Optogenetics: Transforming Neuroscience Methodologies
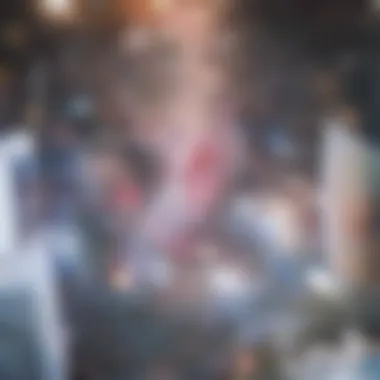
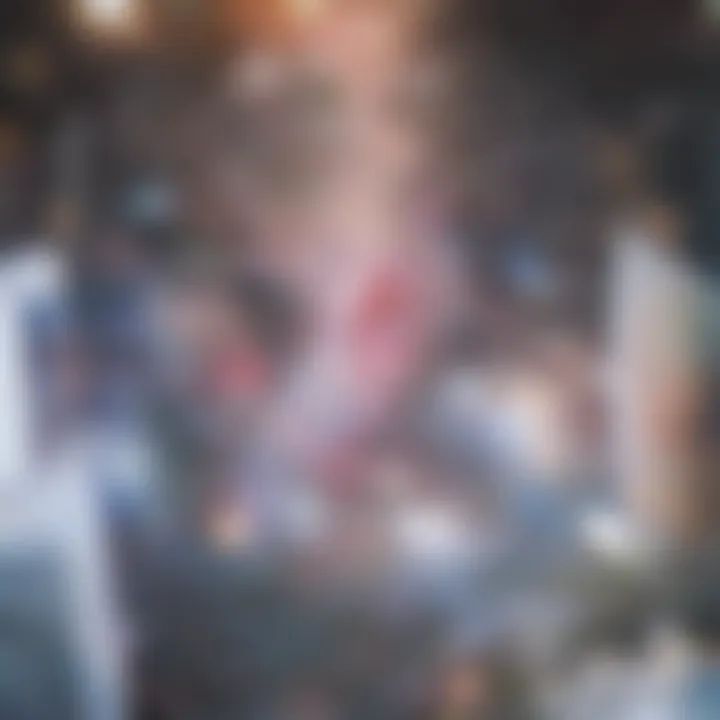
Intro
Optogenetics is an innovative field that has transformed neuroscience by allowing researchers to manipulate and monitor specific neurons with high precision. By integrating genetic engineering with light-controlled techniques, scientists can explore the intricate workings of the brain in real time. This article provides a detailed examination of optogenetics, covering its methodologies, historical development, and significant contributions to experimental neuroscience.
Article Overview
Purpose of the Article
The purpose of this article is to elucidate the principles and applications of optogenetics in neuroscience. It aims to detail how this technique enables the control of neuronal activity through light, leading to a deeper understanding of neural circuits and behaviors. By reviewing various methodologies and their implications, this piece seeks to inform readers about the advancements optogenetics offers.
Relevance to Multiple Disciplines
Optogenetics spans various facets of research beyond neuroscience. Its applications can be seen in psychology, psychiatry, and even some areas of regenerative medicine. As these fields revolve around understanding behavior, mental processes, and cellular mechanisms, optogenetics provides a valuable tool for investigating these areas with unparalleled specificity. This relevance showcases not only its impact on neuroscience but also its importance across interdisciplinary research.
Research Background
Historical Context
Optogenetics has its roots in a blend of genetic engineering and optics, drawing on decades of research in both fields. The discovery of light-sensitive proteins, such as channelrhodopsins, paved the way for researchers to selectively activate neurons using light. In the early 2000s, Karl Deisseroth and Ed Boyden, among others, were pivotal in developing these techniques, demonstrating their transformative potential in probing the brain's complex circuitry.
Key Concepts and Definitions
Optogenetics relies on several foundational concepts:
- Channelrhodopsins: These are light-activated ion channels that facilitate the depolarization of neurons.
- Halorhodopsins: Proteins that inhibit neuronal activity in response to specific wavelengths of light.
- Targeted Delivery: Methods, such as viral vectors, are used to introduce these proteins into specific neuron populations, ensuring precise control.
Experiments utilizing optogenetics involve delivering light to targeted areas of the brain, allowing researchers to modulate neuronal activity. This level of control offers insights into behavior, cognition, and various neural processes.
"Optogenetics stands as a bridge between theoretical neuroscience and practical applications, making it essential for neuroscience research today."
"Optogenetics stands as a bridge between theoretical neuroscience and practical applications, making it essential for neuroscience research today."
The exploration of these concepts lays the groundwork for understanding the methodologies and applications discussed in subsequent sections.
Prolusion to Optogenetics
Optogenetics represents a groundbreaking approach within the realm of neuroscience. Its main feature is the ability to control neuronal activity through light. This innovative technique enables researchers to take precise measurements and manipulate the behavior of specific neurons. The introduction of optogenetics to neuroscience has opened new avenues for understanding brain function and disorders. It allows for dissecting the complex interplay between various neuronal circuits, which was previously challenging with traditional methods.
The significance of this approach lies in its ability to provide temporal and spatial control over neuronal activity. In classical techniques, it is often difficult to determine which neurons are active and when. Optogenetics enhances the granularity of this analysis, opening possibilities to investigate neuronal behavior in real-time.
Definition and Overview
Optogenetics involves the genetic modification of neurons to express light-sensitive ion channels, such as channelrhodopsins and halorhodopsins. Researchers can then use light to activate or inhibit these modified neurons, thus controlling their activity. This manipulation can lead to insights into both normal and pathological states of the brain.
The process starts with the introduction of the genes encoding these light-sensitive proteins into the target neurons. Subsequently, these neurons, when illuminated with specific light wavelengths, can exhibit excitatory or inhibitory responses depending on the ion channel utilized. For instance, blue light typically activates channelrhodopsins, leading to neuronal excitation, whereas yellow or green light is often employed to activate halorhodopsins, causing inhibition.
Historical Context
The concept of optogenetics emerged from earlier research in neuroscience and genetic engineering. The initial ideas date back to the early 2000s when Karl Deisseroth, Ed Boyden, and Feng Zhang began developing this approach. They drew inspiration from the study of microorganisms such as algae and bacteria, which utilize light-sensitive proteins for various biological processes.
The first applications of optogenetics were primarily in animal models, enabling unprecedented exploration of the brain’s complex circuitry. As research progressed, the utility of optogenetics became evident across diverse fields, including neurobiology, psychology, and physiology. These advancements not only boosted our understanding of basic neural processes but also highlighted potential therapeutic avenues for neurological disorders.
Principles of Optogenetics
Understanding the principles of optogenetics is crucial to appreciating its impact on modern neuroscience. This approach enables researchers to observe and manipulate neuronal activity with remarkable precision. By combining optical techniques with genetic engineering, optogenetics provides insight into the complex workings of the brain. It allows scientists to selectively target specific populations of neurons, which is essential for deciphering the neural circuits involved in various functions and behaviors.
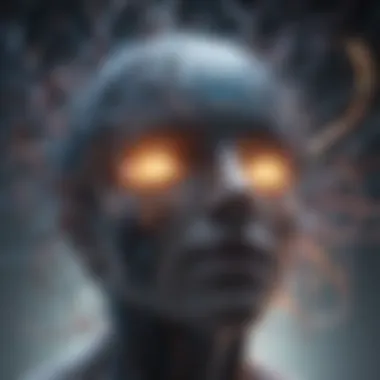
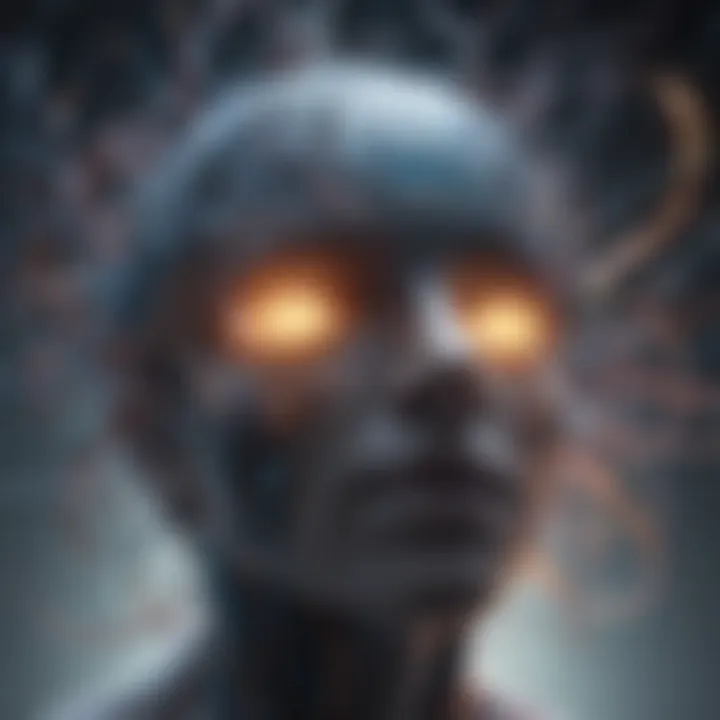
In today's fast-paced scientific landscape, the principles of optogenetics have revolutionized experimental paradigms. They have made it possible to investigate neurological functions that were previously difficult to study. Furthermore, this technique has opened avenues for therapeutic applications that can restore or enhance neuronal functions in a variety of conditions. Understanding these principles also emphasizes the continual integration of technology and biology, representing significant progress for both fields.
Genetic Modification Techniques
At the heart of optogenetics lies genetic modification techniques. These are methods used to introduce light-sensitive proteins into specific neuronal populations. The most commonly used proteins originate from algae and bacteria. The introduction of these proteins into the target neurons often occurs through a viral vector, such as adeno-associated viruses. Viruses can effectively deliver genetic material to neurons, ensuring targeted expression of the optogenetic tools.
Once expressed, these proteins can respond to light, which allows controlled stimulation of the neurons. One of the most widely used proteins is channelrhodopsin-2, which opens ion channels upon exposure to blue light. This leads to neuronal depolarization, activating the neuron in a controlled manner. Conversely, halorhodopsin can inhibit neuronal activity when exposed to yellow light. Such precision in genetic modification enables detailed studies on how specific neurons influence behavior and physiological states.
Optical Control: Mechanisms and Tools
The optical control aspect of optogenetics involves the tools and mechanisms that enable the precise modulation of neuronal activity. Light delivery is typically done using fiber optics or LED systems. Fiber optics allow for targeted light application to a specific brain region, reducing collateral activation of nearby neurons. Additionally, LED systems can be integrated for flexible control of light parameters, such as wavelength and intensity.
"The choice of optical instruments can greatly affect the outcomes of optogenetic experiments."
"The choice of optical instruments can greatly affect the outcomes of optogenetic experiments."
Different wavelengths of light are critical as they correspond to the specific activation or inhibition of the optogenetic proteins. Thus, one must ensure that appropriate equipment is used based on the chosen optogenetic tool. Tools like multi-channel optogenetic stimulators have emerged to control multiple regions of the brain simultaneously, which enhances the capability of experimental designs.
Optical control not only encompasses the delivery mechanisms but also the detection of neuronal responses. By combining optogenetics with other imaging techniques, such as calcium imaging or electrophysiology, researchers can gain insights into how neurons communicate within circuits. Therefore, understanding these optical control mechanisms is essential for advancing optogenetic research and its applications in dissecting neural circuit functions.
Experimental Methodologies
Understanding the experimental methodologies in optogenetics is crucial for harnessing its potential in neuroscience. These methodologies provide the framework that enables scientists to probe deeper into neural mechanisms using light to control genetically modified cells. Properly designed methodologies facilitate reproducibility, enhance the validity of findings, and ensure the reliability of the outcomes. This section focuses on three key areas: designing optogenetic constructs, implementing these constructs in vivo, and analyzing the data obtained from these experiments.
Designing Optogenetic Constructs
The initial step in conducting optogenetic experiments involves designing optogenetic constructs. These constructs typically consist of three components: a promoter that drives expression of a light-sensitive protein, the coding region for the opsin gene, and elements for ensuring stable integration into the host genome. The choice of promoter is particularly important because it dictates the specific cells that will express the opsin. Commonly used opsins include Channelrhodopsin-2 and Halorhodopsin, which allow for the excitation and inhibition of neurons respectively.
During the design phase, researchers must also consider the appropriate method of delivery. Viral vectors are often employed due to their efficiency in transducing neurons. Several types of viral vectors are available, such as lentiviruses or adeno-associated viruses, each with its advantages and limitations. The careful selection of these components is vital because it directly influences the precision of the light control over the targeted neural circuits.
In Vivo Implementation
In vivo implementation refers to the application of optogenetic constructs within living organisms. This phase is critical in bridging in vitro findings to physiological relevance. Prior to implementation, researchers must confirm the expression of the opsin in the intended neuronal populations using techniques such as immunohistochemistry or in situ hybridization.
Once confirmed, optogenetic tools are paired with a light delivery system, commonly fiber optics or LED-based systems. These systems allow for targeted illumination of neurons with specific wavelengths of light, inducing either activation or inhibition. The timing and duration of light application can be precisely controlled, allowing for the exploration of both acute and chronic effects on behavior and physiology.
In vivo experiments often involve the use of animal models such as mice or rats, which necessitates careful ethical considerations. The welfare of the animals must be a priority, and adherence to institutional guidelines is essential.
Data Acquisition and Analysis
Upon performing optogenetic experiments, the next critical step involves data acquisition and analysis. This stage transforms observed phenomena into quantifiable data. Techniques like electrophysiology and calcium imaging are frequently utilized to assess neuronal activity. Electrophysiological recordings allow for direct measurement of action potentials in neurons, while calcium imaging offers insights into the dynamics of neuronal firing in larger populations.
Data analysis often involves complex statistical techniques to validate results. It is important to compare control and experimental groups to ascertain the significance of the findings. Tools like MATLAB or R can facilitate these analyses, providing robust software for handling large datasets and performing multivariate tests.
Proper data acquisition and analysis techniques are key to substantiating the claims made in optogenetics research, ensuring that scientists maintain integrity and reliability in their work.
Proper data acquisition and analysis techniques are key to substantiating the claims made in optogenetics research, ensuring that scientists maintain integrity and reliability in their work.
Applications of Optogenetics in Neuroscience
The applications of optogenetics in neuroscience represent a pivotal area of study. This innovative approach enables precise control of neuronal activity using light, which opens new avenues for understanding the complex brain functions. Various specific elements highlight the relevance of this field. The ability to selectively activate or inhibit specific neurons provides unparalleled insights into neural circuitry and behavior. This technique enables researchers to unravel mechanisms underlying various neurological conditions, offering potential therapeutic strategies.
Mapping Neural Circuits
Optogenetics has revolutionized the way researchers map neural circuits. Traditionally, scientists relied on static models and indirect measures of neuronal connections. However, optogenetics allows for real-time observation of circuit activity. By utilizing light-sensitive proteins, researchers can manipulate neuronal firing and observe the resulting effects on behavior and neural connectivity. This leads to a more accurate and dynamic understanding of how circuits function during various tasks.
For instance, in studies involving ChR2 (Channelrhodopsin-2), researcher can stimulate specific populations of neurons while monitoring animal behavior. By documenting changes before and after modulations, they can ascertain the role of particular circuits in processes such as decision-making or movement. Furthermore, technology such as fiber optics enhances the precision of these interventions, enabling deeper insights into the brain's intricate wiring.
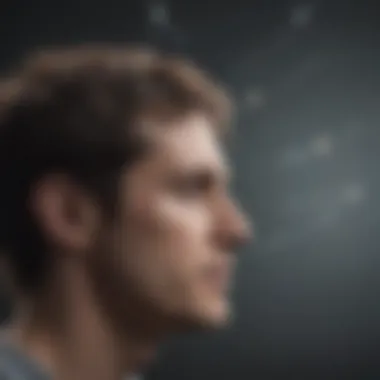
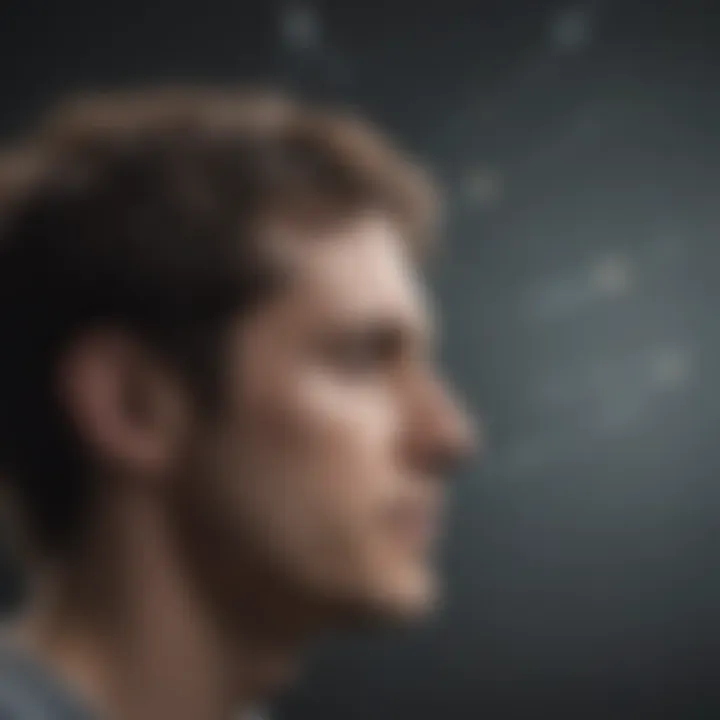
Behavioral Studies of Model Organisms
Using optogenetics in behavioral studies with model organisms like Drosophila or mice has opened new pathways for understanding behavior at a cellular level. By activating or inhibiting certain neural pathways, researchers can closely examine the correlation between neural activity and behavioral outcomes. This granularity is essential in fields such as neuroethology, where small changes in neuronal firing can significantly affect behavior.
For example, studies have shown that activating specific neurons can induce behaviors such as aggression or mating. Conversely, inhibiting others can curtail these behaviors. Insights gained from such studies are crucial. They highlight not only the complexity of behavioral control but also the potential targets for therapeutic intervention in conditions where these behaviors become dysregulated, such as in certain mood disorders.
Investigating Neurotransmission Dynamics
Optogenetics provides a robust framework for investigating neurotransmission dynamics. This approach allows direct monitoring of neurotransmitter release in live tissues with high temporal resolution. Understanding neurotransmission dynamics is critical for unveiling the underlying mechanisms of synaptic plasticity, learning, and memory.
By combining optogenetics with electrophysiological techniques, researchers are equipped to measure the immediate effects of specific neural triggers on neurotransmitter release. The ability to study how different neurotransmitters interact with neural circuits in real time enables the exploration of complex questions regarding synaptic communication. This might lead to identifying potential pharmacological targets that could ameliorate dysfunctions in neurotransmission, which are often observed in conditions such as depression and schizophrenia.
"Optogenetics is a game changer in neuroscience, allowing unprecedented insights into the functioning of neural circuits and their effects on behavior."
"Optogenetics is a game changer in neuroscience, allowing unprecedented insights into the functioning of neural circuits and their effects on behavior."
In summary, the applications of optogenetics in neuroscience are vast and varied. This technique not only enhances our understanding of the brain's complex workings but also holds promise for developing targeted therapies for neurological and psychological disorders.
Therapeutic Potential of Optogenetics
Optogenetics holds significant promise as a therapeutic strategy in neuroscience. Its capacity to manipulate neural activity with high spatiotemporal precision provides researchers and clinicians a unique tool for understanding and treating various neurological conditions. This approach merges genetics with optical engineering, offering potential solutions where traditional methods may fall short. The exploration of this therapeutic potential is crucial, as it may lead to innovative treatments and improved patient outcomes across a spectrum of disorders.
Optogenetic Approaches in Neurodegeneration
Neurodegeneration refers to the progressive loss of neuronal function and structure, commonly seen in diseases like Alzheimer’s and Parkinson’s. Optogenetic techniques present an exciting avenue for intervention. By selectively activating or inhibiting neuronal populations involved in these conditions, researchers aim to restore lost functionalities.
Key considerations include:
- Targeted Delivery: Specific optogenetic constructs can be delivered to precise neuronal populations, allowing for tailored treatment approaches.
- Behavioral Assessment: A direct impact on motor and cognitive functions can be measured through behavioral studies, offering insight into the effectiveness of therapies.
- Potential Risks: Safety is paramount. Researchers must consider the risks and ensure that the light used does not cause tissue damage or unintended activation of surrounding neurons.
Optogenetic methods have shown promise in animal models. For example, enhancing the function of dopaminergic neurons in Parkinson’s models has resulted in improved motor control. Future research might refine these techniques further, leading to clinical applications with the potential for significant patient impact.
Applications in Mental Health Disorders
Mental health disorders such as depression, anxiety, and PTSD pose complex challenges in treatment. Optogenetics allows for the investigation of neural circuits involved in these conditions, focusing on modulation rather than systemic pharmacological approaches.
Considerations include:
- Circuit Specificity: Distinct neural circuits linked to emotional regulation can be selectively targeted, optimizing therapeutic outcomes.
- Rapid Modulation: The ability to quickly alter neuronal activity provides insights into immediate and long-term effects on mood and behavior.
- Ethical Implications: There are ethical concerns surrounding the manipulation of emotions and thoughts. Responsible use of optogenetics requires thorough consideration of these issues.
Research has indicated that optogenetic stimulation of specific brain regions can alleviate symptoms in preclinical models. For instance, activating the medial prefrontal cortex has yielded promising results for anxiety symptom reduction. This illustrates the potential for personalized mental health treatments driven by precise neural manipulation.
"Optogenetics not only enhances our understanding of mental health disorders but also opens new pathways for therapeutic development, reflecting a synthesis of biology and technology."
"Optogenetics not only enhances our understanding of mental health disorders but also opens new pathways for therapeutic development, reflecting a synthesis of biology and technology."
As neuroscience continues to integrate optogenetic techniques, the therapeutic potential for both neurodegenerative diseases and mental health disorders becomes increasingly relevant. This cutting-edge strategy might shape the future of personalized medicine, leading to breakthroughs that fundamentally change treatment paradigms in these critical areas.
Challenges and Limitations
Understanding the challenges and limitations of optogenetics is essential for grasping the current landscape of neuroscience research. Despite its success and potential, optogenetics is not without hurdles. These issues can hinder advancements and must be addressed for the field to progress. Focusing on technological constraints and ethical considerations provides a well-rounded view of where optogenetics stands today.
Technological Constraints
Optogenetics has made significant strides, yet certain technological constraints can limit its efficacy. One major constraint is the delivery of optogenetic tools. Delivering opsins effectively to specific cell types within the target area remains a challenge. Various methods exist, such as viral vectors and transgenic approaches, but each comes with its limitations in terms of efficiency and specificity.
Another concern is the precision of light delivery. The effectiveness of optogenetic stimulation depends heavily on accurate light targeting. Variability in light penetration through biological tissues can lead to unintended activation of surrounding neurons. There are also concerns regarding the spatial resolution of light delivery. Ensuring that only the intended cells are activated is crucial for reliable results in experimental studies.
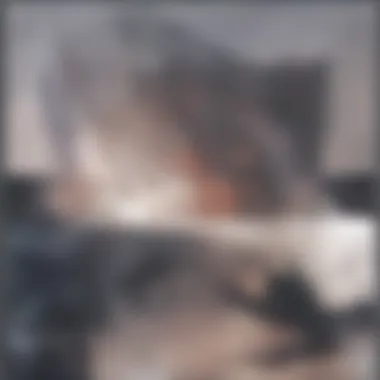
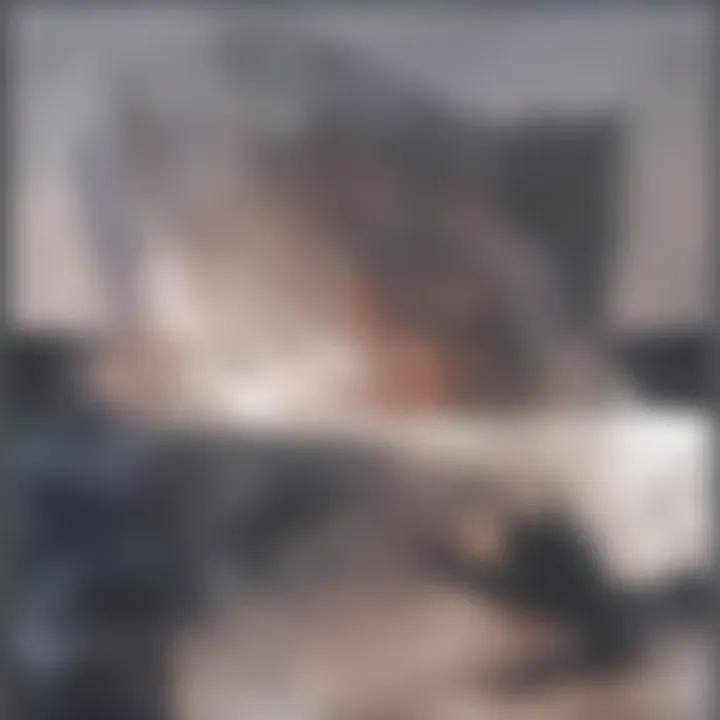
Moreover, the long-term expression of opsins can eventually lead to a decline in performance. Over time, the hosts' immune response can hinder the expression of opsins, affecting research outcomes.
While advancements are made frequently, addressing these technological constraints is critical to enhancing the reliability and applicability of optogenetics.
Ethical Considerations
As the use of optogenetics expands, ethical considerations have gained attention. The ability to manipulate neural circuits raises questions about how this technology is used, especially in living organisms. One primary concern is animal welfare. Ensuring humane treatment of animal subjects is paramount, as the potential for harm increases with more invasive procedures. Researchers are urged to adhere to guidelines that minimize suffering while maximizing scientific knowledge.
Another key ethical dilemma surrounds consent and autonomy, particularly in human studies. If optogenetics were to be used in therapeutic settings or mental health treatments, the implications on patient autonomy and informed consent need thorough examination. The possibility of altering behaviors or experiences raises concerns about whether patients fully understand the risks involved.
Finally, the societal implications of enhancing or modifying neuronal functions need careful consideration. Issues arise when powerful tools are developed without comprehensive understanding or oversight, leading to potential misuse.
"The integration of ethical principles into optogenetic research is vital for ensuring that advancements align with societal values and human rights."
"The integration of ethical principles into optogenetic research is vital for ensuring that advancements align with societal values and human rights."
Future Directions in Optogenetics Research
The field of optogenetics continues to grow, presenting new possibilities in neuroscience. As technologies develop, the future of optogenetics looks promising, with significant implications for research and therapy. There are several critical elements to consider in this expanding frontier. Innovations can lead to more precise techniques for studying cellular activities. Enhanced methods will not only allow scientists to observe neural circuits but also to manipulate them with unprecedented accuracy. This has the potential to refine our understanding of how different brain regions communicate and contribute to behavior.
One important aspect is the potential integration of optogenetics with other advanced technologies. For instance, coupling optogenetics with machine learning could provide insights into complex biological processes. Researchers might analyze vast amounts of data more effectively. Furthermore, improvements in optical devices will likely enhance the capabilities of optogenetic tools. Miniaturized hardware and innovative optical fibers promise to deliver more versatile solutions for in vivo applications.
"Future advancements in optogenetics may unveil the intricate relationship between neuronal circuits and cognitive functions, reshaping our understanding of brain dynamics."
"Future advancements in optogenetics may unveil the intricate relationship between neuronal circuits and cognitive functions, reshaping our understanding of brain dynamics."
The implementation of optogenetic techniques beyond traditional model organisms is also noteworthy. As researchers begin to explore applications in larger animals and potentially humans, it raises both opportunities and challenges. The ability to study human-specific neural mechanisms, for example, can lead to personalized medicine approaches, tailoring therapies to individual needs specifically in cases where conventional treatments are insufficient.
Emerging Technologies
One of the exciting developments is the advancement of new optogenetic tools. Researchers are exploring the use of novel opsins with improved kinetics. For example, newer models such as Chronos and Brandom enable faster and more reliable activation of neurons. This leads to better temporal resolution in neuronal control. Additionally, non-invasive techniques such as ultrasound optogenetics are gaining traction. This method uses ultrasound waves to stimulate neurons without needing light penetration, thus increasing safety and reducing the complexity of setups.
Furthermore, combining optogenetics with CRISPR technology allows precise gene-editing capabilities. This can enhance the specificity of opsins expressed in targeted cells. Applications might range from understanding gene function to unraveling diseases at the genetic level, providing broad benefits to neuroscience.
Interdisciplinary Applications
Optogenetics is not limited to neuroscience alone; its applications span numerous fields. Collaborations with disciplines such as robotics, artificial intelligence, and even sociology are beginning to emerge. For instance, the integration of robotics allows scientists to build models that simulate neuronal activities. This modeling helps in understanding complex behaviors related to neural circuits.
Additionally, interdisciplinary approaches are making their way into treatment methodologies. Collaborative efforts could address mental health disorders by combining optogenetics with psychological frameworks. Such teamwork can lead to innovative interventions and better management of conditions such as depression and anxiety. The convergence of diverse fields like these enriches both research and practical applications, enabling a deeper exploration of human behavior and brain function.
Culmination
The conclusion section serves a crucial role in summarizing the intricate elements discussed throughout this article. It encapsulates the essence of optogenetics in neuroscience, emphasizing the intertwining of genetics with optical techniques to illuminate specific neural processes. The significance of these methodologies cannot be overstated, as they offer unprecedented insights into the workings of the brain and the underlying mechanisms of various neurological conditions.
Summary of Key Insights
In reviewing the key points, it is clear that optogenetics has revolutionized the field of neuroscience. Some central insights include:
- Precision Control: The ability to control neural activity with light provides a level of precision unattainable with traditional methods.
- Neural Circuit Mapping: New advancements allow for detailed mapping of neural circuits, facilitating a deeper understanding of their functions.
- Behavioral Research: Optogenetics has opened up avenues in behavioral studies, allowing researchers to observe the effects of targeting specific neurons in live subjects.
- Therapeutic Applications: This technique holds promise for developing innovative treatments for neurological disorders, such as depression and Parkinson's disease.
All these points highlight a transformative approach to studying and treating brain systems.
Implications for Neuroscience
The implications of optogenetics extend beyond mere research applications. They are multifaceted and touch on numerous aspects of neuroscience:
- Understanding Complex Processes: By enabling targeted manipulation of neural populations, optogenetics paves the way for elucidating complex neuronal processes, such as memory, learning, and emotion regulation.
- Revolutionizing Treatments: The therapeutic potential may lead to novel treatments for various diseases. The integration of optogenetics in clinical settings may improve patient outcomes significantly.
- Interdisciplinary Collaborations: Optogenetics drives collaborative efforts among biologists, engineers, and clinicians. Such interdisciplinary approaches can yield innovative solutions to existing challenges in neuroscience.
- Ethical Considerations: With these advancements come ethical discussions. The implications of manipulating brain activity raise questions that must be thoughtfully addressed as the field evolves.
"Optogenetics provides unprecedented opportunities to dissect the complexities of brain functions and diseases, potentially shaping a new era in neuroscience."
"Optogenetics provides unprecedented opportunities to dissect the complexities of brain functions and diseases, potentially shaping a new era in neuroscience."
In summary, the field of optogenetics not only enhances our understanding of neural mechanisms but also holds great promise for future innovations in neuroscience. As researchers continue to explore its potential, the future holds exciting developments that may reshape how we perceive and interact with the brain.