Mass Spectroscopic: Principles, Methods & Applications
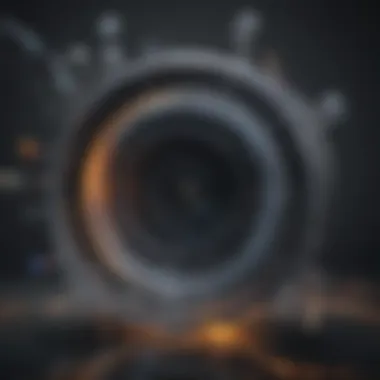

Intro
Mass spectrometry has emerged as a pivotal tool in contemporary scientific research, facilitating the analysis of complex mixtures and the identification of molecular structures. Its relevance spans widely across various disciplines, from chemistry to biology and environmental science. The intricacies underlying its operation are rooted in fundamental physical principles, making it essential to grasp its core methods and applications.
Understanding mass spectroscopy involves elucidating how molecules are ionized, their subsequent separation according to mass-to-charge ratios, and the detection of these ions. This foundational knowledge sets the stage for appreciating how mass spectrometry operates in practical settings.
Article Overview
Purpose of the Article
The purpose of this article is to provide a comprehensive exploration of mass spectrometry. By examining its principles, methods, and applications, readers will gain a deeper understanding of its significance in scientific research and industrial practices. Technological advancements in instrumentation and data analysis will also be discussed, underscoring the evolution of the field.
Relevance to Multiple Disciplines
Mass spectrometry plays a crucial role in various scientific domains, making it relevant to a wide audience. In chemistry, it aids in qualitative and quantitative analysis, while in biology, it is used for proteomics and metabolomics studies. Environmental science benefits from its ability to analyze pollutants and trace elements. The diverse applications highlight its utility as a versatile analytical tool and mark its importance in ongoing research and innovation.
Research Background
Historical Context
The history of mass spectrometry dates back to the early 20th century. The technique evolved significantly, with early pioneers like J.J. Thomson laying the groundwork through the development of the first mass spectrometer. From its inception to its current advanced variants, mass spectrometry has undergone technological transformation, making it faster and more accurate than ever.
Key Concepts and Definitions
To understand mass spectrometry, it is essential to familiarize with some key concepts:
- Ionization: The process where neutral molecules are converted into ions, priming them for analysis.
- Mass-to-charge ratio (m/z): A critical parameter measured during the analysis, it reflects the mass of the ion relative to its charge.
- Detection: The final stage involves detecting ions and generating a spectrum that delineates the composition of the sample.
Mass spectrometry's intricate processes, while complex, are foundational to grasping its applications and future potential in research.
Prologue to Mass Spectroscopy
Mass spectroscopy stands as a pivotal experimental approach employed in the analysis of chemical compounds. Its significance transcends the laboratory, influencing numerous fields such as pharmaceuticals, environmental science, and proteomics. Understanding mass spectroscopy involves dissecting its historical context and core principles, both of which provide a foundation for its vast applications in contemporary science.
Historical Development
The inception of mass spectrometry dates back to the early 20th century. J.J. Thomson is credited with the first mass spectrometer in 1913, utilizing a cathode ray tube to measure the mass-to-charge ratios of ions. Following that, in the 1940s, the introduction of the first successful mass spectrometry technique, known as the "time-of-flight" method, marked a significant advancement. During the latter half of the century, innovations flourished. The development of ionization techniques in the 1950s, such as electron ionization and chemical ionization, vastly improved detection capabilities and broadened the scope of analysis. The academic and industrial applications continued to grow, further solidifying mass spectrometry’s importance in scientific inquiry.
Core Principles of Mass Spectroscopy
At its core, mass spectrometry operates on a few fundamental principles. Analytes are ionized, producing charged particles. Following ionization, these ions are separated based on their mass-to-charge (m/z) ratios in a mass analyzer. Different types of ionization techniques can produce unique ions, influencing the outcome of the results. Once separated, ions reach a detector, generating a signal proportional to the abundance of each ion type.
Mass spectrometry allows for precise quantification and characterization of complex mixtures. The analysis can offer insight into molecular weights, structural information, and chemical properties.
Mass spectrometry allows for precise quantification and characterization of complex mixtures. The analysis can offer insight into molecular weights, structural information, and chemical properties.
The integration of mass spectrometry with other analytical methods, such as chromatography, further enhances its capabilities, allowing for the high-resolution analysis of biomolecules and materials. This interdisciplinary application underscores the relevance of mass spectrometry across various research and industrial fields.
Fundamental Components of Mass Spectrometry
Understanding the fundamental components of mass spectrometry is critical for grasping its efficiency and precision in analyzing various samples. These components work in concert to transform a sample into ionic fragments, separate them based on mass-to-charge ratio, and finally detect them for analysis. Each component plays a distinctive role, making it essential to comprehend their functions, advantages, and challenges.
Ion Source
The ion source is where the mass spectrometry process begins. This component converts molecules from the sample into ions. Ions are necessary because the subsequent analysis relies on measuring the mass-to-charge ratio of these charged particles. There are various ionization techniques available, such as Electron Ionization and Electrospray Ionization. Each technique has unique merits, depending on the sample type and desired outcomes.
Key considerations regarding ion sources include:
- Sample Compatibility: Different ionization methods work better with distinct sample types, such as solids, liquids, or gases.
- Ionization Efficiency: The ability to generate ions from a sample impacts the overall sensitivity of the analysis.
A biased ion source could lead to losses in sensitivity or selectivity, requiring careful selection and optimization for each specific analysis case.
Mass Analyzer
The mass analyzer is the core component responsible for separating ions based on their mass-to-charge ratio. Its efficacy dictates the resolution and accuracy of the mass spectrometry results. Among the different types of mass analyzers, the Quadrupole Mass Analyzer, Time-of-Flight (TOF) Analyzer, and Orbitrap Mass Analyzer are most noteworthy.
- Quadrupole Mass Analyzer: This is widely used for routine applications. It provides good resolution and is especially effective for qualitative and quantitative analysis.
- Time-of-Flight (TOF) Analyzer: It is known for its rapid analysis capabilities. TOF analyzers can measure a wide range of ions in a short duration, making them suitable for complex mixtures.
- Orbitrap Mass Analyzer: This analyzer excels in resolution. It offers high accuracy and is useful for large biomolecules, such as proteins and nucleic acids.
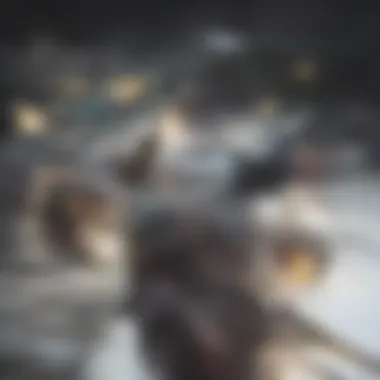
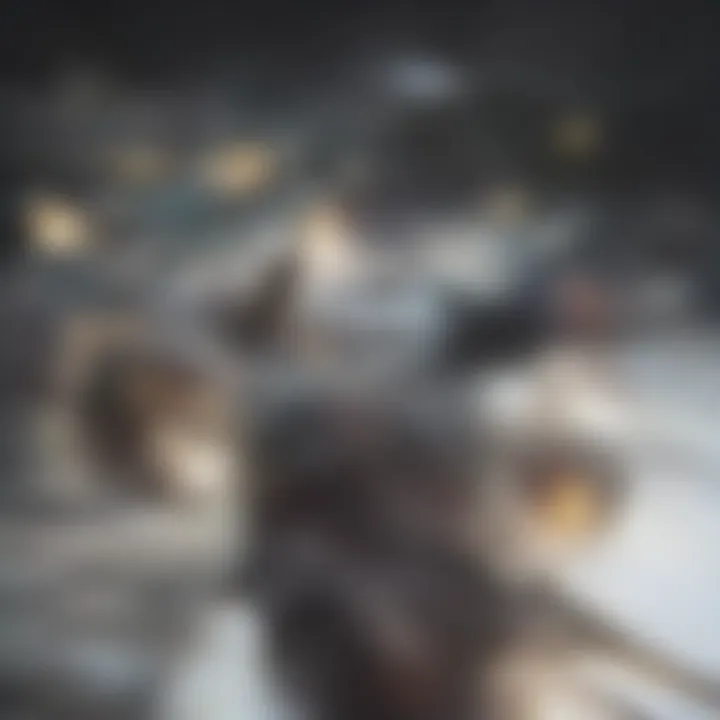
Selecting the right mass analyzer is crucial, as it can heavily impact data quality and analysis time. Each analyzer type brings its own advantages based on the specific application requirements.
Ion Detector
The ion detector is the final component responsible for measuring the separated ions and producing the data for analysis. It detects ions emerging from the mass analyzer and converts their impact into electrical signals. The two main types of detectors are Faraday Cups and Microchannel Plates.
Some critical factors about ion detectors include:
- Sensitivity: The detector must be sensitive enough to detect low-abundance ions without noise interference.
- Dynamic Range: A wide dynamic range allows for effective measurement across a broad spectrum of ion intensities.
- Response Time: Faster response times enable quick analyses, which is particularly beneficial in high-throughput environments.
Effective ion detection plays a key role in data interpretation and accuracy. It directly influences how well the ion spectra can be analyzed and correlated with sample information.
"The efficiency of mass spectrometry relies heavily on the integration and functionality of its core components: ion source, mass analyzer, and ion detector."
"The efficiency of mass spectrometry relies heavily on the integration and functionality of its core components: ion source, mass analyzer, and ion detector."
In essence, each component of mass spectrometry works together to create a robust analytical framework. Understanding these fundamentals offers insights into optimizing methods and improving results within the field of mass spectroscopic analysis.
Ionization Techniques
Ionization techniques are central to the process of mass spectrometry. They enable the conversion of sample molecules into ions, making it possible to analyze them based on their mass-to-charge ratios. The effectiveness of the ionization method can significantly influence the quality of the data obtained. Understanding these techniques is essential for researchers and professionals, as selecting an appropriate ionization method directly affects sensitivity, resolution, and reliability of results.
Electron Ionization
Electron ionization is one of the most commonly used ionization techniques in mass spectrometry. This method employs a beam of high-energy electrons to strip away one or more electrons from atoms or molecules, generating positive ions.
Key points to note about electron ionization include:
- Efficiency: It is very effective for small, volatile organic compounds.
- Fragmentation: This technique often leads to extensive fragmentation. This property can help in identifying molecular structures but may complicate quantitative analysis.
- Temperature Sensitivity: Since it requires the sample to be in the gas phase, it may not be suitable for thermally unstable or high-boiling substances.
This method has been widely employed in applications from environmental monitoring to pharmaceutical analysis. However, users must balance the advantages of high sensitivity against the challenges posed by ion fragmentation.
Chemical Ionization
Chemical ionization offers a gentler alternative to electron ionization. Instead of directly ionizing the sample, this technique involves ionizing a reagent gas, which then transfers energy to the sample molecule.
Important aspects of chemical ionization include:
- Milder Conditions: This method results in lower fragmentation compared to electron ionization, providing cleaner spectral data.
- Reagent Choice: Common reagents include methane, isobutane, and ammonia. The choice of reagent can influence the specific ion fragments observed.
- Applications: It is particularly useful in the analysis of large biomolecules and in cases where preserving the molecular structure is critical.
Chemical ionization can enhance sensitivity and reduce fragmentation, making it particularly suitable for more complex samples.
Electrospray Ionization
Electrospray ionization is a technique that has revolutionized the field of mass spectrometry, especially in the context of biological molecules. It works by applying a high voltage to a liquid sample, which produces an aerosol of charged droplets. As the solvent evaporates, these droplets produce ions suitable for analysis.
Key points about electrospray ionization include:
- Soft Ionization: This method is highly efficient for large biomolecules like proteins and nucleic acids, as it minimizes fragmentation.
- Compatibility: It is compatible with liquid chromatography, allowing for the analysis of complex mixtures directly.
- Wide Dynamic Range: ESI can analyze a broader range of concentrations, from trace levels to high concentrations effectively.
Usage of electrospray ionization extends to proteomics and metabolomics, among other fields.
In summary, the choice of ionization technique can determine the success of a mass spectrometry analysis. Understanding the unique properties, advantages, and limitations of each method is essential for optimizing results in various applications.
In summary, the choice of ionization technique can determine the success of a mass spectrometry analysis. Understanding the unique properties, advantages, and limitations of each method is essential for optimizing results in various applications.
Mass Analyzers Explained
In mass spectrometry, the mass analyzer is a crucial component that determines the mass-to-charge ratio of ions. This process is key to obtaining information about the molecular weight and structure of analytes. The specific choice of mass analyzer affects the performance characteristics, including resolution, accuracy, and sensitivity. Understanding the various types of mass analyzers is therefore essential for both practitioners and researchers in the field. They enable the refinement of data analysis and can lead to more accurate quantification in complex samples.
Quadrupole Mass Analyzer
The Quadrupole Mass Analyzer is one of the most commonly used types in mass spectrometry. It operates using four parallel rods that create an oscillating electric field. This configuration allows it to filter ions based on their mass-to-charge ratio. The ions are directed through the quadrupole where only ions of a specific mass can pass at any given time.
Quadrupoles are favored for their ease of use and robustness. They are capable of operating in both selected ion monitoring (SIM) and full scan modes. This versatility makes them suitable for a variety of applications, particularly in quantitative analysis. Furthermore, the response time is quite fast, allowing for high-throughput analysis, which is critical in many research settings.
Time-of-Flight (TOF) Analyzer
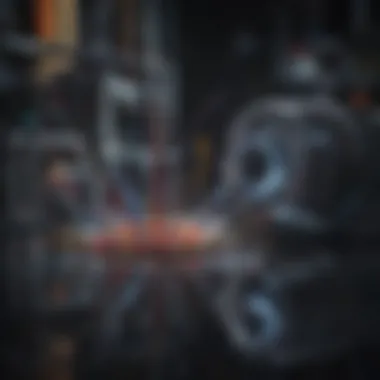
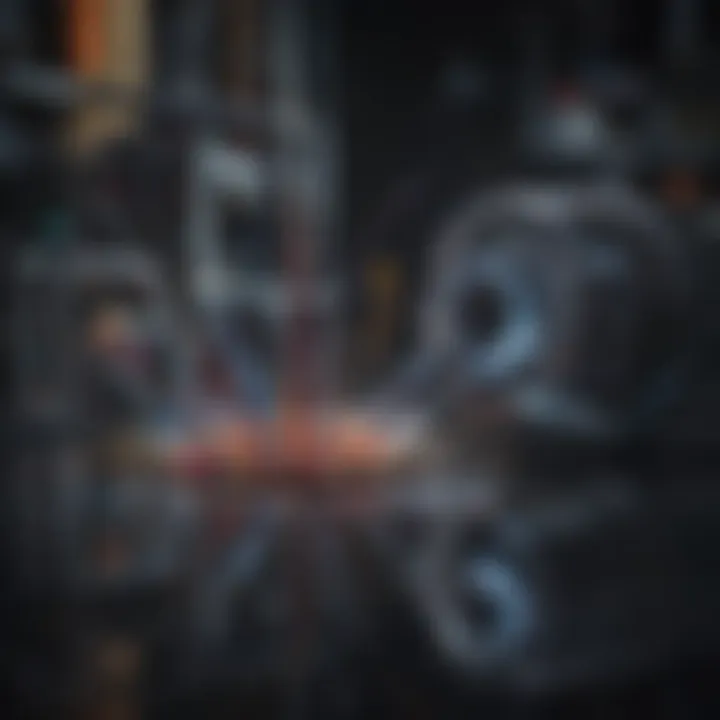
The Time-of-Flight (TOF) Analyzer provides another distinct method for analyzing ions. In this setup, ions are accelerated using a high voltage and then allowed to drift through a field-free region. The time it takes for an ion to reach the detector correlates directly to its mass-to-charge ratio.
TOF analyzers are known for their high mass resolution and accuracy. They can measure the mass of very large biomolecules such as proteins and polymers. With the addition of reflectron technology, which corrects for kinetic energy variations among ions, they offer enhanced resolution. The speed of TOF systems allows for the analysis of complex mixtures in a short amount of time, which is invaluable in proteomics and metabolomics studies.
Orbitrap Mass Analyzer
The Orbitrap Mass Analyzer is a relatively recent innovation in the field. It traps ions in an orbital motion around a central electrode. The frequency of this motion is detected and converted into a mass spectrum. This design leads to high stability and resolution, often surpassing that of other types of analyzers.
One of the significant advantages of the Orbitrap is its extremely high resolving power and sensitivity. It has gained popularity particularly in proteomics and small molecule studies. The ability to analyze complex samples with minimal preparation makes Orbitrap mass analyzers a preferred choice for many researchers interested in detailed molecular characterization. However, they tend to have a longer acquisition time compared to quadrupoles, which might impact throughput in some scenarios.
The choice of mass analyzer ultimately depends on the specific requirements of the analysis, including factors like resolution, speed, and sample complexity.
The choice of mass analyzer ultimately depends on the specific requirements of the analysis, including factors like resolution, speed, and sample complexity.
Applications of Mass Spectrometry
Mass spectrometry (MS) has grown to be an essential analytical technique across various scientific domains. Its applications are as diverse as they are vital, ranging from proteomics and metabolomics to environmental analysis. Understanding these applications is crucial because they demonstrate not only the versatility of mass spectrometry but also its potential to impact areas such as medicine, environmental science, and industrial processes. This section delves into specific applications, highlighting their importance in research and practical usage.
Proteomics
Proteomics, the large-scale study of proteins, has prominently benefited from mass spectrometry. The ability of MS to identify and quantify proteins in complex mixtures makes it indispensable in this field. Mass spectrometry can analyze post-translational modifications, protein interactions, and the dynamics of protein expression under different conditions.
One distinct advantage of using mass spectrometry in proteomics is its sensitivity. This characteristic allows scientists to detect proteins at low concentrations, which is often crucial in biological samples. Furthermore, techniques such as tandem mass spectrometry enhance the detailed analysis of specific peptides derived from proteins. This enables researchers to construct comprehensive protein profiles, facilitating advances in drug discovery, biomarker identification, and understanding disease mechanisms.
Metabolomics
Metabolomics focuses on the comprehensive analysis of metabolites within biological systems. Mass spectrometry plays a significant role in this discipline as it allows the detection and quantification of a wide array of metabolites from complex biological samples. The adaptability of MS in analyzing different classes of metabolites, like lipids, amino acids, and sugars, makes it a powerful tool in metabolic profiling.
By integrating mass spectrometry with techniques such as gas chromatography and liquid chromatography, researchers can achieve high throughput in their analyses. This aspect is critical in clinical studies where rapid and accurate metabolite profiling can lead to early disease detection and better therapeutic strategies. Moreover, the data obtained can help in understanding metabolic pathways and their shifts due to disease or environmental factors.
Environmental Analysis
Another prominent application of mass spectrometry is in environmental analysis. The technique allows for the detection and quantification of pollutants in air, soil, and water samples. Mass spectrometry provides a level of precision that is necessary for monitoring environmental contaminants and ensuring compliance with regulatory standards.
In this context, MS can identify trace levels of pesticides, heavy metals, and organic compounds. This capability is vital for assessing the health of ecosystems and ensuring public safety. Efficient sample processing and method development using mass spectrometry can lead to quicker turnaround times in environmental testing, which is crucial in urgent situations such as contamination events. The detailed chemical information obtained enables stakeholders to take informed decisions regarding pollution management and remediation strategies.
Mass spectrometry is a cornerstone technology in modern analytical chemistry, providing precise measurements that shape research landscapes across multiple disciplines.
Mass spectrometry is a cornerstone technology in modern analytical chemistry, providing precise measurements that shape research landscapes across multiple disciplines.
In summary, the applications of mass spectrometry in proteomics, metabolomics, and environmental analysis underscore its importance as a versatile analytical tool. The implications of its use extend beyond just measurement; they impact research, clinical diagnostics, and environmental conservation efforts significantly. The continued advancements in mass spectrometry technologies are likely to expand its applications further, offering new opportunities for scientific exploration and practical solutions.
Data Analysis and Interpretation
Data analysis and interpretation play a crucial role in mass spectrometry. This aspect process the vast amounts of data generated during experiments. Understanding how to analyze this data helps researchers draw meaningful conclusions about the samples they study. It involves several components that enhance accuracy and reliability.
Mass Spectral Data Processing
The first step in data analysis is mass spectral data processing. This step involves transforming raw data from the mass spectrometer into a usable form. The data typically consists of peaks that represent ions, with each peak showing the mass-to-charge ratio. Processing includes noise reduction, baseline correction, and peak detection.
These steps aim to enhance the signal quality while removing irrelevant information. By using algorithms and software tools, researchers can fine-tune the data. This results in clearer and more precise spectra. Quality data is fundamental for accurate interpretations in subsequent analyses.
Quantitative Analysis Techniques
Quantitative analysis techniques in mass spectrometry assess the concentration of specific compounds in samples. Various methods exist, but some of the most common techniques include calibration curves and internal standards.
- Calibration Curves: This method involves plotting the measured signal against known concentrations. By establishing a standard curve, researchers can quantify unknown samples based on their response.
- Internal Standards: This technique uses a compound that is similar to the target analyte. It helps correct variations that occur during sample preparation and analysis.
Both methods ensure the accuracy and reliability of quantitative measurements.
Software Tools for Mass Spectrometry
The integration of software tools in mass spectrometry has revolutionized data analysis. Several programs assist in various tasks ranging from data acquisition to complex analysis. Commonly used software includes ProteoWizard, MaxQuant, and MZmine. These tools can automate processes, thus minimizing human error and saving time.
Features often include:
- Data Visualization: Helps in easily interpreting complex data sets through graphical representations.
- Automated Processing: Reduces manual intervention, ensuring consistency in results.
- Statistical Analysis: Provides essential statistical tools to validate findings, enhancing the credibility of the research output.
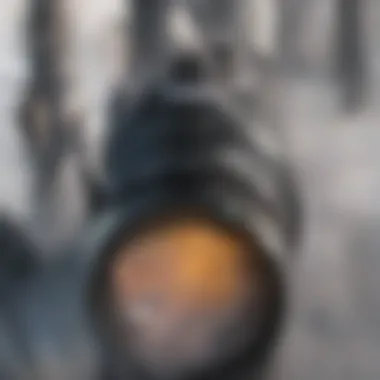
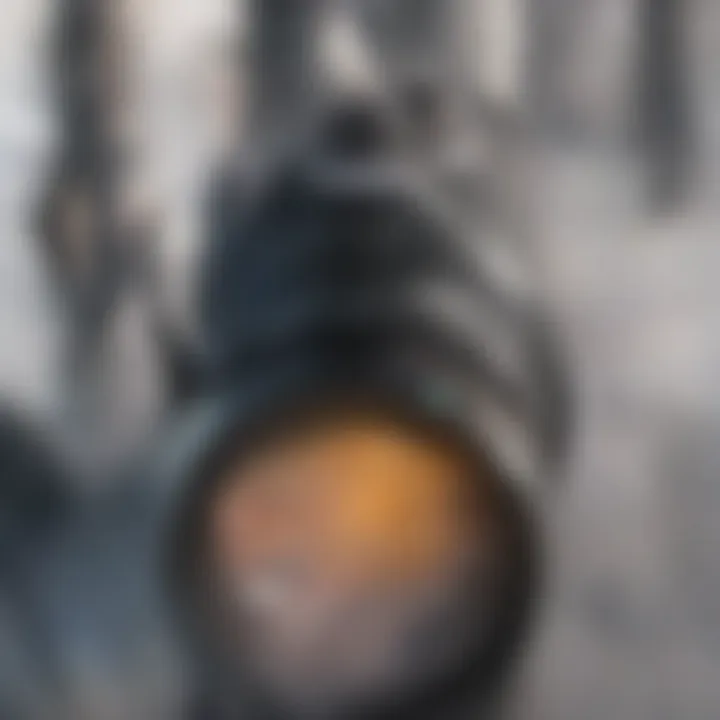
As mass spectrometry continues to advance, the importance of effective data analysis and interpretation remains paramount. Understanding data allows researchers to maximize the potential and impact of their scientific findings.
Challenges in Mass Spectrometry
Mass spectrometry, despite its powerful capabilities, faces some challenges that researchers must navigate to fully harness its potential. Understanding these challenges is crucial for improving analytical methods and achieving better results in various applications. Here, we explore two specific challenges: ion suppression effects and the complexity introduced by sample matrices.
Ion Suppression Effects
Ion suppression occurs when the presence of certain molecules in a sample inhibits the ionization of analytes. This phenomenon can lead to unreliable quantification and detection limits. Ion suppression is particularly problematic in complex biological samples, such as blood or tissues, where a wide variety of compounds may coexist.
Several factors influence ion suppression, such as the physicochemical properties of the matrix components and the chosen ionization technique. For example, in Electrospray Ionization, the solvent composition plays a significant role. A high concentration of salts or detergents can alter the ionization efficiency, leading to skewed analytical results.
To tackle ion suppression, researchers use several strategies:
- Sample Preparation: Techniques such as solid-phase extraction or protein precipitation can improve analyte isolation and decrease matrix interference.
- Dilution: Diluting samples can reduce the matrix effect, although care must be taken to ensure that the analyte remains within quantifiable limits.
- Quality Control: Implementing internal standards can help in compensating for variability introduced by ion suppression.
"Recognizing and minimizing ion suppression is a vital step in ensuring the accuracy and reliability of mass spectrometric analysis."
"Recognizing and minimizing ion suppression is a vital step in ensuring the accuracy and reliability of mass spectrometric analysis."
Complex Sample Matrices
Complex sample matrices present another substantial challenge in mass spectrometry. These matrices can include biological fluids, environmental samples, or food products, which often contain numerous interfering substances. Such complexity can obscure the signals of the target analytes, complicating both detection and quantification processes.
The intricate nature of these matrices requires advanced methods for sample preparation and analysis. Choosing the appropriate ionization technique is essential. For example, while Electrospray Ionization might be well-suited for polar compounds, it may not effectively ionize more hydrophobic substances.
To address the challenges posed by complex matrices, several approaches can be adopted:
- Matrix Removal Techniques: Utilizing methods like liquid-liquid extraction can help eliminate unwanted components from a sample, isolating the target analytes better.
- Advanced Instrumentation: Instruments equipped with high-resolution mass analyzers, like Orbitraps, can help distinguish signals from overlapping peaks, enhancing sensitivity and specificity.
- Method Development: Tailoring the mass spectrometric method to the specific sample type can significantly improve analyte detection.
Future Directions in Mass Spectroscopy
The landscape of mass spectroscopy is evolving. As the need for precise and detailed analysis in various scientific fields increases, so does the importance of understanding the future directions of this technology. Innovations in instrument design, data processing, and analytical methods hold significant promise. These advancements will likely enhance the overall capability and application of mass spectrometry in research and industry.
Understanding these developments allows researchers and professionals to stay ahead in their respective fields. The mentioned future directions not only enhance analytical accuracy but also drive new applications in areas like personalized medicine, environmental monitoring, and materials science.
Emerging Technologies
Emerging technologies in mass spectrometry are reshaping its applications. One noteworthy advancement is the development of miniaturized mass spectrometers. These devices are smaller, more portable, and capable of delivering quick results. They have significant applications in field studies, such as environmental assessments and on-site testing in clinical settings.
Additionally, user-friendly interfaces make these systems accessible to a wider range of users. New ionization techniques, like laser ablation, are facilitating the analysis of solids with high spatial resolution. These technologies are not only increasing throughput but also permitting the analysis of previously challenging samples.
"The future of mass spectrometry is marked by innovations that promise to make this valuable analytical tool more versatile and user-friendly than ever before."
"The future of mass spectrometry is marked by innovations that promise to make this valuable analytical tool more versatile and user-friendly than ever before."
Advancements in Sensitivity and Resolution
The sensitivity and resolution of mass spectrometers are critical for accurate analysis. Recent advancements focus on improving these parameters. Increasing sensitivity allows for the detection of low-abundance compounds that were previously challenging to identify. Scientists are now working on novel ion optics designs and enhanced detector technologies.
Mass spectrometers with better resolution can distinguish between compounds that are very similar in mass. This capability is vital for applications in proteomics, where subtle differences in mass can imply significant biological variations. Techniques like tandem mass spectrometry (MS/MS) further push the boundaries of both sensitivity and resolution, enabling in-depth structural elucidation of complex molecules.
Furthermore, advancements in isobaric tagging and differential mass spectrometry techniques contribute to high-throughput proteomic analysis, improving both speed and accuracy.
The End
The conclusion serves as a critical aspect of this article. It distills the knowledge shared throughout each section, reinforcing the significance of mass spectrometry in various scientific disciplines. This technique does not only provide insights into the composition and structure of atoms and molecules but also bridges understanding across chemistry, biology, and environmental studies.
In today's research landscape, the ability to accurately analyze samples is paramount. Mass spectrometry stands out for its precision and ability to handle complex sample matrices. Its significance becomes evident as we consider its wide array of applications—ranging from proteomics to environmental analysis. This versatility indicates the relevance and urgency of continued research and development in the field.
Summary of Key Points
- Mass Spectrometry Basics: Emphasis on the core principles underlying mass spectrometric analysis, including the fundamental components like the ion source, mass analyzer, and detector.
- Ionization Techniques: Various methods, such as Electron Ionization and Electrospray Ionization, play a pivotal role in sample preparation and data quality.
- Data Analysis: The importance of software tools and quantitative techniques for interpreting mass spectral data aids in various research domains.
- Current Challenges: Understanding and addressing issues like ion suppression effects and managing complex sample matrices is vital for accuracy.
- Future Directions: Innovations in technology and advancements in sensitivity and resolution will likely shape the future of mass spectrometry.
Implications for Future Research
The future of mass spectrometry is promising and warrants further exploration. Emerging technologies will enhance its capabilities, making it an even more powerful tool for researchers. Advances in sensitivity could facilitate the detection of rare compounds, broadening its applicability in fields like biomarker research.
Moreover, the development of new ionization techniques might mitigate current challenges. For instance, improving methods to minimize ion suppression could lead to better results in diverse environmental samples.
In terms of software, machine learning and big data analytics hold the potential to revolutionize data interpretation. Automating data analysis will not only save time but also improve accuracy, ensuring that results are reliable. The scientific community must focus on collaborative efforts to address existing challenges and explore new possibilities in mass spectrometry.
Ultimately, the continuous evolution of mass spectrometry reflects the dynamic nature of scientific inquiry and the relentless pursuit of knowledge. The implications of improved mass spectrometric techniques will resonate across research fields, making this an essential area for future study.
Ultimately, the continuous evolution of mass spectrometry reflects the dynamic nature of scientific inquiry and the relentless pursuit of knowledge. The implications of improved mass spectrometric techniques will resonate across research fields, making this an essential area for future study.