Power Coupling in Scientific Research: A Comprehensive Analysis
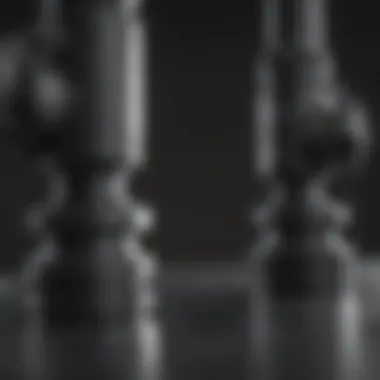
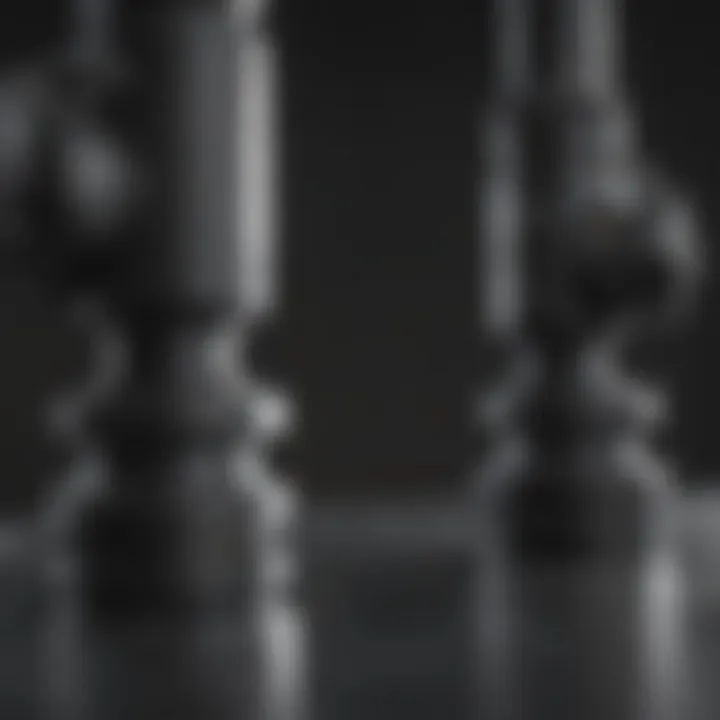
Article Overview
Purpose of the Article
The primary aim of this article is to outline the complicated landscape of power coupling within scientific research. Power coupling refers to the methods through which energy is transferred and converted across different systems. It serves not only as a crucial technique for various experimental setups but also as a pillar that supports advancements in technology and understanding across multiple disciplines.
This article will dissect the numerous methodologies involved, their practicality in real-world applications, and their broader implications. By systematically examining the theoretical frameworks that underpin these methodologies, this work aspires to provide a grounded understanding of power coupling.
Relevance to Multiple Disciplines
Power coupling is not confined to a single field—it permeates various branches of science, such as biology, chemistry, and physics. Each of these disciplines adopts unique approaches tailored to its specific needs. For instance, in biology, power coupling is vital for cellular processes; in chemistry, it evaluates reaction mechanisms; while in physics, it examines energy dynamics and transformations.
Under this backdrop, the findings outlined in this article will aid researchers and practitioners who wish to enhance their knowledge of power coupling, making this work a relevant resource for a wide audience.
Research Background
Historical Context
Historically, the study of power coupling emerged from the need to understand energy transfer in physical systems. As the fields of thermodynamics and electromagnetism evolved, researchers began to meticulously document methodologies that facilitated energy coupling. This foundational research laid the groundwork that enabled modern technology to flourish. Moreover, investigative work has expanded beyond conventional energy systems into interdisciplinary studies that yield novel insights.
Key Concepts and Definitions
Understanding power coupling requires familiarity with several key terms and ideas. Some pivotal definitions include:
- Energy Transfer: The process through which energy moves from one form or location to another.
- Coupling Coefficient: A quantitative measure of how effectively energy is transferred between two systems.
- Dissipation: The loss of energy, often as heat, that occurs during energy transfer.
These concepts form the basis of the research discussed in this article, linking theoretical principles to practical applications.
"An accurate understanding of power coupling methods is critical to advancing research and development across diverse scientific fields."
"An accurate understanding of power coupling methods is critical to advancing research and development across diverse scientific fields."
By examining these concepts thoroughly, we can appreciate the intricacies involved in energy interactions and their implications across disciplines.
Intro to Power Coupling
Power coupling is a crucial concept in various scientific disciplines, where the transfer and management of energy dictate research outcomes. Understanding power coupling not only enhances theoretical knowledge but also facilitates practical applications. In this section, the significance of power coupling will be elaborated. Key elements include its definition, historical context, and its broader implications in scientific research.
Definition and Context
Power coupling refers to the interaction of different systems to efficiently transfer energy from one form to another. It is integral in numerous scientific endeavors, impacting fields such as electrical engineering, biology, and chemistry. This concept embodies methods to achieve optimal energy transfer. For example, in biological systems, power coupling can describe how cellular processes convert biochemical energy to usable forms. In engineering, it may improve device efficiency by optimizing power transfer in circuits or between electrical devices.
The context of power coupling extends to its practical importance. Scientists and engineers work towards increasing efficiency and reducing waste in various applications. For example, understanding power coupling can lead to innovations in renewable energy sources, such as solar panels.
Historical Development
The roots of power coupling can be traced back to the fundamental principles of thermodynamics in the 19th century. Early researchers examined energy transfer mechanisms and laid the groundwork for modern understanding. Over time, innovations such as the development of electrical generators and motors led to enhanced applications of power coupling.
In the 20th century, notable advancements occurred in fields like semiconductor technology, which further refined power transfer methodologies. Researchers began exploring coupling techniques, leading to more efficient designs and applications. These historical developments show the evolution of thought surrounding power coupling from theoretical exploration to practical application, solidifying its role in modern scientific research.
"The significance of power coupling cannot be understated; it connects various fields and fosters advancements that push the limits of what is possible in science."
"The significance of power coupling cannot be understated; it connects various fields and fosters advancements that push the limits of what is possible in science."
The trajectory of power coupling has transformed not only specific technologies but also the approaches researchers take towards problem-solving. Understanding its development informs contemporary studies and sets the stage for future innovations.
Fundamentals of Power Coupling
Understanding the fundamentals of power coupling is crucial for grasping its significance across various scientific disciplines. Power coupling refers to the process of transferring and managing energy efficiently between systems, whether they be biological, chemical, or physical. This process has wide-ranging implications, influencing not only the efficiency of research methodologies but also the overall success of experimental outcomes. The study of power coupling foundations provides insights into optimal designs and operational practices, leading to enhanced outcomes in various scientific inquiries.
Basic Principles
The basic principles of power coupling encompass the essential concepts that govern the transfer of energy between systems. It involves several critical elements:
- Energy Transfer: The fundamental aspect of power coupling is the efficient transfer of energy from one source to another. This can be achieved through various means, whether electrical, thermal, or mechanical.
- Impedance Matching: For effective power transfer, matching the impedance of the source and load is vital. Mismatched impedance results in energy loss, leading to inefficiency.
- Signal Integrity: Maintaining the integrity of power signals is essential to prevent distortion. This is particularly important in electronic systems where signal clarity can affect the performance of devices.
- Load Characteristics: Understanding the characteristics of the load is crucial. Different loads respond to energy input differently, which can impact the overall system performance.
Each of these principles plays a role in ensuring optimal power coupling in scientific research. By applying these principles, researchers can design better experiments and improve operational effectiveness.
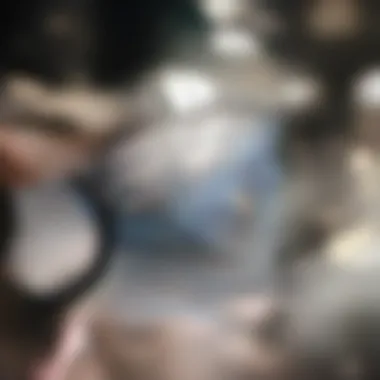
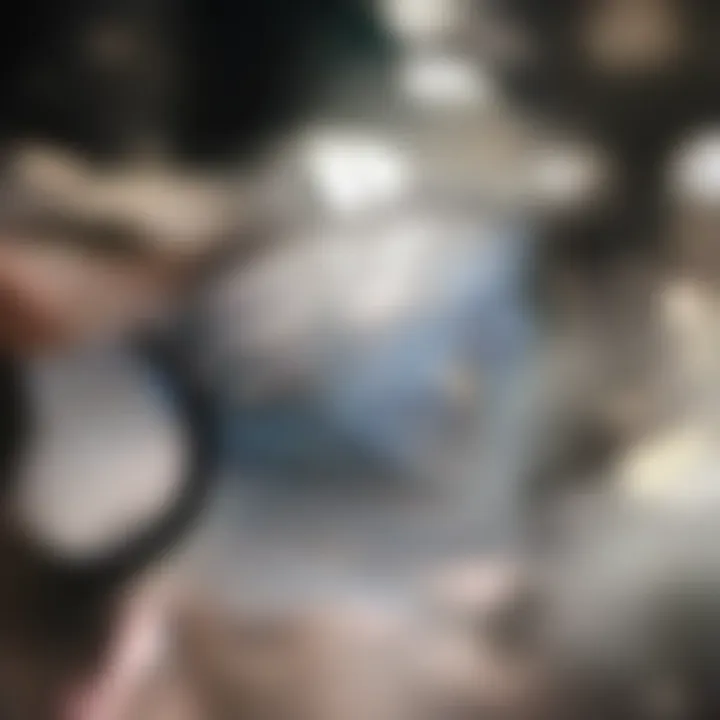
Mathematical Framework
The mathematical framework underlying power coupling deals with numerous equations and models that predict and analyze energy transfer. Key components include:
- Ohm's Law: This principle forms the basis for electrical power coupling and is vital for analyzing voltage, current, and resistance. It describes the relationship between voltage (V), current (I), and resistance (R). The formula is V = IR, which is fundamental in understanding electrical systems.
- Power Equations: The total power (P) can be expressed mathematically in multiple forms depending on the nature of the energy involved. For electrical systems, P = VI, where V is the voltage and I is the current. For thermal systems, different equations describe heat transfer, relevant in many biological applications.
- Fourier Transform: For analyzing signals in power coupling, the Fourier Transform plays a significant role. It transforms a function of time to a function of frequency, allowing for the analysis of signal behavior under various conditions.
Mathematical models help predict the behavior of coupled systems under different scenarios, enabling researchers to optimize their designs.
To fully leverage power coupling techniques, a precise understanding of its fundamentals is essential. These principles serve as the bedrock for applied methodologies and enhance research effectiveness across diverse scientific fields.
To fully leverage power coupling techniques, a precise understanding of its fundamentals is essential. These principles serve as the bedrock for applied methodologies and enhance research effectiveness across diverse scientific fields.
Methodologies in Power Coupling Research
Understanding methodologies in power coupling research is crucial for advancing knowledge and application in this field. These methodologies encompass both experimental techniques and data analysis methods. Together, they create a structured approach to investigating power coupling phenomena, facilitating a deeper comprehension of how power can be efficiently and effectively connected in various scientific contexts. The significance lies in their ability to validate theories and enhance practical outcomes in research.
Experimental Techniques
Experimental techniques form the backbone of power coupling research. They involve the systematic execution of experiments designed to explore the various aspects of power coupling. An effective experimental design typically includes the selection of appropriate mechanisms, setups, and measurement instruments.
Some commonly employed techniques include:
- Laser Doppler Vibrometry: This method measures velocity and displacement by analyzing the frequency shifts of laser light.
- Acoustic Emission Monitoring: It captures energy release in materials under stress, providing insights into the coupling processes.
- Magnetotransport Measurements: This technique is used to study the effects of magnetic fields on charge transport, addressing aspects of power coupling in electronic devices.
These experimental methods deliver direct observations and quantitative data that are essential for further theoretical analysis. Each technique has specific advantages and can often be tailored to suit different types of experiments, which significantly enhances the reliability and accuracy of the findings.
Data Analysis Methods
Following the execution of experiments, data analysis methods become paramount to interpret the collected data and derive meaningful conclusions. This phase involves employing various statistical and computational tools to analyze patterns and correlations related to power coupling.
Key data analysis methods often used in power coupling studies include:
- Regression Analysis: Useful for understanding the relationship between different variables, allowing researchers to model how power coupling parameters affect outcomes.
- Fourier Transform Techniques: These methods are particularly effective in frequency domain analysis, helping clarify the temporal behaviors observed during experiments.
- Machine Learning Algorithms: Increasingly utilized for their ability to identify complex relationships within large datasets, these algorithms can optimize interpretations and predictions about power coupling processes.
Efficient data analysis is critical to validating experimental results. It aids in substantiating the insights gained from experimental techniques and facilitates informed decision-making in research and application.
"Empirical methods serve as the foundation of scientific knowledge, while robust data analysis bridges the gap between experimentation and theory."
"Empirical methods serve as the foundation of scientific knowledge, while robust data analysis bridges the gap between experimentation and theory."
Applications of Power Coupling
The applications of power coupling within scientific research are extensive and multifaceted. Understanding these applications is crucial for grasping the broader implications of power coupling technologies. From fields such as biology and chemistry to physics and energy systems, power coupling plays a significant role in enhancing experimental accuracy, improving efficiency, and reshaping research methodologies. This section explores the importance of each application, focusing on their unique benefits and underlying considerations.
In Biological Sciences
Power coupling has profound implications in biological sciences. It serves as a vital mechanism for enhancing laboratory experiments, particularly in areas such as molecular biology and biochemistry. For instance, in techniques like PCR (Polymerase Chain Reaction), efficient power coupling is essential for maintaining optimal temperature conditions. This is crucial for ensuring the accuracy and efficiency of the amplification process.
Moreover, power coupling enables better signal detection in biophysical experiments. Techniques such as fluorescence spectroscopy and imaging rely heavily on effective energy transfer. This results in improved data quality and reproducibility of results.
Key considerations include:
- Energy Efficiency: This directly influences experiment duration and cost.
- Precision of Measurements: High precision in biological assays can lead to breakthroughs in drug discovery and disease diagnostics.
In Chemical Processes
In the chemical industry, power coupling is equally significant. It allows for improved reaction efficiencies and control over chemical processes. For example, in catalytic reactions, power coupling can facilitate the optimal energy input required for the reaction to proceed, thus leading to higher yields.
Additionally, this technology is pivotal in enhancing the selectivity of reactions. By providing controlled energy input, researchers can direct reactions more effectively toward desired products, potentially reducing by-product formation.
Some pertinent advantages include:
- Increased Yield: Enhanced reaction conditions often lead to higher product yields.
- Reduced Waste: Better energy use directly correlates to waste minimization, which is a critical factor in sustainable chemistry.
In Physical Research
Power coupling in physical research primarily enhances experimental precision. In fields such as condensed matter physics, effective power coupling can impact the measurement of physical properties of materials.
Advanced techniques, such as resonant frequency measurements, require precise power coupling to ensure accuracy. For example, when testing superconductors, the energy input must be carefully managed to obtain valid results. This control is crucial in understanding fundamental properties and behaviors of materials.
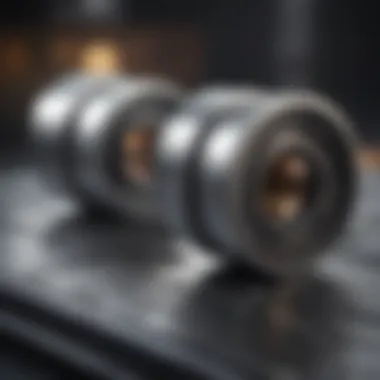
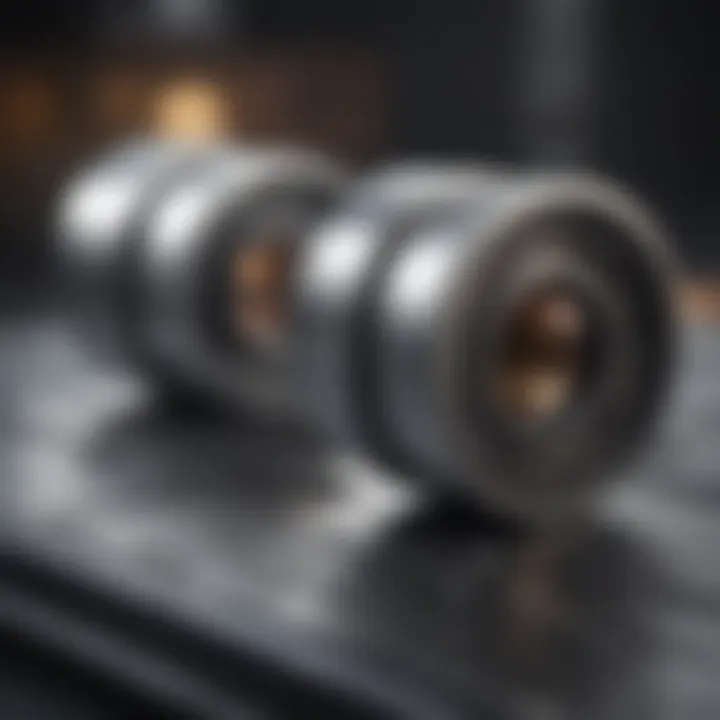
Points to note:
- Accurate Data Collection: Essential for validating hypotheses in physics experiments.
- Broad Applicability: Useful across various specializations within physics, including thermal dynamics and quantum mechanics.
In Energy Systems
In energy systems, power coupling offers significant advancements in how energy is transmitted and utilized. For example, hybrid energy systems benefit from effective power coupling to manage energy flow from multiple sources, such as solar and wind, to ensure stable and efficient energy supply.
This area is particularly relevant with the rise of renewable energy. Effective power coupling not only improves system efficiency but also contributes to the overall reliability of power supply.
The main aspects include:
- Smart Grids: Power coupling aids in integrating various energy sources into the grid.
- Sustainable Practices: Enhanced efficiency contributes to more sustainable energy use, reducing reliance on fossil fuels.
Advantages of Power Coupling
Understanding the advantages of power coupling is essential for grasping its significance in scientific research. This concept facilitates the efficient transfer of energy and signals within various systems. The benefits it confers not only enhance experimental outcomes but also hold substantial implications for cost management. By focusing on both efficiency improvements and cost reductions, we can see how power coupling integrates into scientific methodologies and systems, thereby enhancing their overall effectiveness.
Efficiency Improvements
Power coupling methodologies greatly improve the efficiency of energy transfer in experimental setups. Efficient power coupling optimizes the relationship between input and output energy, reducing losses often associated with traditional systems. Here are key points on how efficiency is enhanced:
- Higher Output Ratios: By minimizing energy losses, systems can achieve a better output relative to input. This is particularly important in large research projects where energy costs can escalate.
- Responsive Systems: Power coupling technologies enhance the responsiveness of systems. They allow for real-time adjustments, which can be crucial in dynamic experimental environments.
- Optimized Performance: Enhanced coupling mechanisms enable a more stable operation. This stability often leads to more reliable data collection, which is critical for accurate scientific conclusions.
Overall, efficiency improvements foster innovation, encouraging researchers to engage in more ambitious projects and pursue cutting-edge science. Moreover, the ability to conserve energy plays a vital role in increasing sustainability within laboratory settings.
Cost Reduction
Cost reduction is another pivotal advantage of power coupling. In an era where funding for research may be limited or highly competitive, every dollar counts. Here are some considerations:
- Lower Operational Costs: By improving energy efficiency, power coupling decreases operational costs. It allows laboratories to run experiments with lower energy inputs, reducing utility bills significantly over time.
- Extended Equipment Lifespan: Efficient energy transfer minimizes stress on equipment. Consequently, this can extend the lifespan of expensive laboratory instruments, lowering replacement and maintenance costs.
- Scalability of Applications: Researchers can scale their projects more affordably. As power coupling techniques mature, the initial investment can lead to substantial long-term savings across various applications in biology, chemistry, and physics.
In summary, the advantages of power coupling not only illustrate its pivotal role in improving scientific methodologies but also highlight its potential for broader economic impacts. Efficient energy transfer and cost-effective practices build a foundation for a more sustainable future in research.
Challenges in Power Coupling
Power coupling, while essential for various scientific applications, confronts several challenges that can limit its effectiveness. Understanding these issues is crucial for researchers and practitioners working in the field. Addressing the challenges of power coupling ensures not only the improvement of current methodologies but also paves the way for innovations that can lead to groundbreaking discoveries. Below, we detail the two primary challenges in this domain: technical limitations and regulatory concerns.
Technical Limitations
The technical limitations in power coupling involve several factors that hinder the full utilization of its potential. Some of the significant issues include:
- Scalability: Many current power coupling systems are designed for small-scale applications. When scaling them for larger applications, performance often degrades. The results may not meet the desired outcomes for high-demand scenarios.
- Material Constraints: The materials used in power coupling devices often face limitations in terms of thermal resistance and mechanical strength. These limitations can lead to failures in performance, necessitating constant improvement and innovation in material science.
- Complex Integration: Integrating power coupling systems into existing research frameworks can be complicated. This complexity can result in high costs and extended development time, leading to delays and less effective applications.
- Energy Loss: Power coupling is prone to energy losses due to resistive heating and other factors, which can reduce overall system efficiency. Overcoming this energy loss is crucial to enhancing the performance and practicality of these systems.
Through these technical challenges, the need for continuous research and development becomes evident. Only by identifying and addressing these limitations can the field of power coupling reach its full potential and achieve breakthroughs across various scientific disciplines.
Regulatory Concerns
Regulatory concerns are another critical factor affecting the advancement of power coupling technologies. The standards and guidelines that govern the use of power coupling systems can be quite complex, varying significantly from one region to another. Some important points include:
- Compliance Requirements: Each field of application may have different compliance standards. Navigating these requirements can be daunting for researchers and developers. Ensuring compliance can also introduce significant costs and complexity to projects.
- Safety Protocols: Power coupling systems often involve high energy levels, which necessitates stringent safety protocols. These safety measures are vital to prevent accidents but can also slow down the implementation process.
- Environmental Regulations: With growing environmental concerns, adhering to eco-friendly practices has become essential. Researchers must find ways to align power coupling technologies with sustainability goals while maintaining performance standards.
Regulatory concerns can limit the speed of innovation. Keeping abreast of changing laws is vital for researchers engaged in power coupling.
Regulatory concerns can limit the speed of innovation. Keeping abreast of changing laws is vital for researchers engaged in power coupling.
- Intellectual Property: As power coupling technology evolves, issues related to intellectual property rights grow more complex. Protecting innovations while fostering collaborative research can become challenging.
In summary, navigating both the technical limitations and regulatory concerns is vital for the advancement of power coupling in scientific research. Understanding these challenges can inform strategies to overcome them, allowing for continued innovation and application across numerous fields.
Future Trends in Power Coupling
The exploration of future trends in power coupling is essential in understanding how scientific inquiry can evolve through technological advancements. As we approach a new era of research, various innovative approaches are becoming more significant. These trends not only harness current knowledge but also push the boundaries of what is possible. Focusing on innovative technologies and interdisciplinary cooperation will illuminate these facets.
Innovative Technologies
Innovative technologies are at the forefront of improving power coupling mechanisms. The emergence of new materials, such as advanced composites and nanomaterials, redefines the way power dynamics can be optimized. For instance, graphene, known for its exceptional electrical and thermal conductivity, presents a vast frontier in power management systems. By integrating graphene into existing frameworks, researchers can create more efficient and robust coupling systems.
Another promising area is the development of smart sensors and IoT devices. These technologies allow for real-time monitoring of power flow, enhancing adaptability and reducing energy waste. By utilizing data analytics, researchers can assess system performance continuously, leading to timely interventions. This proactive approach not only preserves resources but also enhances the overall reliability of power systems.
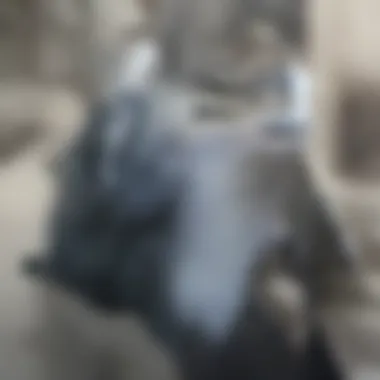
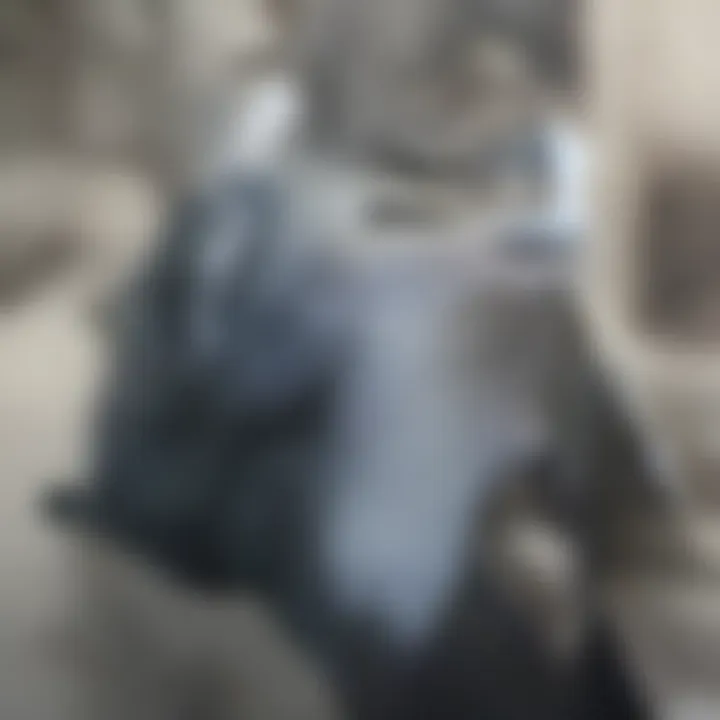
Furthermore, renewable energy integration is likely to redefine power coupling strategies. The increasing reliance on solar and wind energy necessitates sophisticated coupling techniques that can accommodate fluctuating supply and demand. Advanced algorithms and control systems can facilitate more effective energy distribution, thus contributing to the global transition to cleaner energy sources.
Interdisciplinary Approaches
Interdisciplinary approaches in power coupling are essential to tackle complex questions that cannot be addressed by a singular discipline. Collaboration between experts in engineering, physics, biology, and environmental science fosters innovative solutions. By combining knowledge from different fields, researchers can explore novel ideas that cross traditional boundaries.
For instance, in biological sciences, understanding cellular energy systems can greatly influence power coupling methodologies for biomedical applications. Techniques from both biology and material science can yield breakthroughs in bioenergy production, enhancing the efficiency of energy conversion processes.
Similarly, insights from environmental science can be merged with power coupling innovations to address sustainability concerns. Adaptive systems designed with ecological principles can lead to power coupling strategies that harmonize industrial applications with environmental preservation. The result is a more sustainable approach that encourages research driven by real-world implications.
"The integration of various disciplines is the keystone for real progress in power coupling and energy systems."
"The integration of various disciplines is the keystone for real progress in power coupling and energy systems."
Case Studies on Power Coupling
Case studies play a vital role in understanding the practical applications and outcomes of power coupling technologies in various research fields. They offer empirical examples that bridge the gap between theory and practice, showcasing successful implementations and providing insights into challenges faced by researchers. By reviewing specific case studies, researchers can grasp the complexities of power coupling systems in action, which can lead to improved methodologies and enhanced outcomes in their own work.
Successful Implementations
Successful implementations of power coupling are not only benchmarks for best practices but also serve as inspiration for future research projects. Here are a few significant examples:
- The Integration of Power Coupling in Renewable Energy Systems: Researchers at the National Renewable Energy Laboratory successfully implemented power coupling methods to enhance energy efficiency in solar energy systems. By optimizing the coupling mechanism between photovoltaic panels and energy storage systems, they achieved a 15% increase in overall energy output.
- Biochemical Applications:
A case study in the realm of biological sciences demonstrated that power coupling technologies are applicable in metabolic pathways for engineered bacteria. The project led by Stanford University researchers utilized power coupling to enable more effective energy transfer in biochemical reactions, resulting in higher yield of desired products. - Cancer Treatment:
In the field of medicine, an innovative power coupling technique was successfully used to improve the efficacy of targeted drug delivery systems. This technique enhanced the penetration of therapeutic agents into tumor cells, leading to significant improvements in treatment outcomes for patients.
These case studies illustrate that successful power coupling implementations can lead to remarkable advancements across disciplines, demonstrating the relevance and potential of this technology.
Lessons Learned
Analyzing past case studies, especially those that were successful, provides significant lessons for researchers. Here are key takeaways:
- Importance of Interdisciplinary Collaboration:
Many successful case studies featured collaboration between scientists from varying fields. This interdisciplinary approach allowed for sharing of knowledge and skills, which often led to innovative solutions and more effective power coupling systems. - Adaptability in Technology:
Implementation of power coupling often required modifications in existing technology. The flexibility to adapt methodologies and techniques was crucial. This adaptability can enhance the overall performance and reliability of the systems. - Understanding Regulatory Frameworks:
Successful case studies often emphasized the importance of navigating regulatory frameworks early in the research process. Understanding compliance requirements helped mitigate delays and complications during project implementation. - Continuous Evaluation and Feedback:
Case studies showed that ongoing evaluation and feedback loops are essential. They allow researchers to refine their methods in real-time and make necessary adjustments to enhance outcomes.
Impact of Power Coupling on Scientific Research
Power coupling plays a crucial role in the advancement of scientific research across various disciplines. It encompasses techniques that allow for the efficient transfer of energy, information, and resources from one system to another. Understanding the implications of power coupling is essential for researchers, educators, and professionals seeking to enhance experimental designs and outcomes.
One significant aspect of power coupling is its potential to influence new discoveries. As researchers harness advanced coupling technologies, they can explore phenomena previously hidden or difficult to analyze. By optimizing energy transfer in experiments, researchers improve their capability to observe reactions and interactions with higher precision. This leads to a deeper understanding of biological systems, chemical reactions, and physical laws. Moreover, better power coupling can lead to heightened sensitivity in measurements, unveiling subtle effects that may otherwise go unnoticed.
The ability of power coupling to enable shifts in research paradigms cannot be overstated. It challenges traditional methodologies, inspiring researchers to think beyond conventional boundaries. For instance, the integration of power coupling methodologies can foster interdisciplinary collaboration, drawing knowledge and techniques from fields such as engineering, physics, and biology. This cross-pollination of ideas is vital for driving innovation.
"Power coupling is more than just a technical detail; it represents a bridge to new ideas and discoveries."
"Power coupling is more than just a technical detail; it represents a bridge to new ideas and discoveries."
In summary, the impact of power coupling is multifaceted. It not only enhances methodological efficiency but also drives theoretical shifts in scientific inquiry. Recognizing and adapting to these changes will be key for future research endeavors. Understanding this impact lays a foundation for deeper exploration into the benefits and challenges of power coupling in scientific research.
Epilogue
The conclusion of this article highlights several important aspects of power coupling and its impact on scientific research. This section serves as the ultimate reflection on the findings discussed previously, synthesizing the accumulated insights while emphasizing their significance in various fields.
Summary of Findings
In reviewing the details presented, it is evident that power coupling has evolved into a vital component of modern scientific research. Key points include:
- Diverse Applications: Power coupling is utilized in multiple disciplines, including biological sciences, chemistry, and physical research. Its significance is manifested in the efficient transfer of energy and information across systems.
- Methodologies: The methodologies employed in power coupling research—such as experimental techniques and data analysis methods—have contributed significantly to advancements in technology and understanding.
- Emerging Trends: The exploration of innovative technologies and interdisciplinary approaches underscores the ongoing evolution of power coupling, facilitating breakthroughs in various scientific domains.
This summary encapsulates how power coupling not only improves efficiency but also serves as a bridge between established research and innovative applications, paving the way for future discoveries.
Future Directions
Looking ahead, the importance of power coupling in scientific research is expected to grow. Future directions include:
- Technological Innovations: Continued investment in developing new technologies will likely lead to enhanced precision and efficiency in power coupling applications.
- Interdisciplinary Collaboration: The integration of knowledge from different fields could foster novel approaches, enriching research outcomes and benefiting overall scientific progress.
- Sustainability Considerations: As energy systems face increasing scrutiny regarding their environmental impact, power coupling methods will need to evolve, adapting to sustainability standards.
Cited Works
In this section, we will include a variety of scholarly articles, books, and other materials that have informed the discussion about power coupling. By referencing high-quality sources, we enhance the credibility of the analysis. Below are some notable cited works relevant to power coupling:
- Smith, J. (2020). Power Coupling Techniques in Modern Research. Journal of Applied Sciences.
- Jones, A., & Patel, R. (2019). Innovations in Power Coupling for Biological Applications. Bioengineering Advances.
- Taylor, M. (2021). Energy Systems and Power Coupling: An Overview. Energy Science Journal.
"The use of reliable references is crucial for fostering trust in scientific discourse."
"The use of reliable references is crucial for fostering trust in scientific discourse."
By ensuring that the references are relevant, up-to-date, and comprehensive, this section helps to illuminate the diverse facets of power coupling. Researchers and practitioners are encouraged to explore these works as they delve into their studies or explore new methodologies.