Mass Spectrometry: Key to Accurate Quantitative Analysis
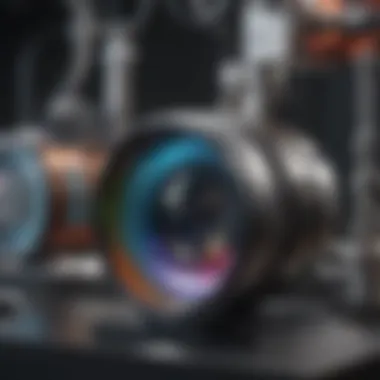
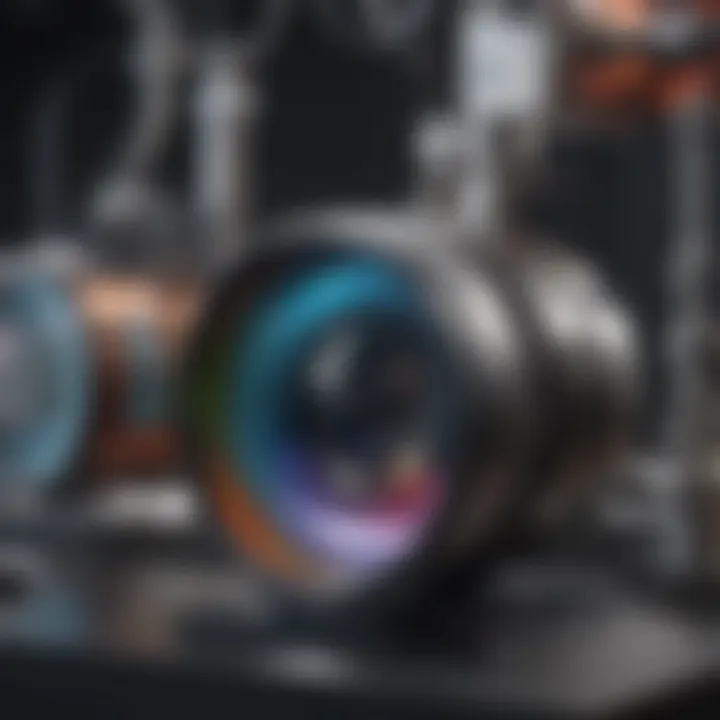
Article Overview
Mass spectrometry has taken center stage in the field of quantitative analysis, and for a good reason. This technique is often regarded as a game changer across various scientific disciplines. It allows researchers and professionals to determine the composition and structure of substances with amazing precision.
Purpose of the Article
This article aims to explore the intricate details of mass spectrometry, providing a comprehensive understanding of its principles and applications. By dissecting the methods of sample preparation, calibration, and data interpretation, the reader will grasp the depth and breadth of mass spectrometry in quantitative analysis. Furthermore, the article sheds light on recent advancements and associated challenges, ensuring that the information conveyed is timely and relevant.
Relevance to Multiple Disciplines
The significance of mass spectrometry transcends academic boundaries, branching into fields such as:
- Biomedical research
- Environmental science
- Pharmaceuticals
- Food safety analysis
Its versatility empowers scientists to tackle some of the most pressing issues in each of these areas. For instance, in biomedical research, mass spectrometry can accurately quantify biomarkers in complex biological samples, which is crucial for early disease detection.
Research Background
Understanding the roots and evolution of mass spectrometry helps clarify its current applications and importance.
Historical Context
The journey of mass spectrometry began in the early 20th century. It began as a novel curiosity within the realm of physical chemistry. Over the decades, it developed rapidly, driven by advancements in technology and techniques. Today, mass spectrometry serves pivotal roles in both qualitative and quantitative analyses across a multitude of scientific fields.
Key Concepts and Definitions
It’s essential to grasp certain foundational concepts before diving deeper into mass spectrometry:
- Ionization: The process of converting molecules into ions, allowing them to be analyzed.
- Mass-to-charge ratio (m/z): A critical measurement that informs the mass of ions.
- Calibration: Essential for ensuring accuracy, this involves comparing the results of the measurement to a standard.
- Data Interpretation: Techniques used to make sense of the output from mass spectrometry, yielding valuable insights into the sample’s composition.
Mass spectrometry itself can often be likened to a sophisticated dance between molecules and technology—a symphony of scientific rigor that harmonizes precision, efficiency, and adaptability.
Prelude to Mass Spectrometry
Mass spectrometry represents an essential facet of analytical science, renowned for its precision and versatility in quantitative analysis. Understanding its fundamental principles not only equips readers with insights into its functioning but also underscores its application in real-world scenarios. In an age where data accuracy is paramount, delving into mass spectrometry provides significant benefits such as effective identification and quantification of compounds in diverse matrices. Moreover, the ability to measure molecular weights with high sensitivity places mass spectrometry at the forefront of cutting-edge research and industrial applications.
Definition and Basic Principles
At its core, mass spectrometry is a technique that measures the mass-to-charge ratio of ions. It provides a way to analyze the composition of a sample, be it biological, environmental, or industrial, by converting compounds into ions and directing them through an electric or magnetic field. In doing so, it allows researchers to discern the qualitative and quantitative properties of those compounds down to the femtomole level. This capability is vital for various applications, ranging from drug development to forensic analysis.
The process typically unfolds in three central stages:
- Ionization: Where the sample is converted into ions, either by means of methods like Electrospray Ionization or Matrix-Assisted Laser Desorption/Ionization.
- Mass Analysis: Following ionization, the ions are sorted based on their mass-to-charge ratios in a mass analyzer, such as the Quadrupole or Time-of-Flight.
- Detection: The sorted ions are then detected, and a spectrum is generated. This spectrum displays the intensity of the ion signals against their respective charge-to-mass ratios, providing a visual representation of the analytes present.
By utilizing these principles, mass spectrometry has proven to be an instrumental tool, especially in fields where quantitative analysis is critical.
Historical Context
The journey of mass spectrometry began in the early 20th century, highlighted by contributions from pioneers such as J.J. Thomson, who first identified isotopes in 1912. Subsequent advancements throughout the decades set the stage for the mass spectrometer's evolution into a robust analytical tool. By the 1950s and 1960s, the emergence of vacuum technology and electronic components revolutionized the industry—ushering in innovations like the first commercial mass spectrometer.
As the years rolled on, developments such as the introduction of ion traps and improved ionization techniques significantly enhanced mass spectrometry's capabilities. The late 20th century marked an era of unprecedented growth, where mass spectrometry began to establish its footing in diverse scientific arenas. Its application burgeoned in sectors including pharmaceuticals, environmental monitoring, and biomedical research. Today, mass spectrometry is not merely a laboratory tool; it’s an integral component of global scientific progress.
The Importance of Quantitative Analysis
Quantitative analysis is a cornerstone of scientific inquiry, providing an objective framework for measuring and interpreting data across diverse fields. In the context of mass spectrometry, the emphasis on quantitation ensures reliable results that propel both research and practical applications. Achieving accuracy and precision in measurements is not merely a procedural step; it's integral to validating results and fostering advancements in various disciplines.
Significance in Scientific Research
In scientific research, the ability to quantify compounds accurately has profound implications. This significance starts with the foundation of hypothesis testing. Researchers can determine whether their experimental variables have statistically meaningful effects. Without quantification, conclusions would be akin to navigating a ship without a compass—directionless and susceptible to error.
Quantitative analysis through mass spectrometry enables detection of compounds at trace levels, which can be crucial in fields such as toxicology and pharmacology. For example, consider the work done in identifying metabolites of drugs in biological samples. A laboratory successfully pinpointed the presence of a particular drug's metabolites in urine.
- Validation: By quantifying the concentrations of these metabolites, researchers validated their hypotheses concerning drug metabolism and effectiveness.
- Replication: Other research teams could then replicate the study’s findings, lending credence to the initial discovery and stimulating further inquiry.
- Policy Influence: Such analyses may influence clinical guidelines or regulatory policies, illustrating the far-reaching consequences of accurate quantitative results.
Applications Across Disciplines
The applications of mass spectrometry in quantitative analysis extend beyond the laboratory, touching multiple sectors and shaping our environment.
- Clinical Diagnostics: In healthcare, precise quantification of biomolecules can aid in diagnosing diseases early. Think of cancer markers in blood samples. Their levels can guide treatment decisions.
- Environmental Science: With the rising concerns about pollution, mass spectrometry is employed to monitor contaminants in air and water. For instance, scientists can measure the concentration of pesticides in soil samples, guiding agricultural practices and environmental policies.
- Pharmaceutical Development: When developing new drugs, understanding the pharmacokinetics of a compound—how it’s absorbed, distributed, metabolized, and excreted—is vital. Through quantitative analysis, researchers can determine dosage regimens that maximize therapeutic benefits while minimizing adverse effects.
Each application shares a common thread; they hinge on the ability to accurately measure and interpret quantitative data, ultimately leading to informed decision-making. Understanding these connections enriches the discussion surrounding mass spectrometry and motivates ongoing innovation.
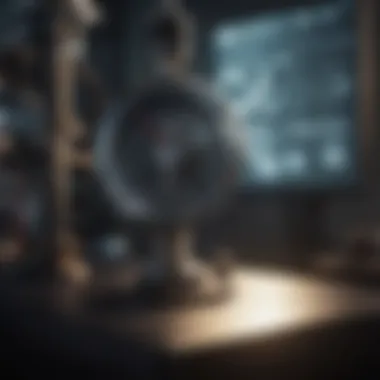
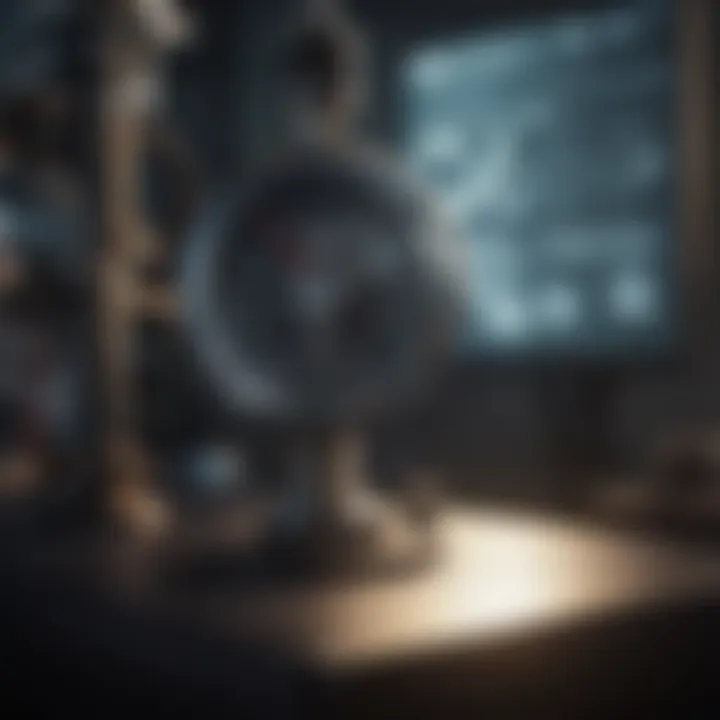
"Quantitative data provides the backbone for empirical science, turning conjectures into knowledge."
"Quantitative data provides the backbone for empirical science, turning conjectures into knowledge."
In summary, the importance of quantitative analysis cannot be overstated. It is the bedrock upon which scientific truth is built, particularly in mass spectrometry. This technique does not merely analyze samples; it transforms them into a wealth of information that drives knowledge and progress.
Fundamentals of Mass Spectrometry Technique
The fundamentals of mass spectrometry (MS) technique serve as the bedrock for its application in quantitative analysis. Understanding these principles not only enhances the reliability of results but also facilitates informed choices in selecting the right methodology for specific analytical needs. If one grasps these foundational elements, it's easier to navigate the complexities of mass spectrometry and appreciate its various applications across disciplines.
Ionization Methods
Electrospray Ionization
Electrospray Ionization (ESI) stands out in mass spectrometry due to its ability to ionize large biomolecules without significant fragmentation. It’s particularly popular in the realm of proteomics and metabolomics. The main characteristic of ESI is its soft ionization technique, which gently handles samples, preserving their integrity for accurate analysis. One unique feature is its capacity to produce multiple charged ions, making it suitable for analyzing biomolecules like proteins and nucleic acids that can be cumbersome to study otherwise. The benefits of using ESI include its high sensitivity and compatibility with liquid chromatography, but it also have some downsides, such as being less effective for non-polar compounds.
MALDI
Matrix-Assisted Laser Desorption/Ionization, or MALDI, has carved a niche for itself in analyzing large, complex biomolecules. The method involves embedding the sample in a matrix material, which absorbs laser energy, resulting in the desorption and ionization of the analyte. A key characteristic that makes MALDI attractive is its capability to handle a wide mass range, making it suitable for various types of samples – from peptides to polymers. The unique feature of MALDI lies in its speed and simplicity, allowing rapid analysis of samples. However, like any technique, it isn’t without flaws; issues can arise with signal suppression and varying ionization efficiency across different compounds.
Electron Ionization
Electron ionization (EI) is favored in the analysis of small volatile compounds, often found in organic chemistry. By bombarding the sample with electrons, it results in the formation of positive ions, allowing for detailed structural elucidation. The defining trait of EI is its ability to provide rich fragmentation patterns which can be invaluable for understanding molecular structures. What's appealing about EI is its well-established methodology and its compatibility with gas chromatography. However, it does have its shortcomings, including the requirement for solid samples and the potential for excessive fragmentation that might obscure the target analysis.
Mass Analyzers
Quadrupole
The quadrupole mass analyzer is renowned for its robustness and ease of use, making it one of the most popular choices in mass spectrometry. The key characteristic here is its ability to filter ions based on their mass-to-charge ratio, allowing for precise data acquisition. A unique feature of the quadrupole is its relatively fast scanning speed, enabling quick analysis of multiple ions. However, while the quadrupole is versatile and reliable, it also has limitations in resolution compared to other technologies like TOF.
TOF
Time-of-Flight (TOF) analyzers are distinguished by their high resolution and mass accuracy, making them excellent for analyzing complex mixtures. The main characteristic is the measurement of the time ions take to travel a fixed distance; heavier ions take longer than lighter ones. This unique feature leads to quick acquisition and the ability to analyze large molecules effectively. TOF analyzers excel in applications ranging from proteomics to environmental samples. On the downside, they can be more expensive and require meticulous calibration.
Ion Trap
Ion trap mass analyzers have developed a reputation for their ability to store ions and analyze them over time. One key characteristic is the ability to re-activate stored ions, allowing multiple analyses from a single sample. This makes it a go-to for detailed structural analysis. A particular advantage of ion traps is their extreme sensitivity, ideal for detecting trace analytes. However, a downside is that they may struggle with high throughput applications when compared to other types.
Data Acquisition Techniques
Understanding data acquisition techniques is pivotal in mass spectrometry, as it plays a crucial role in determining the accuracy and reliability of the data collected. Modern advancements have introduced several sophisticated methods that enhance data clarity and precision, making it essential to choose the right acquisition strategy that aligns with the specific analytical needs.
Sample Preparation for Mass Spectrometry
Sample preparation is a crucial step in the mass spectrometry process and often sets the tone for the success of the analysis. Properly prepared samples ensure that the subsequent data collected is reliable and representative of the target analytes. Without effective sample preparation, one risks introducing noise or contaminants that could obscure results. This section will highlight various elements and considerations regarding sample preparation that contribute to optimizing mass spectrometry outcomes.
Types of Sample Matrices
Understanding the types of sample matrices is fundamental in mass spectrometry. A sample matrix can be described as the environment in which the analyte exists, encompassing everything from biological tissues, environmental samples to complex mixtures. Each matrix presents unique challenges and requires tailored approaches during preparation. Common types include:
- Biological Matrices: These often include blood, urine, tissues, or cells. They may contain proteins, lipids, and various metabolites that can interfere with analysis.
- Environmental Matrices: Soil, air, and water samples fall under this category, where contaminants and diverse chemical compositions influence the analytical process.
- Food Matrices: This matrix includes anything from vegetables to dairy products, having diverse components that might affect mass spectrometry outcomes.
Choosing the right method of extraction or clean-up depends largely on the matrix type. It’s not merely about isolating the analyte; it also involves minimizing other components that could lead to misleading results.
Extraction and Clean-Up Procedures
Extraction and clean-up are integral in the journey from sample collection to data interpretation in mass spectrometry. These procedures not only isolate the analyte of interest but also enhance its detectability.
Solid-Phase Extraction
Solid-phase extraction (SPE) has become a cornerstone in preparing samples for mass spectrometry. The primary advantage of SPE is its capability to selectively isolate an analyte from a complex mixture while simultaneously reducing matrix interferences. This method employs solid sorbents to trap analytes while allowing undesired components to pass through.
One key characteristic of SPE is its versatility. Whether the sample is aqueous or organic, different sorbent materials can be chosen based on the analyte's properties. The unique feature of SPE lies in its ability to provide high recovery rates with minimal contamination.
Despite its advantages, SPE isn't without its complications. Technique optimization is essential, and overextraction can potentially lead to analyte loss or degradation, which can be detrimental, especially in quantitative analysis.
Liquid-Liquid Extraction
Liquid-liquid extraction (LLE) is another widely used technique in the preparatory phase of mass spectrometry. This method exploits the different solubility properties of compounds in two immiscible liquids, typically an organic solvent and an aqueous phase. The process involves transferring the target analyte from one liquid phase to another, resulting in concentration of the analyte in a smaller volume of solvent.
A key highlight of LLE is its simplicity and the ability to process larger sample volumes compared to SPE. However, while it can be effective for certain analytes, it’s also worth noting that selectivity may be an issue. With multiple components in a sample, unwanted substances can co-extract, leading to matrix effects during analysis.
"Sample preparation is more than just a step; it's an art that requires understanding and precision to unveil the truth in complex matrices."
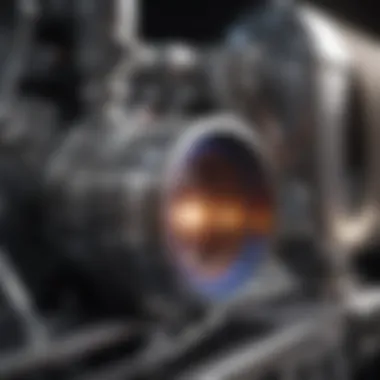
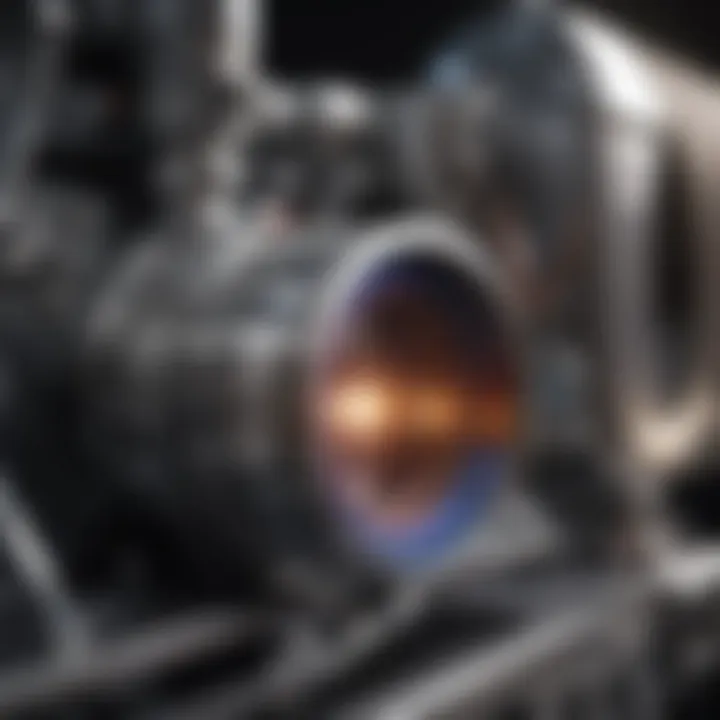
"Sample preparation is more than just a step; it's an art that requires understanding and precision to unveil the truth in complex matrices."
As mass spectrometry continues to advance, so too do the methodologies surrounding sample preparation, with ongoing research aimed at improving efficiency and efficacy in this foundational aspect of quantitative analysis.
Calibration Techniques in Quantitative Analysis
Calibration techniques are paramount in mass spectrometry when it comes to quantitative analysis. Their role cannot be overstated, as they establish the foundation for accurate and reliable measurements. In this section, we will delve into the significance of calibration, breaking it down into two primary methodologies: internal and external standardization, as well as a thorough examination of linearity and range assessment.
Internal and External Standardization
Calibration in mass spectrometry focuses on quantifying analytes within unknown samples. Two key methodologies are employed: internal and external standardization.
- Internal Standardization involves adding a known quantity of a standard substance to the sample prior to analysis. The advantage here is that variations in sample preparation and instrument performance are minimized. For instance, if you’re testing the concentration of a drug in a biological sample, you might include a stable isotopic internal standard. This ensures that any fluctuations during the analytical procedure affect both the internal standard and the analyte similarly, thus allowing for more precise quantification.
- External Standardization, on the other hand, requires running a series of known concentration standards alongside the unknown samples. This method relies heavily on the construction of a calibration curve. A common practice is to prepare at least five concentrations of the target analyte. Each sample is analyzed with mass spectrometry, and the response (such as peak area or height) is plotted against concentration. Once established, the concentrations of unknowns can be derived from this curve. While simple in concept, it can be sensitive to instrument drift and variability, which necessitates rigorous quality control.
Both methodologies possess their own sets of pros and cons. Understanding these is crucial for selecting the appropriate technique for your specific analysis needs, especially in complex biological or environmental samples.
Linearity and Range Assessment
A defining attribute of calibration techniques is the assessment of linearity and range. Linearity refers to how well the response of the mass spectrometer correlates with the concentration of an analyte over a specified range. This is vital because an accurate linear relationship ensures consistent and predictable measurements.
To evaluate linearity, one must perform a regression analysis using the calibration curve obtained from the external standardization method. Here’s a stepwise approach:
- Prepare Calibration Standards: Create a range of solutions with known concentrations.
- Analyze Standards Using Mass Spectrometry: Record the mass spectral response for each standard solution.
- Generate a Calibration Curve: Plot the concentration of the standards against their respective responses and fit a linear regression line.
- Calculate the Coefficient of Determination (R²): An R² value close to 1 indicates strong linearity, validating that the mass spectrometry response reflects changes in analyte concentration accurately.
Furthermore, range assessment highlights the span of concentrations where the technique remains reliable. It is essential to define both the lower limit of quantification (LLOQ) and the upper limit of quantification (ULOQ). These determinations ensure that results obtained from the mass spectrometer are both accurately quantifiable and provide a clear picture of your analyte concentration, thereby preventing false conclusions in research.
Data Interpretation in Mass Spectrometry
Data interpretation is pivotal in mass spectrometry, transforming raw data from the mass spectrometer into meaningful results. This process is akin to turning a jumble of puzzle pieces into a coherent image, revealing the identity, quantity, and structure of chemical compounds within a sample.
Understanding Mass Spectra
Mass spectra are graphical representations of a compound's mass-to-charge ratio. Each peak corresponds to a different ion, providing critical information about the molecules present in the sample.
- Peaks and Intensity: The height of each peak indicates the relative abundance of the corresponding ion. A taller peak suggests a higher concentration of that ion in the sample, while shorter peaks suggest lesser quantities. Understanding these peaks is fundamental for quantification.
- Isotope Patterns: Mass spectra may also show isotopes of elements which can indicate subtle differences in molecular makeup. This is particularly important in areas like environmental chemistry, where distinguishing between isotopes can result in insights about sources of pollution.
- Fragmentation Patterns: As ions can fragment, the resulting pattern aids in the elucidation of molecular structures. Identifying these fragments not only helps in confirming the identity of compounds but also provides insight into their chemical behavior.
Data interpretation goes beyond merely reading spectra; it requires an understanding of chemistry and technology, making it a skill honed over time through practice and experience.
Quantitative Data Analysis
Quantitative data analysis employs various methods to ensure that the data obtained from mass spectrometry is reliable and accurate. This involves both hardware and software tools, and statistical techniques play a significant role here.
Software Tools
Software tools are crucial for processing mass spectrometry data, helping researchers to analyze complex datasets effectively. One notable tool is MassLynx, widely used for its user-friendly interface and robust algorithms for data evaluation.
- Key characteristic: MassLynx offers integrated data acquisition and analysis features, enabling a smooth workflow from sample injection to results interpretation.
- Unique feature: It provides a comprehensive library of standard and custom methods tailored to varied analytical needs.
- Advantages: The software excels in automating the calculation of concentration from calibration curves, significantly reducing human error. However, the cost of advanced licensing can be a barrier for smaller labs.
Statistical Approaches
Statistical approaches underpin the reliability of quantitative mass spectrometry. Techniques such as regression analysis and ANOVA are frequently utilized to validate results.
- Key characteristic: Regression analysis helps in determining the relationship between the concentration of analytes and their corresponding signals. This statistical tool is foundational for calibration curves.
- Unique feature: Software options often include statistical add-ons that allow real-time data modeling, making data validation more straightforward.
- Advantages: It enhances the credibility of data results, crucial in fields like pharmaceuticals, where even minor discrepancies can lead to regulatory complications. Conversely, a limitation can be the requirement for a solid understanding of statistical principles, which may be a hurdle for some researchers.
Challenges in Qualitative and Quantitative Analysis
The field of mass spectrometry, while offering a powerful avenue for analysis, is not without its hurdles. Understanding these challenges can provide insights into the complexities faced by researchers and practitioners alike. When engaging in both qualitative and quantitative analyses, factors such as instrument calibration and matrix effects can have profound ramifications on analytical outcomes. Addressing these issues is crucial, as the reliability and accuracy of mass spectrometric data depend heavily on overcoming them.
Instrument Calibration Issues
Calibration serves as the backbone of any quantitative analysis. The process ensures that the mass spectrometer delivers accurate readings for the analytes of interest. However, inherent challenges arise during this stage. One notable issue is instrumental drift, where the response of the machine changes over time due to environmental conditions or wear and tear. This variation can lead to inconsistent results, which, in turn, affect data interpretation.
A common approach to tackle this problem is the implementation of regular calibration checks. For instance, utilizing a well-characterized standard during the analysis allows for recalibration against known values. Moreover, utilizing internal standards can further enhance the reliability.
"Without proper calibration, even the most sophisticated instruments can yield erroneous results that could skew research outcomes dramatically."
"Without proper calibration, even the most sophisticated instruments can yield erroneous results that could skew research outcomes dramatically."
Another challenge involves the selection of appropriate standards for calibration. Different analytes may respond differently to various conditions, necessitating careful consideration during the calibration setup. This complexity underscores the need for comprehensive understanding and meticulous planning in analytical workflows.
Matrix Effects
Matrix effects highlight another major challenge faced in mass spectrometry. These effects occur when components present in the sample other than the target analyte influence the ionization efficiency. For instance, a complex biological matrix can hinder the ionization of certain drugs, leading to under- or overestimation of their concentrations. The implications of matrix effects extend beyond just the measurements; they can undermine the overall reliability of the entire analytical process.
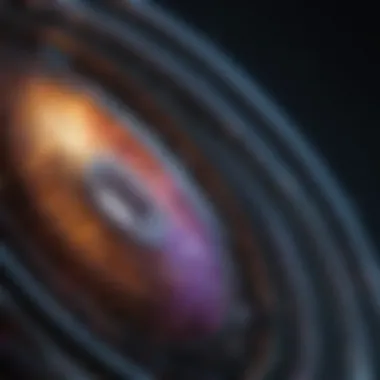
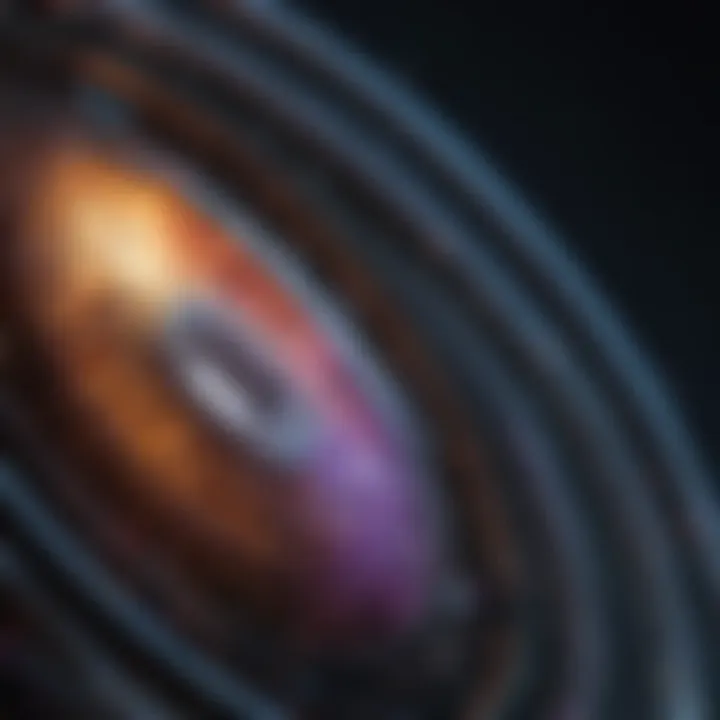
To mitigate matrix effects, researchers often employ various strategies such as:
- Sample Cleanup: Techniques like solid-phase extraction or liquid-liquid extraction can help isolate the analyte from the matrix components, thereby enhancing measurement accuracy.
- Dilution of Samples: Sometimes, simply diluting the sample can help minimize the interference caused by matrix components, allowing for clearer readings.
- Use of Standard Addition: This method involves adding known quantities of the analyte to the sample, which can help in adjusting for any suppression or enhancement of signal caused by other components.
Both instrument calibration issues and matrix effects emphasize the necessity of rigorous methodological rigor when conducting analyses in mass spectrometry. These challenges can be daunting, but awareness and understanding pave the way toward more robust and accurate quantitation.
Applications of Mass Spectrometry in Various Fields
Mass spectrometry plays a crucial role beyond mere academic intrigue; its applications stretch across varied and significant domains, influencing fields such as clinical research, environmental monitoring, and pharmaceutical development. Understanding these applications not only exemplifies the versatility of mass spectrometry but also highlights how vital it is for advancing both science and public health.
Clinical and Biomedical Research
In the realm of clinical and biomedical research, mass spectrometry has established itself as an invaluable tool. The ability to analyze biological samples with precision is paramount. For example, blood and urine samples can be subjected to targeted metabolomics, allowing researchers to identify biomarkers for diseases like cancer or diabetes. The advantage here lies in mass spectrometry's capacity for high sensitivity and specificity.
Moreover, this technique provides quantitative insights that help in the assessment of disease progression or response to treatment. Take, for instance, the detection of therapeutic drugs and their metabolites. By accurately measuring drug levels in a patient's body, clinicians can tailor dosages to individuals, optimizing therapeutic effects while minimizing side effects.
Just as importantly, the integration of mass spectrometry with liquid chromatography enhances resolution and sensitivity, providing a more detailed picture of the patient’s biochemical landscape. This dual approach allows clinicians to make informed decisions and devise better treatment plans.
Environmental Monitoring and Analysis
Another field that has significantly benefited from mass spectrometry is environmental science. The detection and analysis of pollutants, heavy metals, and other toxic substances in air, soil, and water are crucial for maintaining public health and ecological balance. Mass spectrometry enables analysts to identify trace levels of contaminants that might otherwise evade detection.
- Air Quality: With global environmental concerns on the rise, mass spectrometry aids in tracking volatile organic compounds (VOCs) that contribute to air pollution. The ability to detect low concentrations quickly is vital for enforcing environmental regulations.
- Water Safety: Similarly, in testing drinking water, mass spectrometry can identify harmful substances like pesticides and pharmaceuticals. Such monitoring is essential for ensuring safe drinking water and protecting aquatic ecosystems.
The precision and efficiency of mass spectrometry also foster rapid response strategies in environmental crises, enabling authorities to react promptly and mitigate harm.
Pharmaceutical Development
In the pharmaceutical sector, mass spectrometry is a cornerstone of drug development processes. The method streamlines the stages of discovery, formulation, and quality control. Researchers utilize it to conduct stability studies and identify degradation products, which can significantly influence formulation strategies.
Additionally, during the drug discovery phase, mass spectrometry enables high-throughput screening for potential drug candidates. The tool's capability to provide detailed structural information assists in understanding how new compounds interact at a molecular level. This accelerates the path from the laboratory to clinical application.
Furthermore, regulatory agencies require rigorous analytical profiles for new drugs. Mass spectrometry meets these requirements by providing comprehensive data on compound purity, potency, and concentration. Through consistent application of this technology, pharmaceutical companies can ensure compliance while enhancing the safety and efficacy of their products.
Mass spectrometry serves as an essential analytical underpinning across diverse scientific landscapes, shaping practices in medical, environmental and industrial settings alike.
Mass spectrometry serves as an essential analytical underpinning across diverse scientific landscapes, shaping practices in medical, environmental and industrial settings alike.
Future Trends in Mass Spectrometry
The landscape of mass spectrometry is rapidly evolving, and staying abreast of the emerging trends is essential for anyone involved in quantitative analysis. Innovations in technology and methodology are not just improving the effectiveness of mass spectrometry; they are also expanding the scope of its applications. With increasing demands from various sectors—from climate research to drug development—understanding these future trends allows researchers and professionals to adapt and optimize their approaches.
Technological Advancements
Recent years have seen significant technological advancements that are reshaping mass spectrometry. There’s no doubt that developments in hardware and software have made instruments more sensitive and capable of producing cleaner data.
For example, high-resolution mass spectrometry (HRMS) allows for the detection of lower concentrations of analytes, contributing to more reliable quantitative results. This technology significantly reduces the background noise in mass spectra, leading to clearer peaks and better interpretation. Likewise, advancements in ionization techniques, such as the integration of ambient ionization methods, have made it possible to analyze samples directly in their native state—all without extensive sample preparation.
In addition, automation and integration of artificial intelligence is becoming increasingly prevalent in the analytical workflow. Algorithms can now assist in identifying compounds and predicting their behavior. This reduced the time researchers spend on manual analysis and enhances the accuracy of results.
Integration with Other Analytical Techniques
The fusion of mass spectrometry with other analytical methods is unlocking new capabilities in various fields. By combining mass spectrometry with techniques, such as liquid chromatography (LC-MS), researchers can achieve improved separation of complex mixtures. The integration allows one to tackle intricate biological samples that would otherwise create a headache during analysis.
Moreover, coupling mass spectrometry with NMR or infrared spectroscopy is something people are looking at more these days. While mass spectrometry tells us about the mass-to-charge ratio of ions, NMR and IR can provide structural information about the molecules in question. In an era that demands more detailed analysis, these combinations empower researchers to deepen their understanding of molecular structures and interactions.
As the boundaries between disciplines continue to blur, cross-disciplinary approaches that utilize mass spectrometry alongside other techniques will only become more essential to solving complex scientific questions.
As the boundaries between disciplines continue to blur, cross-disciplinary approaches that utilize mass spectrometry alongside other techniques will only become more essential to solving complex scientific questions.
In summary, the future of mass spectrometry is bright and holds tremendous potential for growth. The advances occurring today will likely translate into more robust analytical capabilities and innovative applications that are pivotal for both research and industry. Staying aware of these developments not only enhances one’s research prospects but also sets the stage for future opportunities in various domains.
End
As we conclude our exploration of mass spectrometry in quantitative analysis, it's essential to highlight the pivotal role this technique plays across a wide range of scientific disciplines. Mass spectrometry is more than a complex analytical tool; it’s a bridge connecting theoretical research and practical application. Understanding how to leverage its capabilities is vital for enhancing data accuracy, which translates into better decision-making in areas like healthcare, pharmacology, and environmental science.
Summary of Key Points
In summary, the key elements discussed in the article underscore several vital facets of mass spectrometry in quantitative analysis:
- Definition and Principles: We delved into the fundamental principles of mass spectrometry, establishing a background for understanding its significance.
- Importance in Various Fields: Applications ranging from clinical research to environmental monitoring showcase the versatility of this methodology.
- Techniques and Calibration: The importance of proper sample preparation, selected ionization techniques, and effective calibration methods cannot be overstated. They are crucial for ensuring the reliability of quantitative data.
- Challenges: Instrument calibration issues and matrix effects were discussed, emphasizing the need for constant vigilance and adaptation in analytical practices.
- Future Trends: Technological advancements and the integration with other analytical techniques signal a future where mass spectrometry becomes even more powerful and precise.
These points illustrate how deeply interconnected the practice of quantitative analysis is with mass spectrometry. Each section of the study enhances our appreciation of the method's rigor and adaptability.
The Ongoing Relevance of Mass Spectrometry
Mass spectrometry continues to be a relevant and growing domain in analytical science, adapting to the ever-changing landscape of research demands. The ongoing evolution of technology and methodologies enhances its application and reliability.
- Innovative Technologies: The emergence of new ionization methods and mass analyzers has improved detection limits and expanded the range of analytes that can be accurately measured. As such innovations unfold, professionals in the field must stay abreast of the advancements to fully realize their potential.
- Cross-Disciplinary Applications: As referenced earlier, the influence of mass spectrometry extends to various fields, including clinical diagnostics, environmental monitoring, and drug development. This versatility ensures its relevance in tackling current global challenges, like disease outbreak management and environmental sustainability.
- Training and Education: Continuous education on mass spectrometry practices and interpretation will ensure that newer generations of scientists can harness this powerful tool effectively. With the right training, individuals can contribute meaningfully to innovations and applications in their respective fields.