Comprehensive Overview of DNA Targeting Techniques
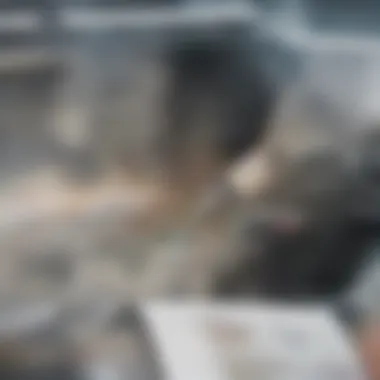
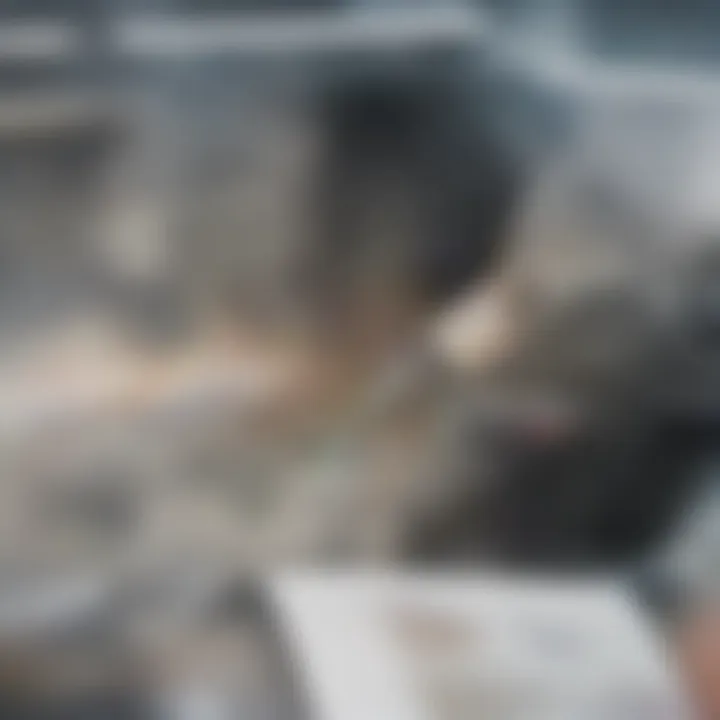
Article Overview
Purpose of the Article
This article aims to provide a thorough understanding of DNA targeting, a vital process in molecular biology. It will focus on the methods used to target specific DNA sequences and the applications in gene editing and therapeutic settings. By exploring current challenges and ethical considerations, this article presents a well-rounded view of the significance of DNA targeting in modern science.
Relevance to Multiple Disciplines
DNA targeting has wide implications across various fields such as genetics, medicine, and biotechnology. Whether it is in developing gene therapies, enhancing agricultural traits, or studying genetic disorders, the principles of DNA targeting remain fundamental. As such, the insights presented here will be useful for students, researchers, educators, and professionals alike.
Research Background
Historical Context
The exploration of DNA began in the early 20th century, culminating in the discovery of the double helix structure by James Watson and Francis Crick in 1953. Since then, advancements in molecular biology have led to significant developments in DNA manipulation techniques, paving the way for targeted interventions. Early methods like restriction enzymes have evolved, leading to modern approaches such as CRISPR.
Key Concepts and Definitions
DNA targeting encompasses processes designed to bind and modify specific DNA sequences. This includes techniques for gene editing like CRISPR-Cas9, which utilizes guide RNA to locate target sequences. Other methods include zinc-finger nucleases and transcription activator-like effector nucleases (TALENs). Understanding these concepts is crucial for grasping the complexities of molecular biology today.
"The ability to target specific genes opens new doors for therapeutic applications and genetic research."
"The ability to target specific genes opens new doors for therapeutic applications and genetic research."
In summary, the integration of theoretical principles with practical applications will help readers navigate the landscape of DNA targeting effectively.
Prelims to DNA Targeting
DNA targeting encompasses a range of techniques and methodologies aimed at specifically interacting with DNA sequences. This area of molecular biology is critical for purposes such as gene editing, diagnostics, and therapeutic interventions. Understanding the principles and applications of DNA targeting allows researchers to explore genetic modifications and understand fundamental biological processes more deeply.
The significance of DNA targeting extends beyond research; it has implications in medicine, agriculture, and biotechnology. For example, targeted gene editing can lead to breakthroughs in treating genetic disorders. Additionally, in agriculture, modifying specific genes can enhance crop resilience and nutritional value. Thus, DNA targeting not only opens doors for scientific inquiry but also has real-world applications that can benefit society at large.
Defining DNA Targeting
DNA targeting refers to the ability to identify and bind to specific DNA sequences to induce a desired biological effect. This can encompass a variety of methodologies, such as inserting, deleting, or modifying DNA within an organism’s genome. The precision of DNA targeting is vital for minimizing unintended consequences, such as off-target effects, which can lead to undesirable mutations.
- Key elements of DNA targeting include:
- Specificity to targeted sequences
- Predictable outcomes for genetic modifications
- Potential applications in multiple fields
The ability to manipulate DNA with precision is a cornerstone of modern genetic research.
Historical Context and Development
The journey of DNA targeting began with foundational discoveries in molecular biology, notably the structure of DNA and its role in heredity. The most significant development in DNA targeting came with the advent of gene editing technologies. Early methods included restriction enzymes, which allowed scientists to cut DNA at specific sites. However, these approaches had limitations in terms of specificity and control.
In the early 21st century, revolutionary techniques such as CRISPR-Cas9 emerged, vastly improving the precision and efficiency of DNA targeting. The simplicity and flexibility of CRISPR has made it a widely adopted tool in research laboratories around the world.
"The development of CRISPR-Cas9 represents a transformative shift in genetic engineering, making precise DNA manipulation accessible and efficient."
"The development of CRISPR-Cas9 represents a transformative shift in genetic engineering, making precise DNA manipulation accessible and efficient."
As the field continues to evolve, techniques are becoming more refined, allowing for diverse applications in genetic research and medicine. Understanding this historical context provides critical insight into the current landscape of DNA targeting technologies.
Mechanisms of DNA Targeting
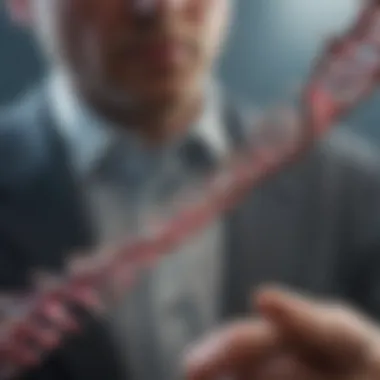
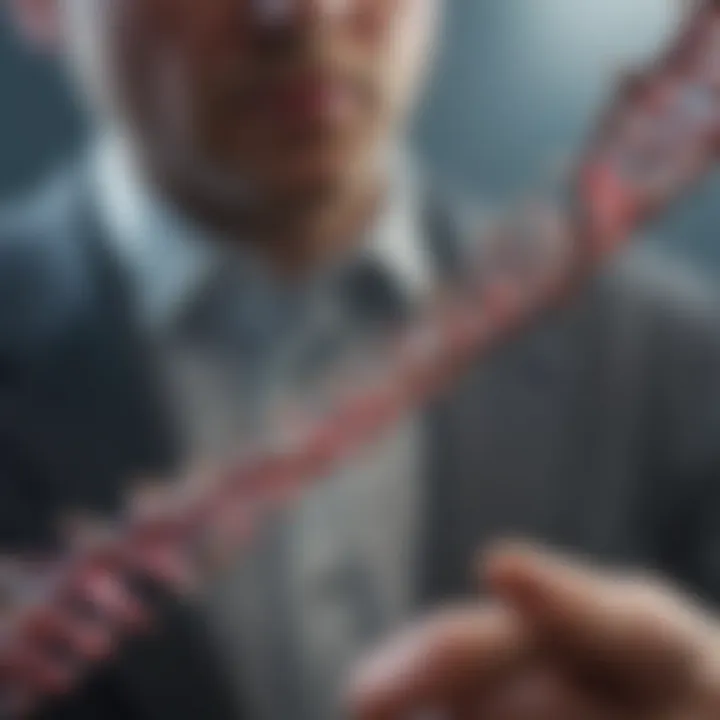
Understanding the mechanisms of DNA targeting is crucial for grasping how specific DNA sequences can be manipulated for various applications. These mechanisms can be broadly categorized into two types: natural and synthetic. Each type offers unique advantages and challenges, influencing how researchers approach gene editing and therapy.
Natural DNA Targeting
Natural DNA targeting refers to the processes evolved in various organisms that allow specific interaction with DNA. This type primarily includes systems like restriction endonucleases and naturally occurring RNA-guided nucleases like CRISPR-Cas. These mechanisms have been honed through millions of years of evolution and offer several benefits:
- Precision: Natural systems often exhibit high specificity for their target sites, reducing the likelihood of unwanted modifications.
- Efficacy: Many natural systems are efficient at identifying and cleaving DNA, which can be advantageous for applications such as genome editing or the development of gene therapies.
- Versatility: Natural mechanisms can be adapted for various applications, from basic research to therapeutic interventions.
Natural targeting employs proteins or enzymatic functions that facilitate the recognition of specific DNA sequences. For example, the CRISPR-Cas9 system utilizes a guide RNA to direct the Cas9 nuclease to its specific genomic target. This genetic 'memory' allows the system to target invasive sequences with exceptional accuracy, representing a giant leap forward in genetic engineering.
Synthetic DNA Targeting Strategies
Synthetic DNA targeting strategies encompass artificially designed tools that enable precise modifications to the genome. These strategies have emerged from an in-depth understanding of natural systems and provide additional flexibility. Among the most prominent synthetic strategies are Zinc Finger Nucleases (ZFNs) and Transcription Activator-Like Effector Nucleases (TALENs).
- Zinc Finger Nucleases: ZFNs combine a zinc finger DNA-binding domain with a nuclease, allowing for targeted double-strand breaks at any desired location within the genome. They have become instrumental in creating knockout models and inserting genes with high precision.
- TALENs: TALENs are similar to ZFNs but offer more straightforward design rules for creating specific DNA-binding domains. They have gained popularity due to their ease of use and effectiveness, especially in organisms where delivery of other targeting systems may be more complicated.
- CRISPR-Cas Variants: While originally natural, CRISPR technology has seen countless adaptations, with numerous variants created for specific targeting purposes. Modifications to the Cas9 enzyme, guide RNA sequence design, and PAM recognition have expanded the repertoire of targeting possibilities.
Synthetic targeting systems, such as ZFNs and TALENs, allow customized approaches to gene editing, offering unique advantages in experimental flexibility and specificity.
Synthetic targeting systems, such as ZFNs and TALENs, allow customized approaches to gene editing, offering unique advantages in experimental flexibility and specificity.
Techniques for DNA Targeting
DNA targeting plays a crucial role in molecular biology, enabling researchers to alter genetic sequences with precision. Various techniques have emerged in recent years, each with specific advantages and limitations. This section delves into prominent methods that researchers utilize in DNA targeting, highlighting the underlying principles, applications, and practical considerations.
CRISPR-Cas Systems
CRISPR-Cas technology has revolutionized the field of genetic engineering. It is based on a natural defense mechanism found in bacteria that use CRISPR arrays to protect against viral infections. The main components of CRISPR-Cas systems include a guide RNA, which directs the Cas enzyme to the target DNA sequence, and the Cas enzyme itself, which cleaves the DNA, allowing for modifications.
The precision and efficiency of CRISPR make it a preferred choice for many genetic editing applications. Researchers can easily design guide RNAs for specific targets, making the process adaptable across various species. Moreover, CRISPR systems can be programmed not only for editing but also for transcriptional regulation and epigenetic modifications. This versatility opens avenues for complex genetic studies and therapeutic interventions.
However, ethical and technical challenges remain, particularly concerning off-target effects. Ensuring the accuracy of CRISPR modifications is essential for reliable outcomes in potential therapies.
Zinc Finger Nucleases
Zinc finger nucleases (ZFNs) represent another innovative approach to DNA targeting. ZFNs are engineered proteins that can create double-strand breaks at targeted sites in the genome. Each zinc finger domain can sense a specific base pair in DNA, allowing ZFNs to be tailored for precise targeting. This feature makes ZFNs particularly useful for gene knockout, replacement, or insertion.
One of the main advantages of ZFNs is the ability to develop highly specific tools that can be adapted for various organisms. Despite this specificity, the design and assembly of ZFNs can be resource-intensive and time-consuming. Additionally, ZFN technology has faced criticism related to potential off-target activity, similar to that of other gene-editing technologies. Nonetheless, when used judiciously, ZFNs have facilitated significant advancements in gene therapy and functional genomics.
TALENs (Transcription Activator-Like Effector Nucleases)
TALENs are another category of engineered nucleases for precise DNA targeting. They function as fusion proteins composed of a transcription activator-like effector domain and a DNA cleavage domain. By modifying the effector domain, researchers can develop TALENs to bind specific DNA sequences and induce double-strand breaks.
Like ZFNs, TALENs provide a flexible framework for genome editing. They have been utilized in various applications, such as generating knockout models and inserting specific genes for research or therapeutic purposes. Their modularity allows for easier design and construction compared to ZFNs.
Despite their advantages, TALENs are not without their limitations. Issues related to delivery efficiency and off-target effects persist. Careful consideration of these factors is crucial in ensuring the effectiveness and safety of TALEN-mediated DNA targeting.
In summary, understanding the different techniques for DNA targeting is essential for advancing molecular biology and genetic engineering. Each method provides unique benefits and considerations, emphasizing the importance of selecting the appropriate tool based on specific research goals and applications.
In summary, understanding the different techniques for DNA targeting is essential for advancing molecular biology and genetic engineering. Each method provides unique benefits and considerations, emphasizing the importance of selecting the appropriate tool based on specific research goals and applications.
Applications of DNA Targeting
DNA targeting has become a pivotal focus in multiple scientific disciplines. This section delves into its various applications, highlighting the importance of understanding how DNA manipulation can affect ecosystems, health, and technological advancement. Each application not only demonstrates the versatility of DNA targeting techniques but also opens up avenues for further inquiry and innovation.
Gene Editing and Modification
Gene editing represents one of the most transformative applications of DNA targeting. Techniques such as CRISPR-Cas9 allow precise modifications to an organism's genome. This precision facilitates the removal of faulty genes and the introduction of beneficial ones. In research, scientists can manipulate genetic sequences to investigate the functions of specific genes, paving the way for breakthroughs in understanding disease mechanisms.
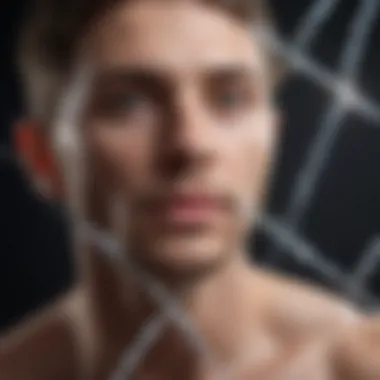
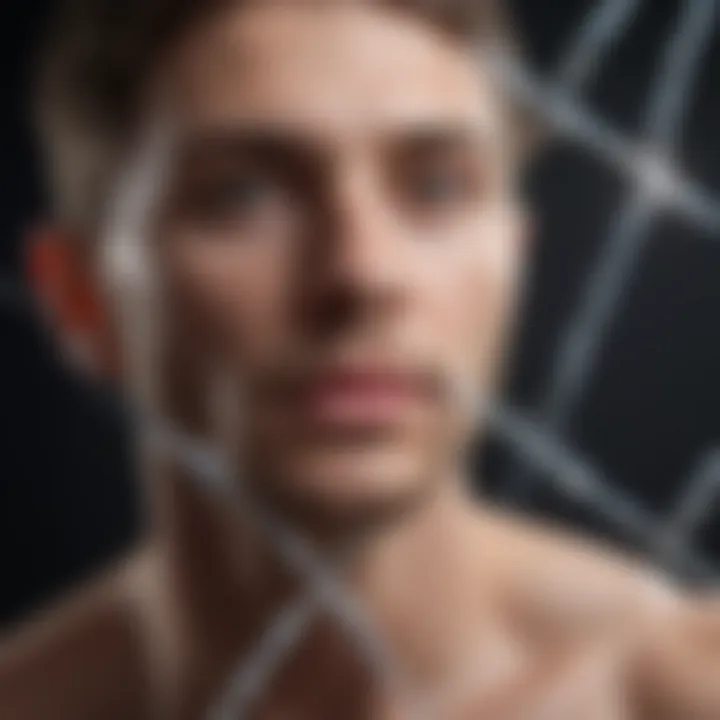
Additionally, gene modification holds potential in therapeutic contexts. For instance, the targeted alteration of genes responsible for hereditary diseases could lead to revolutionary treatments. However, ethical considerations surrounding such modifications are significant, especially in human germline editing, raising debates that echo throughout the scientific community.
Research has already highlighted several successful applications of gene editing in therapeutic scenarios, including sickle cell disease and cystic fibrosis treatments, showcasing its potential for changing lives.
DNA Targeting in Medicine
In medicine, DNA targeting serves as a critical tool for developing targeted therapies. By identifying and altering precise DNA sequences in cancer cells, treatments can become more effective and less toxic compared to traditional chemotherapy. This approach not only enhances treatment efficacy but also minimizes damage to healthy cells.
"Targeted therapies arise from DNA targeting, aiming for precision in treatment that classical methods might lack."
"Targeted therapies arise from DNA targeting, aiming for precision in treatment that classical methods might lack."
One notable example is the use of CAR-T cell therapy, which involves modifying a patient's T cells to better recognize and combat cancer. These targeted treatments mark a significant shift in how diseases are approached, focusing on the unique genetic makeup of individual patients. Such personalized medicine indicates a future where treatments are tailored, improving outcomes while reducing adverse effects.
Applications in Agriculture
DNA targeting also finds application in agriculture, where it revolutionizes crop improvement strategies. Through gene editing, scientists can create crops that are resistant to pests and diseases, thereby enhancing yield and sustainability. For instance, the development of drought-resistant maize through precise gene modifications is a notable achievement.
Moreover, DNA targeting facilitates advancements in nutritional quality. By altering specific genes in staple crops, researchers can enhance the nutritional profile of these foods; for example, biofortified rice produced with increased levels of vitamin A. Such enhancements not only combat malnutrition but also expand the scope of food security in vulnerable regions.
However, the integration of these technologies into agriculture must consider ecological implications and regulatory environments. The balance between innovation and environmental responsibility is crucial.
Synthetic Biology and Biotechnology
Synthetic biology leverages DNA targeting to engineer organisms for various purposes, including biofuels, pharmaceuticals, and industrial enzymes. By rewriting genetic codes, scientists design microorganisms that can produce complex compounds efficiently. This field exemplifies how DNA targeting drives innovation in biotechnology.
For example, genetically modified bacteria have been developed to produce insulin, which is essential for diabetes management. Similarly, synthetic yeast strains have been designed to produce precursors for biofuels, showcasing how DNA targeting contributes to solving energy crises.
The future landscape of biotechnology will likely be shaped by advancements in DNA targeting, leading to more sustainable practices and innovative solutions to global challenges. As research progresses, the potential applications across diverse fields will continue to expand, underscoring the relevance of this technique in modern science.
Challenges in DNA Targeting
The field of DNA targeting is not without its complexities and challenges. Understanding these challenges is crucial for the advancement of research and practical applications. Moreover, these challenges can impact the efficacy and safety of DNA manipulation methods. As we navigate through these issues, it is important to consider their implications for not only scientific breakthroughs but also ethical dilemmas.
Off-Target Effects
Off-target effects are one of the most significant concerns in DNA targeting. These refer to unintended modifications that occur in regions of the genome that are not the intended targets. The implications of off-target effects can be quite serious, including unwanted gene modifications that may lead to adverse side effects or even the development of diseases. For example, in gene therapy, off-target modifications can result in oncogenesis or immune reactions against modified cells. Researchers are continually working on methods to minimize these errors, utilizing improved specificity in tools like CRISPR and other nuclease systems. Improved bioinformatics tools can also help in predicting and assessing potential off-target sites during experimental designs.
"Reducing off-target effects is not just about precision; it's about ensuring the safety of genetic therapies that could change lives."
"Reducing off-target effects is not just about precision; it's about ensuring the safety of genetic therapies that could change lives."
Delivery Mechanisms
The delivery of DNA targeting systems into cells is another critical challenge. The efficiency of delivery methods can greatly influence the success of DNA targeting. Various techniques are employed, including viral vectors, liposomes, and electroporation. However, these methods come with their own set of limitations. For instance, viral vectors may elicit immune responses while liposomes may face difficulty penetrating cellular membranes. Electroporation can be effective, but it also risks damaging the target cells.
Continued innovation in delivery systems is essential. New strategies, such as nanoparticles and biomolecular delivery systems, promise improved functionality. These methods need to ensure not only efficiency in delivery but also minimize cytotoxicity. The challenge lies in developing a balance between efficacy and safety in these methods of delivery.
Regulatory Hurdles
Finally, regulatory hurdles present a significant barrier in the field of DNA targeting. As techniques advance, many countries are grappling with how to effectively regulate gene editing and DNA manipulation. Regulations vary widely, and inconsistent enforcement can hinder research and application. The uncertainty can create reluctance among investors and researchers when considering new projects or transformations within existing frameworks. Establishing clear guidelines that reflect the rapid pace of scientific advancement is necessary.
Furthermore, public perception plays a role in shaping regulations. Misinformation or exaggerated narratives around genetic editing can sway public opinion, leading to stricter laws or public pushback. Scientists, regulators, and the community must engage in transparent dialogue to build trust and understanding of the scientific goals and ethical considerations involved in DNA targeting.
Ethical Considerations
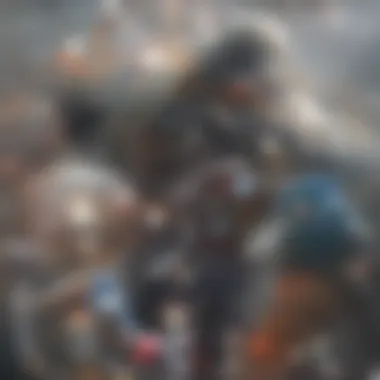
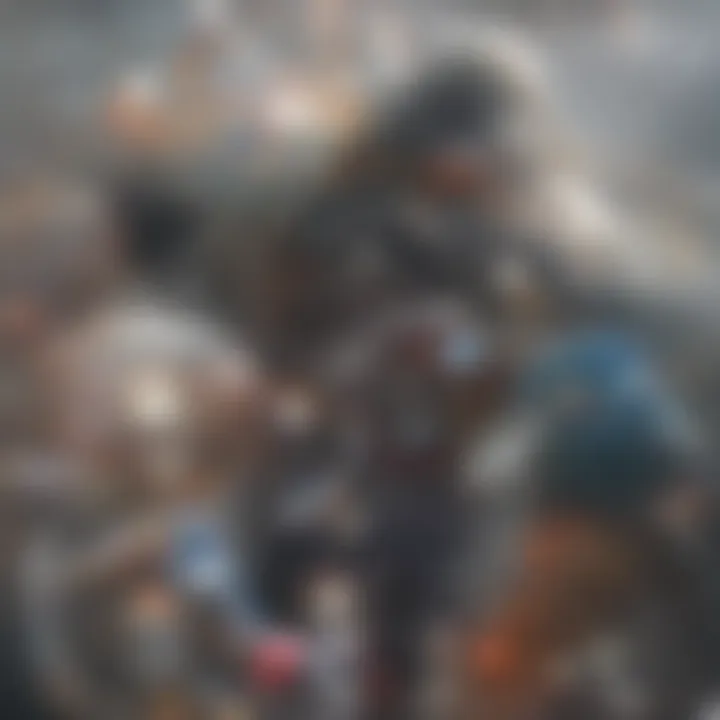
In the rapidly evolving landscape of molecular biology, particularly within the realm of DNA targeting, ethical considerations are of paramount importance. As techniques continue to advance, the implications of these technologies extend beyond mere scientific inquiry. They delve deep into the moral fabric of society, affecting regulatory frameworks, public sentiment, and the very essence of life itself. Understanding these ethical considerations can help inform both scientists and the public about the responsibilities that accompany such powerful tools.
Ethics of Gene Editing
Gene editing techniques, especially CRISPR-Cas9, have opened pathways in medical research, agriculture, and conservation efforts, but ethical dilemmas abound. One major concern lies in the potential for unintended consequences. Even with precise tools, risks of off-target effects can lead to unpredicted mutations, raising questions about safety and long-term impacts on ecosystems and human health.
Additionally, the possibility of designer babies introduces complex ethical questions about equity and access. Would this technology create disparities where only affluent families can afford genetic enhancements? Furthermore, the question of consent arises. When editing genes within embryos, future generations have no say in the matter. These considerations necessitate thorough ethical review processes and public dialogue to ensure that gene editing is employed judiciously.
Public Perceptions and Misconceptions
Public perception of DNA targeting and gene editing is highly variable. Misinformation can skew understanding and spark fear. People may equate gene editing with playing God or causing genetic abnormalities without recognizing the scientific rigor behind these techniques. Misconceptions can also lead to regulatory backlash, hindering innovation.
To bridge the gap, it is crucial to engage in transparent communication about the science behind these technologies. Educational initiatives can help demystify the processes involved, emphasizing that while gene editing holds profound promise, it is equally accompanied by responsibilities. Efforts should focus on clarifying the difference between therapeutic applications and enhancements that may breach ethical boundaries. Moreover, ensuring that ethical frameworks evolve alongside scientific advancements is essential for fostering public trust.
"As we navigate the complexities of DNA targeting, it is imperative to reflect on the societal implications of our scientific endeavors."
"As we navigate the complexities of DNA targeting, it is imperative to reflect on the societal implications of our scientific endeavors."
In summary, ethical considerations regarding DNA targeting are crucial for its acceptance and safe application. By addressing these issues head-on, we can harness the potential of these technologies while safeguarding against their risks. This balance is critical for fostering an informed society that values both scientific progress and ethical responsibility.
Future Perspectives in DNA Targeting
The realm of DNA targeting is rapidly evolving. Understanding the future prospects is crucial for several reasons. It allows researchers, educators, and practitioners to pave pathways for innovative solutions powered by advanced techniques. The advancements in DNA modulation not only enhance genetic research but also improve agricultural practices and therapeutic strategies.
Advancements in technology will play a pivotal role in shaping these future perspectives. With tools and methods becoming more precise and efficient, the potential to manipulate genetic material grows exponentially. This can lead to breakthroughs that fundamentally change our understanding of genetics.
Advancements in Technology
Emerging technologies are continuously redefining DNA targeting. Among them, the CRISPR-Cas systems stand out for their user-friendly nature and adaptability. Unlike its predecessors, CRISPR allows for multi-target edits with minimal off-target effects. This ensures more specific gene modifications, reducing unintended consequences in research and therapy.
Other promising advances include synthetic biology approaches. Techniques are rapidly being developed to enhance the predictability of DNA interactions. For instance, the use of machine learning algorithms helps scientists predict the effectiveness of targeting sequences, thus streamlining the process.
Moreover, innovative delivery methods, such as nanoparticles, are being explored. These carriers can package DNA editing tools, ensuring that they reach their specific targets within cells effectively. This improvement addresses one of the main challenges previously faced in the field.
Potential New Applications
The potential applications of DNA targeting are vast and varied, extending beyond immediate scientific interests to real-world implications. One significant area is in medicine, particularly personalized therapy. With advancements in DNA targeting, researchers have the capability to treat genetic disorders by correcting faulty genes at the source.
In agriculture, new genetically modified organisms can lead to crops that withstand environmental stresses better. Targeting can allow for traits such as pest resistance or enhanced nutritional value, benefiting food security and sustainability.
Furthermore, the integration of DNA targeting with synthetic biology could lead to the creation of entirely new organisms. This can have applications in biofuels, pharmaceuticals, and even biosensing technologies, expanding the horizon of biotechnology.
"Innovative developments in DNA targeting techniques have the potential to revolutionize not just genetic research, but healthcare and environmental sustainability as well."
"Innovative developments in DNA targeting techniques have the potential to revolutionize not just genetic research, but healthcare and environmental sustainability as well."
Epilogue
In the realm of molecular biology, the exploration of DNA targeting stands as a pivotal focus. This article has delved into numerous facets of DNA targeting, shedding light on its significance in both theoretical and practical contexts. As science progresses, understanding DNA targeting becomes essential not only for researchers but also for a broader audience invested in genetics and biotechnological advancements.
Summary of Key Insights
Throughout the article, several key insights emerged regarding DNA targeting. First, the techniques employed, such as CRISPR-Cas systems and TALENs, have revolutionized gene editing. These methodologies enhance precision in targeting specific sequences of DNA, leading to groundbreaking developments in genetic engineering.
Second, the applications of DNA targeting are far-reaching. From medical interventions, where it enables gene therapy for genetic disorders, to agricultural innovations that promote crop resilience, the profound impact is evident. Additionally, synthetic biology leverages these techniques for creating novel organisms designed for various purposes.
Furthermore, the challenges faced in DNA targeting highlight the need for continued vigilance. Off-target effects pose a significant risk, necessitating advancements in delivery mechanisms to enhance accuracy. Regulatory hurdles also impede progress, making it imperative to establish clear guidelines that align ethics with scientific exploration.
Implications for Future Research
The future of DNA targeting appears promising, with numerous implications for research. Identifying and refining techniques that reduce off-target effects can lead to safer and more effective applications in medicine and agriculture. Moreover, integrating artificial intelligence in DNA design and targeting can accelerate discovery and innovation.
As new technologies emerge, exploring potential new applications will be key. Field trials in agriculture could reshape food security, while personalized medicine could progress significantly with tailored gene therapies.