Unraveling the Role of Packaging Vectors in Science
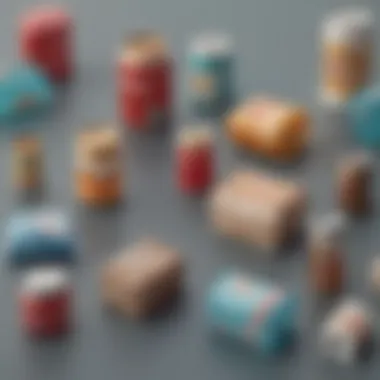
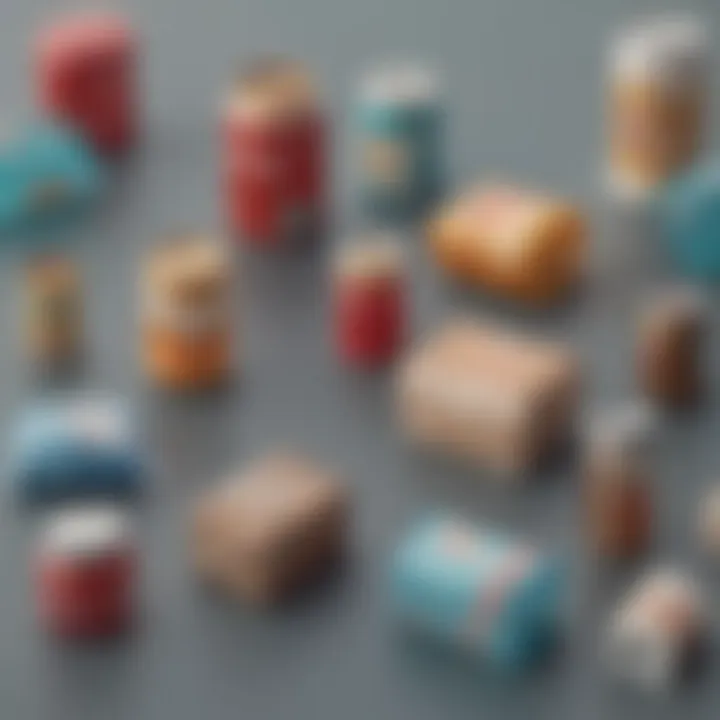
Article Overview
Purpose of the Article
The purpose of this article is to provide an in-depth analysis of packaging vectors and their essential role in scientific research, particularly in molecular biology and genetics. As these fields evolve, it becomes increasingly important to understand the mechanisms behind packaging vectors and their application in gene delivery systems. This article aims to elucidate the types of packaging vectors available, their operational mechanisms, and the recent innovations that drive their effectiveness. By doing so, this comprehensive exploration will serve as a valuable resource for students, researchers, educators, and professionals in related disciplines.
Relevance to Multiple Disciplines
Packaging vectors are not limited to molecular biology; they intersect various fields of research. The implications span areas like synthetic biology, gene therapy, and biomedical engineering. Their ability to facilitate gene delivery makes them crucial for developing novel therapeutics and diagnostics. Understanding the dynamics of packaging vectors thus holds relevance for a wide spectrum of disciplines, making this article particularly significant for those working at the interface of biology and technology.
Research Background
Historical Context
The concept of packaging vectors has developed significantly over the last few decades. Initially, research focused on other vectors like plasmids for simple gene transfer. However, challenges arose with efficiency and safety, necessitating more sophisticated alternatives. The advent of viral vectors marked a turning point, opening new avenues for efficient gene delivery. Today, the evolution of packaging vectors continues with ongoing innovations that broaden their applications across scientific fields.
Key Concepts and Definitions
To engage with the topic effectively, several key concepts must be clearly defined:
- Packaging Vectors: These are tools designed to encapsulate genetic material and deliver it into host cells. They can be viral or non-viral, each type having distinct advantages and limitations.
- Gene Delivery: The process through which genetic material is introduced into cells to enable expression of specific genes.
- Molecular Biology: A branch of biology that focuses on the structure and function of the molecules essential to life. It studies how genes are expressed and regulated.
Understanding these key terms lays the groundwork for appreciating the complexity and significance of packaging vectors in modern scientific research.
"The advancement of packaging vectors is a testament to the collaborative nature of modern science, where multiple disciplines converge to solve complex biological challenges."
"The advancement of packaging vectors is a testament to the collaborative nature of modern science, where multiple disciplines converge to solve complex biological challenges."
In exploring packaging vectors, this article will address their role in synthesizing innovative therapies and enhancing methodologies in research while also considering safety and ethical implications. Through an analytical lens, the information presented aims to provide comprehensive insights into a rapidly advancing area of scientific inquiry.
Foreword to Packaging Vectors
The significance of packaging vectors in molecular biology and genetics cannot be overstated. These vectors serve as critical tools for the delivery of genetic material into host cells, which is fundamental for various research and therapeutic applications. Understanding their function and utility is paramount as the field of gene therapy advances rapidly. This section provides a detailed overview of packaging vectors, examining their definition, importance, and historical context which shapes current practices.
Definition and Importance
Packaging vectors are biological carriers designed to transport genetic material into cells. This genetic material can include DNA or RNA, which is essential for gene expression. The precise management of these vectors ensures that the genetic information is delivered effectively and securely, minimizing risks and maximizing potential benefits. The significance of these vectors lies in several key factors:
- Gene Delivery: They facilitate the transfer of complex genes that can be challenging to introduce using other methods.
- Therapeutic Applications: Packaging vectors are vital in developing treatments for genetic disorders and cancers, enabling advancements in gene therapy.
- Research Applications: In basic research, they allow scientists to manipulate genetic materials to understand gene functions and interactions better.
In summary, the role of packaging vectors is essential in both therapeutic contexts and fundamental research, making their study a top priority for those working in genetics.
Historical Context
The journey of packaging vectors began decades ago, at a time when molecular biology was emerging as a distinct scientific field. The discovery of the structure of DNA by James Watson and Francis Crick in 1953 laid the groundwork for understanding genetic material. However, it wasn't until the 1970s and 1980s that the first successful applications of vectors were reported. Early vectors primarily included plasmid vectors, which paved the way for more sophisticated systems over time.
As research progressed, scientists started employing viral vectors, exploiting their natural ability to transfer genes into host cells. The introduction of lentiviral and adenoviral vectors represented a significant milestone. They provided efficient methods for gene transfer, leading to breakthroughs in gene therapy.
Today, the field continues to evolve with emerging technologies such as CRISPR-based systems and innovative non-viral delivery methods. Understanding this historical context helps to appreciate the advancements in vector design and their implications for future research and therapeutic strategies.
Types of Packaging Vectors
The understanding of packaging vectors is crucial in modern biological research and therapeutic applications. These vectors serve as vehicles to deliver genetic material into target cells, influencing gene expression and enabling various experiments in genetics and molecular biology. Identifying the right type of packaging vector can enhance the efficiency of gene delivery, making them a focal point in gene therapy and related studies. This section explores the different types of packaging vectors, categorizing them into viral and non-viral vectors, each with unique features and applications.
Viral Vectors
Viral vectors are among the most widely used tools for gene delivery due to their natural ability to infect host cells. These vectors are derived from viruses but are modified to ensure safety and to enhance their utility in research. They can efficiently introduce genetic material into cells and facilitate strong and stable expression of genes. This efficiency makes them a popular choice in the field of gene therapy.
Lentiviral Vectors
Lentiviral vectors are especially notable for their ability to deliver genes into both dividing and non-dividing cells. The most distinctive characteristic of lentiviral vectors is their capability to integrate into the host genome, ensuring persistent gene expression. This feature is beneficial for long-term therapies, such as in the treatment of chronic diseases. However, potential insertional mutagenesis poses a risk, requiring careful consideration in therapeutic applications.
Adenoviral Vectors
Adenoviral vectors are derived from adenoviruses and have unique properties that facilitate robust gene delivery. One principal characteristic is their high transduction efficiency in various cell types. They are particularly notable for not integrating into the host genome, which can be advantageous when short-term expression is desired. However, their inability to achieve stable genomic integration may limit their use in long-term therapies.
Adeno-Associated Virus Vectors
Adeno-associated virus (AAV) vectors are popular for their safety and ability to induce a long-lasting gene expression. They are characterized by their unique ability to maintain a low immunogenic profile, making them suitable for repeated administration. AAV vectors can integrate into the host genome in a site-specific manner, providing stability. Nonetheless, their smaller carrying capacity for genes limits their application in delivering larger genetic constructs.
Non-Viral Vectors
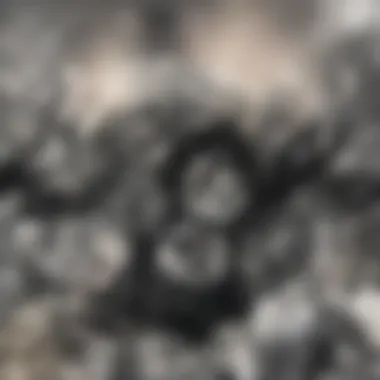
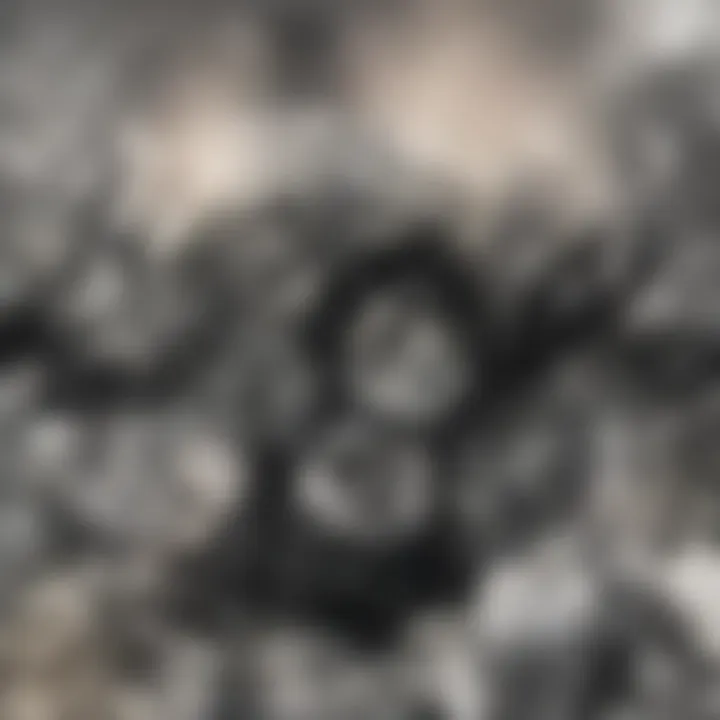
In contrast to viral vectors, non-viral vectors provide alternative methods for gene delivery that often circumvent issues related to immunogenicity and safety. They are becoming increasingly relevant due to advancements in materials science and biochemistry. Non-viral delivery methods range from lipid-based systems to polymeric approaches, presenting diverse options for researchers.
Liposomes
Liposomes are spherical vesicles composed of lipid bilayers and are used to encapsulate genetic materials. Their key characteristic lies in their ability to merge with lipid membranes of target cells, promoting the release of genetic content inside. This feature encourages a gentler delivery method with reduced cellular toxicity, making them a favorable option for therapeutic applications.
Polymeric Vectors
Polymeric vectors utilize synthetic or natural polymers to deliver genes. One strong advantage is their tunable properties; researchers can modify these vectors to control release profiles and improve targeting efficiency. However, potential cytotoxicity and challenges in large-scale production can be concerns in their widespread application in clinical settings.
Physical Methods
Physical methods include techniques such as electroporation, microinjection, and gene gun technology. These methods have the advantage of being straightforward and allowing for direct delivery of genetic material into cells. The main characteristic is their non-dependency on biological vectors, providing a way to deliver large plasmids. However, uniform delivery can be a challenge, leading to inconsistent results.
The choice between viral and non-viral vectors ultimately depends on the specific requirements of research or therapeutic goals, considering factors like efficiency, safety, and stability.
The choice between viral and non-viral vectors ultimately depends on the specific requirements of research or therapeutic goals, considering factors like efficiency, safety, and stability.
Mechanisms of Action
Understanding the mechanisms of action of packaging vectors is crucial in the realm of gene delivery and therapeutic developments. These mechanisms define how effectively the vector can introduce genetic material into host cells, ensuring that the desired treatment or research outcome is achieved. The significance of these processes lies in their direct correlation with the efficiency of gene expression, the safety of the methods involved, and the longevity of the delivered genetic material. Evaluating these mechanisms also allows researchers to refine and develop better vectors that are responsive to specific therapeutic needs while minimizing potential risks associated with gene therapy.
Insertion into Host Cells
The insertion of packaging vectors into host cells is a fundamental step in the gene delivery process. The efficiency of this insertion directly impacts the success of any subsequent gene expression. Different packaging vectors utilize varied methods to penetrate cell membranes. For example, viral vectors integrate their genetic materials by attaching to specific receptors on the host cell surface. This specificity can enhance the accuracy of gene delivery, targeting particular cell types that may be affected by a genetic disorder.
Non-viral methods, such as liposomes or polymeric vectors, generally rely on passive or active mechanisms to facilitate entry into cells. Liposomes, which are versatile carriers formed of lipid bilayers, can encapsulate genetic material and fuse with cell membranes, releasing their payload directly into the cytoplasm. Techniques such as microinjection or electroporation can also provide direct entry for these vectors but often require specialized equipment and may induce cellular stress.
Ultimately, the choice of vector impacts the insertion effectiveness. Factors like vector size, charge, and targeting capabilities play critical roles in determining how efficiently the genetic material is introduced into the host cells.
"The process of insertion into host cells determines the foundational success of gene therapy applications."
"The process of insertion into host cells determines the foundational success of gene therapy applications."
Gene Expression Regulation
Once the packaging vector has successfully inserted its genetic content into the host cell, the next challenge is to regulate gene expression. This involves ensuring that the introduced genes are not only integrated into the cellular machinery but also actively expressed at appropriate levels. Gene expression regulation is crucial for therapeutic efficacy and avoiding unwanted effects such as overexpression or toxicity.
Several factors influence gene expression after delivery. The vector design, including promoter choice and regulatory elements, must be tailored to achieve desired activity levels in specific contexts. For example, constitutive promoters may drive continuous expression, while inducible systems allow for conditions that can control activity in response to external stimuli.
Furthermore, the stability of the delivered genetic material affects how long these genes will remain active. Epigenetic modifications and chromatin structure can also modulate expression levels, affecting the therapeutic outcome. Understanding these interactions and controls can lead to more effective gene therapies.
In summary, unraveling the mechanisms of action demonstrates their significance in developing and refining packaging vectors for gene therapy and research applications. By improving insertion methods and regulating gene expression, researchers can enhance the overall effectiveness and safety of gene can deliver their required therapeutic outcomes.
Applications in Gene Therapy
Gene therapy represents a transformative approach in treating various diseases, particularly genetic disorders and cancers. The utilization of packaging vectors is crucial in this process, as they serve as vehicles that facilitate the delivery of therapeutic genes into target cells. This section emphasizes the significance of applications in gene therapy, discussing beneficial elements and considerations that are vital for successful interventions.
Treatment of Genetic Disorders
Genetic disorders arise from mutations in specific genes, leading to improper functioning of biological processes. Gene therapy offers hope by introducing modified genes to rectify these mutations. Packaging vectors play a key role in ensuring that therapeutic genes effectively reach their destination—often cells that are affected by the disorder.
The benefits of using packaging vectors in treating genetic disorders include:
- Precision: They allow for targeted delivery, minimizing off-target effects that can lead to unintended consequences.
- Sustainability: Some vectors can provide long-term expression of the therapeutic gene, offering ongoing benefits rather than a single treatment.
- Versatility: Different types of vectors can be designed to adapt to various needs, accommodating a broad range of genetic disorders.
However, challenges exist. The immune response can limit the effectiveness of the treatment. Careful selection and design of packaging vectors help mitigate these immune reactions, enhancing the overall efficiency of gene therapy. Regulatory considerations also play a significant role; all treatments must comply with strict guidelines to ensure patient safety and efficacy.
Oncology Applications
Cancer therapy has also benefited from advancements in gene therapy, with packaging vectors facilitating targeted anti-cancer strategies. These vectors can deliver genes that induce apoptosis in cancer cells or modify immune responses, promoting a better attack on tumors.
Key aspects of oncology applications include:
- Targeting Specific Tumors: Utilizing vectors designed for specific cancer types increases the probability of therapeutic success and reduces side effects related to traditional treatments.
- Enhanced Immune Modulation: Some vectors can be engineered to enhance the immune system’s ability to recognize and destroy cancer cells, working alongside other treatments like immunotherapy.
- Combination Therapies: Gene therapy can be paired with conventional treatments like chemotherapy and radiation, presenting a multi-faceted approach to combat cancer.
While the promise is considerable, potential challenges in oncology applications include managing the severity of the immune response to vector delivery and ensuring the stability of the therapeutic gene. As research continues, innovative strategies are being developed to enhance vector design, aiming for higher delivery efficiency and reduced toxicity.
"The evolution of packaging vectors stands at the forefront of revolutionary changes in gene therapy applications, influencing treatment paradigms for genetic disorders and cancer."
"The evolution of packaging vectors stands at the forefront of revolutionary changes in gene therapy applications, influencing treatment paradigms for genetic disorders and cancer."
Designing Efficient Packaging Vectors
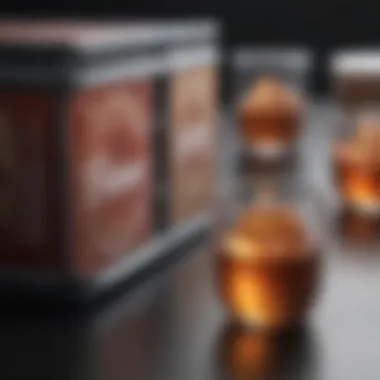
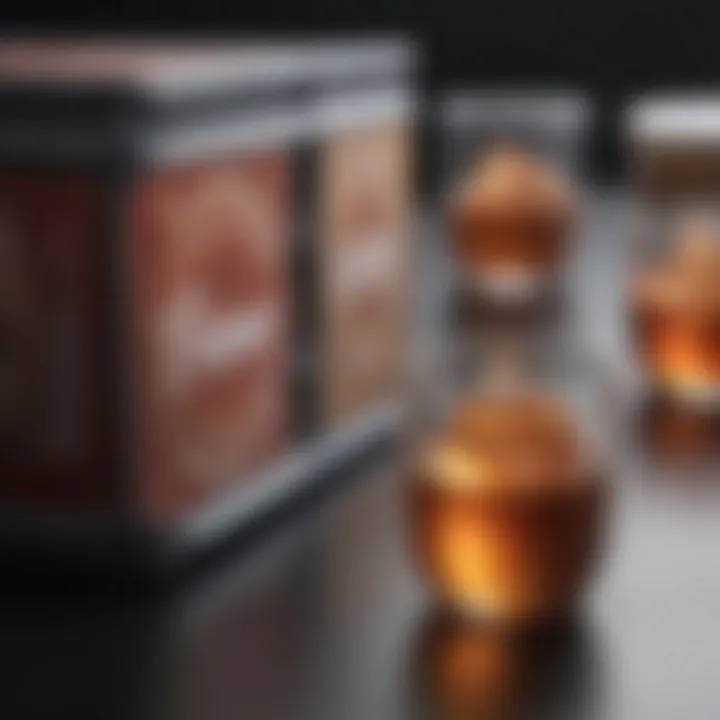
Designing efficient packaging vectors is crucial in the field of molecular biology and genetic engineering. These vectors must effectively deliver genetic material into host cells to achieve desired therapeutic outcomes. Rethinking vector design enhances gene therapy efficacy, reduces immunogenicity, and improves the targeting of specific tissues. An optimized packaging vector can lead to significant advancements in gene delivery systems, enabling breakthroughs in both research and clinical applications.
Optimization Techniques
Optimization techniques are essential for refining the performance of packaging vectors. These techniques can include increasing the transfection efficiency, maximizing payload capacity, and minimizing off-target effects. Researchers often employ various strategies, such as chemical modifications and genetic engineering approaches, to achieve these optimizations.
Key optimization methods involve:
- Modification of surface properties: Altering the charge or hydrophobicity of the vector can enhance its interaction with cellular membranes, leading to higher uptake rates.
- Improving stability: Enhanced stability of vectors in physiological conditions ensures that the genetic material remains intact and functional until it reaches the target cells.
- Targeted delivery: Incorporating ligands that recognize specific receptors on target cells can increase the precision of gene delivery, reducing unwanted side effects.
Innovative Approaches
Innovative approaches in designing packaging vectors focus on harnessing advanced technologies to enhance their efficiency and effectiveness. Two notable directions are Synthetic Biology and Nanotechnology.
Synthetic Biology
Synthetic Biology represents a transformative approach to constructing new biological parts and systems. In the context of packaging vectors, Synthetic Biology can facilitate the design of custom vectors tailored for specific applications. Its key characteristic lies in the ability to create novel constructs with precise control over genetic elements. This modular approach allows researchers to engineer packaging vectors that are not only efficient but also adaptable to various types of therapies.
One unique feature of Synthetic Biology is the use of standardized biological parts, such as promoters and resistance genes. This standardization simplifies the vector design process and fosters collaboration among researchers. The advantage of this methodology is the potential for rapid prototyping and testing of new vectors, which can significantly accelerate the pace of research and clinical translation.
Nanotechnology
Nanotechnology, on the other hand, introduces unique materials and methodologies that tremendously enhance the functionality of packaging vectors. The specific aspect of Nanotechnology that contributes significantly to efficient vector design is its ability to utilize nanoscale materials to improve delivery systems. These materials can encapsulate genetic material effectively, enhancing stability and bioavailability.
The key characteristic of Nanotechnology in this context is its capacity for precise control over particle size, shape, and surface chemistry. These attributes are particularly beneficial in overcoming biological barriers to gene delivery, such as cell membranes. A fundamental advantage is that nanomaterials can also facilitate the co-delivery of therapeutic agents alongside genetic material, which may enhance overall therapeutic efficacy. However, challenges include potential toxicity and regulatory hurdles that need careful consideration throughout the vector development process.
Efficiently designed packaging vectors can revolutionize gene therapy and molecular research, leading to more targeted and effective treatments.
Efficiently designed packaging vectors can revolutionize gene therapy and molecular research, leading to more targeted and effective treatments.
In summary, the design of efficient packaging vectors involves a multi-faceted approach, incorporating optimization techniques and innovative strategies from areas such as Synthetic Biology and Nanotechnology. The ongoing exploration of these pathways promises to unlock new potential in gene therapy and research efficacy.
Safety and Ethical Considerations
Understanding the safety and ethical considerations surrounding packaging vectors is of utmost relevance in modern scientific research. As these vectors play a pivotal role in gene delivery and therapy, ensuring their safe use and adherence to ethical guidelines is crucial. Without appropriate regulations and ethical frameworks, the potential for misuse and unintended effects increases significantly. This section delves into the regulatory measures and the dichotomy of potential risks and benefits associated with packaging vectors.
Regulatory Frameworks
Regulatory frameworks are essential for overseeing the use of packaging vectors in research and clinical applications. These frameworks help maintain a balance between innovation and safety. Key regulations often involve guidelines set forth by organizations such as the World Health Organization (WHO), the U.S. Food and Drug Administration (FDA), and the European Medicines Agency (EMA). These agencies establish protocols for:
- The evaluation of new techniques and technologies in genetic modifications.
- The risk assessment processes that consider both the intended outcomes and unintended consequences.
- Ongoing monitoring of clinical trials to ensure participant safety and data integrity.
Countries may also have unique guidelines depending on local laws and ethical norms. This can lead to variations in the approval process for gene therapies, which can affect international collaboration in research.
Potential Risks and Benefits
The exploration of packaging vectors presents a complex interplay of risks and benefits. Each innovation brings considerable promise as well as challenges. The following points highlight these aspects:
Potential Risks:
- Immune Reactions: Both viral and non-viral vectors can trigger immune responses, which can negate therapeutic effects.
- Unintended Genetic Changes: The integration of foreign genes can unintentionally alter other important genomic sites, leading to detrimental effects or even cancer.
- Environmental Impact: The release of genetically modified organisms into the environment can disrupt ecosystems if not properly controlled.
Potential Benefits:
- Advanced Therapies: Packaging vectors enable targeted delivery of genes, which can treat previously untreatable genetic disorders.
- Personalized Medicine: They facilitate tailor-made treatments based on an individual’s genetic makeup, promising higher effectiveness due to specificity.
- Research Advancements: Innovations improve our understanding of gene functions and interactions, contributing to foundational biological knowledge.
In essence, the discourse surrounding packaging vectors must always weigh both potential breakthroughs against inherent risks.
In essence, the discourse surrounding packaging vectors must always weigh both potential breakthroughs against inherent risks.
Ensuring safety and promoting ethical practices are not mere regulatory necessities; they are fundamental to sustaining the trust of the public and the scientific community. Comprehensive regulations and continuous dialogue between stakeholders are necessary to navigate these complex issues effectively.
Emerging Trends in Packaging Vectors
The field of packaging vectors is constantly evolving. Emerging trends indicate advancements that hold great potential for scientific research and therapeutic approaches. As research progresses, new technologies and methodologies are changing how vectors are designed and utilized. Understanding these trends is crucial for researchers and professionals who aim to implement the latest developments in gene delivery systems.
CRISPR-Cas Systems
CRISPR-Cas systems have revolutionized genetic engineering and significantly influenced the design of packaging vectors. These systems provide a mechanism for precise editing of genomes, making them an invaluable tool in modern biotechnology.
The integration of CRISPR technology with packaging vectors enhances the efficiency of gene editing and delivery. This combination allows for targeted delivery of CRISPR components to specific cells, reducing off-target effects. Furthermore, using viral vectors, like lentiviral or adenoviral systems, increases the delivery success rates of CRISPR components to desired tissues. The outcome of this synergy provides a platform for advanced therapies in various diseases, from genetic conditions to certain cancers.
Some key points regarding CRISPR-Cas systems in the context of packaging vectors include:
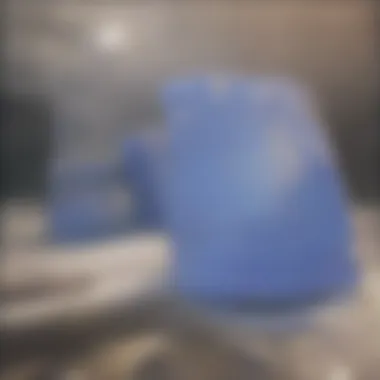
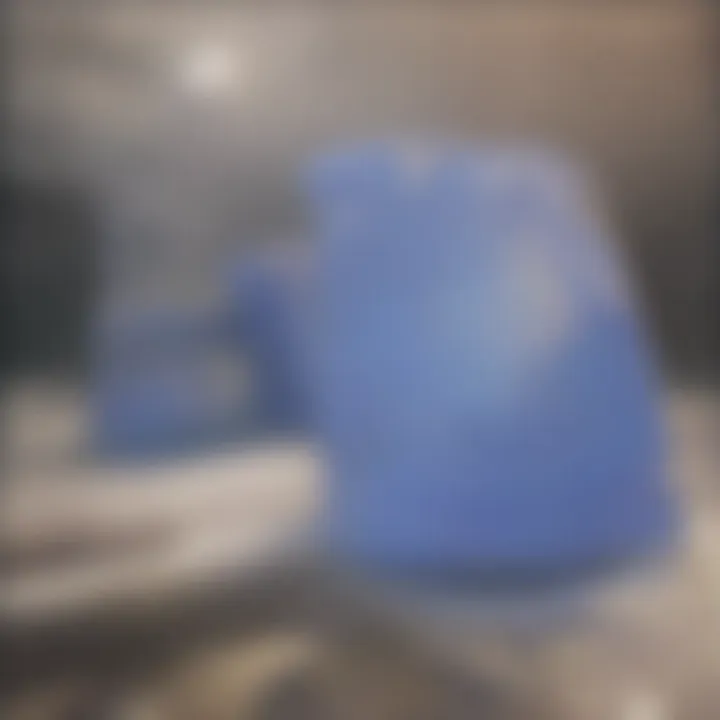
- Targeted Gene Editing: Enhances specificity in genetic modifications.
- Increased Delivery Efficiency: Ensures CRISPR components reach intended cells.
- Versatility of Applications: Adaptable for different types of treatments.
Vectored Antigen Delivery
Vectored antigen delivery represents another significant trend in packaging vectors. This method employs viral or non-viral vectors to deliver antigens into host cells, allowing the body’s immune system to recognize and respond to potential pathogens. This approach is particularly useful in vaccine development, where the goal is to elicit an immune response without exposing individuals to the actual pathogen.
The advantages of vectored antigen delivery are considerable, especially in the context of emerging infectious diseases and public health responses. By using vectors to introduce specific antigens, researchers can create targeted vaccines that are potentially more effective and swift to produce.
Some benefits of vectored antigen delivery include:
- Improved Vaccine Efficacy: Targeted delivery enhances immune responses.
- Rapid Development: Allows quicker vaccine design in response to outbreaks.
- Broader Range of Applications: Useful for various infectious and non-infectious diseases.
The integration of innovative packaging vectors is crucial in changing the landscape of vaccine development and therapeutic intervention.
The integration of innovative packaging vectors is crucial in changing the landscape of vaccine development and therapeutic intervention.
Challenges in Current Research
The exploration of packaging vectors has become a focal point in scientific research, particularly in molecular biology and genetic engineering. However, various challenges persist that can hinder effective applications and advancements. Addressing these challenges is vital for the continued development of this field. This section discusses some of the most significant issues currently faced in research involving packaging vectors.
Delivery Efficiency
One major challenge is ensuring high delivery efficiency of packaging vectors into target cells. The efficiency of delivery affects how well a therapeutic gene can exert its effects once it is integrated into the host genome. Factors influencing delivery efficiency include the type of packaging vector used, the method of delivery, and the specific characteristics of the target cells. For example, viral vectors generally show a higher delivery efficiency compared to non-viral methods; however, they also come with safety concerns.
Research is ongoing to improve delivery methodologies. Techniques such as microinjection, electroporation, and liposome-based delivery are under investigation. Each of these techniques has its pros and cons, and the refinement of these methods is essential to enhance success rates. The development of new strategies must also focus on the specificity of delivery, ensuring that the intended cells are targeted to avoid unintended consequences.
Additionally, optimizing the physical and chemical properties of vectors can impact their ability to penetrate cellular barriers. Continued research into this area is crucial to reduce off-target effects and improve overall therapeutic impact.
Immune Response
Another significant challenge is the immune response elicited by packaging vectors, especially those derived from viral origins. The immune system may recognize these vectors as foreign, prompting an immune response that can lead to the destruction of the vector before it delivers its payload. This creates a significant barrier to the effectiveness of gene therapies.
Researchers study the immunogenic properties of different vectors to understand how they interact with the host's immune system. Strategies such as improving vector design or employing immunosuppressive drugs could mitigate adverse immune reactions. The administration schedule is also a factor to consider, as repeated doses may provoke a more robust immune response.
Future Directions
The future of packaging vectors in scientific research is an area of intense exploration and innovation. As we consider the trajectory of gene therapy and molecular biology, it becomes clear that the advancement in this field has potential to profoundly impact healthcare and biomedicine. Packaging vectors are not just tools for gene delivery; they can be pivotal in personalized medicine and collaborative research initiatives. This section will delve into these specific elements, emphasizing their significance and the associated benefits.
Personalized Medicine
Personalized medicine represents a shift from a one-size-fits-all approach to healthcare. It tailors treatment based on individual genetic profiles, resulting in more effective and targeted therapies. Packaging vectors play a crucial role in this transformation by enabling precise gene delivery mechanisms.
Utilizing vectors such as lentiviral and adeno-associated virus vectors can facilitate the introduction of therapeutic genes specifically tailored to an individual's genetic makeup. This method can improve the efficacy of treatment for genetic disorders, cancer, and other diseases. In addition, the ability to manipulate vectors allows for adaptations that enhance their targeting capability, ensuring that therapeutic payloads only reach the desired cells.
In practice, personalized medicine can lead to significant improvements in treatment outcomes. For example, by using vectors optimized for specific genetic mutations, researchers can develop treatments that are more likely to succeed, minimizing the trial-and-error approach that traditionally characterizes drug development.
Collaborative Research Initiatives
The complexity of modern scientific challenges necessitates collaboration across disciplines. In the realm of packaging vectors, this theme is particularly pronounced. Collaborative research initiatives can accelerate the development of innovative vectors through shared expertise and resources. By combining knowledge from molecular biology, engineering, and bioinformatics, researchers can create more sophisticated and effective packaging vectors.
"Collaboration is not just a strategy; it is an essential pathway to innovation in science."
"Collaboration is not just a strategy; it is an essential pathway to innovation in science."
International partnerships can also expand the geographic reach of research. By pooling resources from different institutions and laboratories, scientists can tackle larger-scale studies and clinical trials more efficiently. Diverse teams can bring unique perspectives that may lead to breakthroughs in vector design and optimization.
Furthermore, interdisciplinary approaches can enhance our understanding of the ethical implications surrounding the use of packaging vectors. Engaging bioethicists alongside researchers in genetic sciences ensures that societal considerations are integrated into scientific advancements.
Epilogue
The conclusion brings together the intricate threads explored in this article about packaging vectors. It is essential to recognize the profound impact these vectors have on molecular biology and genetic research. They are not merely tools for gene delivery but are crucial in engineering therapies that have the potential to transform medical practices. By emphasizing the significance of design, effectiveness, and safety, we ensure that the use of packaging vectors is both innovative and responsible.
Summary of Key Insights
In this article, we have detailed various dimensions of packaging vectors:
- Definition and Importance: Understanding packaging vectors is crucial for perspectives in genetics, as they facilitate gene delivery efficiently.
- Types of Vectors: Differentiating between viral and non-viral vectors underscores their diverse applications and unique capabilities.
- Mechanisms of Action: An examination of how these vectors operate at a cellular level reveals the complexities involved in gene therapy.
- Applications and Innovations: The discussion reflected on current methodologies and breakthroughs made through new designs.
- Safety and Ethics: Addressing regulatory concerns fosters an environment of trust and responsibility in scientific exploration.
- Challenges and Future Directions: Acknowledging the hurdles in delivery and immune responses sets the stage for future academic inquiries and technological advancements.
"Packaging vectors have the potential to revolutionize not just therapy but the entire landscape of genetic research."
"Packaging vectors have the potential to revolutionize not just therapy but the entire landscape of genetic research."
Implications for Future Research
Looking ahead, several crucial directions emerge from this exploration of packaging vectors:
- Personalized Medicine: As research advances, the customization of gene therapies using specific vectors may become a norm, allowing for more tailored and effective treatments.
- Collaborative Research: Interdisciplinary efforts among scientists will likely drive innovations that enhance the application and efficiency of packaging vectors.
- Technical Innovations: Continued efforts in optimizing vector design could lead to minimized immune responses and increased delivery efficacy, propelling the field into new territories of research.
In summary, the dynamics of packaging vectors hold profound potential for the enhancement of gene therapy and broader genetic research while warranting careful consideration of safety and ethical frameworks.