic acids in their near-native states. With high-resolution imaging capabilities, researchers can observe the subtle conformational changes in biomolecules that are often critical for their function. Technologies such as cryo-electron microscopy allow scientists to study proteins in their hydrated form, minimizing artifacts caused by traditional sample preparation methods.
- In-depth Molecular Visualization: A focus on capturing fine details, such as atomic arrangements, provides valuable data for drug design and understanding disease mechanisms.
- Dynamic Studies: The ability to visualize dynamic processes in real-time offers insights into how macromolecules alter their structures in response to various stimuli.
This improved ability to analyze molecular structures at atomic resolution enhances our understanding of how proteins work, paving the way for discoveries in genetics and biochemistry.
Cellular Imaging
Cellular imaging plays a vital role in understanding cellular function and the organization of cellular components. Electron microscopy, particularly scanning electron microscopy, allows researchers to take detailed images of cell surfaces and interior structures. The high resolution enables a clearer observation of the spatial organization and interactions among organelles. This adds significant meaning to research on cell biology.
- Detailed Organellar Analysis: Investigating organelles such as mitochondria, lysosomes, and the endoplasmic reticulum help shed light on their roles in cellular health and disease.
- Understanding Cell Interactions: Visualizing how different cells interact with each other—or with their microenvironment—yields insights beneficial for developmental biology and immunology studies.
By bridging the gap between molecular and cellular levels, electron microscopy offers a comprehensive perspective that is invaluable for biological research.
Macromolecular Complexes Analysis
The analysis of macromolecular complexes is crucial for understanding multi-protein interactions and their functional consequences within cells. Electron microscopy provides tools to visualize these complexes in their near-native context, which is difficult to achieve using traditional techniques like X-ray crystallography.
"Understanding the architecture of macromolecular assemblies is fundamental for drug discovery and bioengineering."
"Understanding the architecture of macromolecular assemblies is fundamental for drug discovery and bioengineering."
- Elucidating Complex Structures: Electron microscopy facilitates the study of large and heterogeneous complexes, such as ribosomes and viral assemblies, which can hold the key to understanding viral infections or protein synthesis.
- Reconstruction Techniques: Advanced imaging techniques like 3D electron tomography enable researchers to reconstruct the three-dimensional architecture of these complexes, offering insights into their functional mechanisms.
As these analyses continue to evolve, they promise remarkable advancements in fields like structural biology, pharmacology, and virology, highlighting the power of improved electron microscopy techniques in biological research.
Applications in Material Science
The exploration of material science is greatly enhanced through the innovations brought by new electron microscopy techniques. Material science fundamentally relies on understanding the composition and structure of materials. The new electron microscopes offer unprecedented insights into the micro and nanostructures of various materials, which can lead to significant advancements in this field.
Utilizing high-resolution imaging provided by advanced electron microscopy allows researchers to visualize materials at the atomic level. This capability is essential for several applications, including nanomaterials investigation, defect analysis, and characterization of interfaces. As such, these techniques provide critical information on material properties that are invaluable in both research and practical applications.
Nanomaterials Investigation
Nanomaterials represent a crucial area in material science due to their unique properties that differ from their bulk counterparts. With the ability to manipulate matter at the nanoscale, researchers can design materials for specific applications, such as drug delivery, catalysis, and electronics. New electron microscopes allow for high-resolution analysis of these nanomaterials, enabling scientists to observe their structure and behavior directly. For instance, using tools like Scanning Electron Microscopy (SEM) and Transmission Electron Microscopy (TEM), one can obtain detailed images of nanomaterials, examining their size, shape, and distribution. This information is crucial for understanding how nanomaterials interact at the molecular level and how their structural characteristics influence their overall performance.
Defect Analysis in Materials
Understanding defects within materials is fundamental for predicting their performance and reliability. Defects can include vacancies, interstitials, and dislocations, which can dramatically affect the physical properties of materials. New electron microscopy techniques provide sophisticated ways to detect and analyze these defects. By employing methods like atomic resolution imaging, researchers can observe defects at an unprecedented scale. This analysis can lead to improved material properties and guide the design of materials with optimal strength and durability, particularly in fields such as aerospace and automotive engineering where material performance is critical.
Characterization of Interfaces
The interfaces between different materials play a significant role in determining the properties of composite materials and multilayer structures. Innovative electron microscopy enables detailed characterization of these interfaces. By examining the interface at high resolution, researchers can understand how different phases interact, which is essential in material engineering. For example, in semiconductor manufacturing, the interface between silicon and other materials carries immense importance for the functionality of electronic devices. Through these advanced imaging techniques, essential information about interface roughness, thickness, and composition can be gleaned, leading to improvements in material design and overall device performance.
"The impact of advanced electron microscopy techniques on material science cannot be overstated; they open new doors for scientific discovery and innovation."
"The impact of advanced electron microscopy techniques on material science cannot be overstated; they open new doors for scientific discovery and innovation."
Overall, applications in material science underscore the potential of new electron microscopes to drive advancements in both fundamental research and technology development. As the demand for better materials continues to grow, the role of electron microscopy becomes increasingly critical in understanding and innovating within this space.
Challenges and Limitations
The field of electron microscopy, while rich in potential and technical achievements, does not come without its own set of challenges and limitations. These obstacles can influence the accuracy, reliability, and applicability of the imaging techniques employed. Understanding these issues is essential for researchers who wish to utilize this sophisticated technology effectively. By recognizing the constraints, scientists can develop strategies to mitigate their impact and optimize their research outcomes.
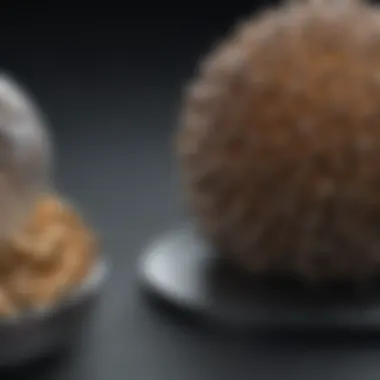
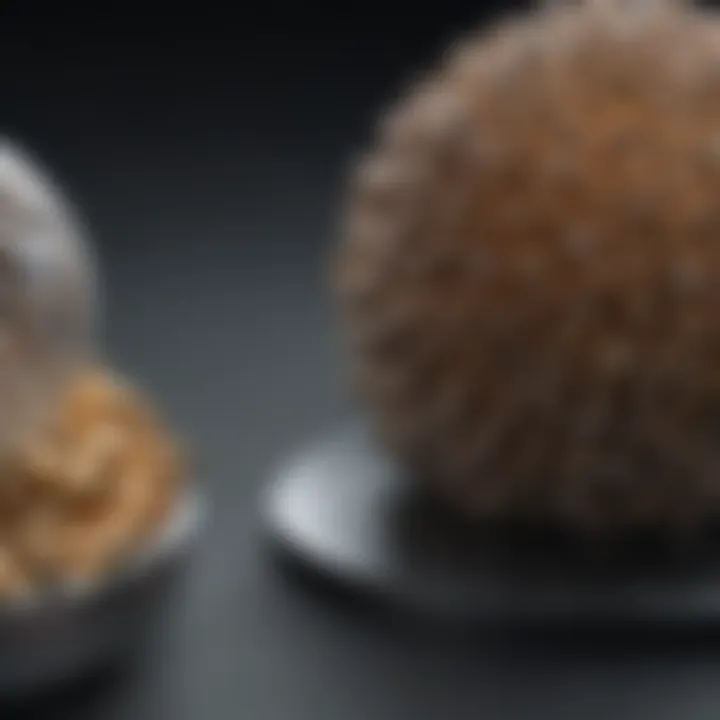
Sample Preparation Constraints
One of the most significant challenges in electron microscopy is the process of sample preparation. The materials that researchers wish to examine often need extensive preparation, which can sometimes alter their natural state. Sample preparation can involve a range of processes, such as fixation, dehydration, embedding, and sectioning, each of which requires careful execution. If improperly conducted, these steps can introduce artifacts that misrepresent the true structure of the specimen.
For example, biological samples may require quick freezing using cryo-embedding methods to preserve the cellular structure. However, this technique can be technically demanding. Researchers must ensure that samples are cooled rapidly and uniformly to avoid ice crystal formation that can distort the morphology of the sample.
Moreover, certain materials may be challenging to section into the thin slices needed for imaging. Hard or brittle materials can fracture, leading to uneven surfaces that complicate analysis. Consequently, researchers must possess strong expertise in various techniques to optimize sample preparation. Failure to do so not only affects the quality of the imaging but also limits the types of samples that can be analyzed effectively.
Interpretation of Complex Data
After successfully acquiring images, researchers face the next hurdle: the interpretation of the complex data. Electron microscopy generates an enormous amount of information, which can be overwhelming. The images produced often have intricate structures that require specialized knowledge to analyze accurately.
Interpreting data from techniques like three-dimensional electron tomography necessitates a deep understanding of both the imaging process and the biological or material context of the sample. Researchers must consider various factors, including noise from the imaging process, variations in contrast, and the potential for overlapping structures. Furthermore, the need for advanced computational skills adds another level of complexity. Many modern electron microscopy techniques use software that applies algorithms to process images. Still, the results depend heavily on the algorithms' design and parameters. Misinterpretations can occur if researchers are unfamiliar with the software or the theoretical principles behind the data processing.
In summary, while electron microscopy provides unparalleled insights into the microcosm, the challenges posed by sample preparation and data interpretation must be navigated carefully. Addressing these issues is vital for ensuring the accuracy and reliability of research findings in both biological and material sciences.
"Success in electron microscopy relies not only on cutting-edge technology but also on the ability to adapt to its inherent challenges."
"Success in electron microscopy relies not only on cutting-edge technology but also on the ability to adapt to its inherent challenges."
By recognizing these limitations, researchers can enhance their methodologies and contribute to the evolving field of electron microscopy.
Future Directions in Electron Microscopy Research
The realm of electron microscopy is at a pivotal juncture. Innovations are not only refining existing methodologies but are also paving the way for future explorations. The integration of advanced technologies holds the potential to revolutionize how scientists approach microscopy. As researchers seek to uncover the intricacies of materials and biological entities, certain directions emerge as particularly promising.
Integrating Artificial Intelligence
Artificial intelligence (AI) is increasingly influential in many fields, and electron microscopy is no exception. The incorporation of AI into electron microscopy enhances data analysis efficiency and precision. Machine learning algorithms can identify patterns in imaging data that human analysts may miss. For example, segmentation of complex images becomes streamlined, allowing researchers to focus on interpretation rather than labor-intensive processing.
Moreover, AI breeds greater predictive capabilities. By training models on existing datasets, researchers can generate hypotheses regarding new materials or biological pathways before experimental confirmation. This not only accelerates research timelines but also promotes a more hypothesis-driven exploration of significant scientific questions.
AI tools can also optimize imaging procedures through real-time feedback loops that adjust settings for optimal resolution and contrast. Such automated adjustments reduce the margin of error associated with manual setups, enhancing reproducibility in experiments. As AI technologies evolve, their integration with electron microscopy is expected to become more sophisticated, opening up new avenues for research.
The potential of AI in electron microscopy not only augments current methodologies but also ushers in a new era of automated scientific discovery.
The potential of AI in electron microscopy not only augments current methodologies but also ushers in a new era of automated scientific discovery.
Developing New Imaging Techniques
The continuous quest for enhanced imaging resolution and depth has sparked the innovation of new imaging techniques in electron microscopy. Researchers are focused on developing methods that can capture dynamic biological processes in real-time. For instance, advances in time-resolved electron microscopy allow for the observation of interactions at the atomic level as they occur. This capability is especially significant for studying bio-molecular interactions, which are critical for understanding cellular behaviors.
Furthermore, new techniques aim to improve imaging quality while minimizing sample damage. Novel methodologies, such as ultra-fast electron pulses, seek to acquire high-resolution images without the drawback of radiation damage to sensitive biological specimens. This balance between detail and integrity is essential, particularly when the target of observation is fragile, such as proteins or living cells.
As developments in materials science progress, innovations in electron microscopy also respond to the need for examining increasingly complex structures. Techniques like phase plates or in situ microscopy are gaining traction for their ability to analyze materials in their natural environment, providing insights into stress and strain at the nanoscale.
In summary, the future directions of electron microscopy research present exciting opportunities. The integration of AI and the advent of new imaging techniques signal a transformative phase that enhances our ability to visualize and understand the microscopic world.
Finale
The conclusion serves as a vital encapsulation of the discussions presented throughout the article. It synthesizes the insights gained about new electron microscopy technologies and reinforces their importance in advancing scientific research. Specifically, the concluding section highlights how innovations such as cryo-electron microscopy and scanning transmission electron microscopy have expanded our capacity to visualize and analyze samples at unprecedented resolution. This is not merely a technological evolution; it represents a significant leap in our ability to understand complex biological and material systems.
Summary of Key Points
The key points elucidated in this article include the following aspects:
- Historical Context: Electron microscopy has a storied past that laid the foundation for its current capabilities.
- Types of Electron Microscopes: There are three main types: Transmission Electron Microscope (TEM), Scanning Electron Microscope (SEM), and Scanning Transmission Electron Microscope (STEM), each with unique applications.
- Advancements in Technology: Key advancements encompass high-resolution imaging, cryo-electron microscopy techniques, and 3D electron tomography, which provide deeper insights into structures and dynamics.
- Applications Across Fields: Biological research applications involve structural biology and cellular imaging, while material science uses include nanomaterials investigation and defect analysis.
- Challenges and Limitations: Significant challenges remain, such as sample preparation constraints and the complex interpretation of data.
- Future Directions: Integrating artificial intelligence and developing new imaging techniques are paramount to further enhancing electron microscopy's capabilities.
The Broader Impact of Enhanced Electron Microscopy
Enhanced electron microscopy is reshaping various scientific disciplines. It fosters greater understanding of biological processes at the molecular level, informing fields such as medicine and genetics. In materials science, the ability to analyze nanomaterials and interface characteristics drives innovation in engineering and technology.
"The advancements in electron microscopy have not only improved imaging quality but have also influenced the way researchers approach scientific questions."
"The advancements in electron microscopy have not only improved imaging quality but have also influenced the way researchers approach scientific questions."
Such developments can potentially accelerate discoveries in other areas of research. The intricate link between the innovations of electron microscopy and their wider implications for technology, health, and knowledge is a crucial takeaway. As the field evolves, the impact of these improvements will extend well beyond the laboratory, providing significant contributions to our understanding of the natural world.