RNA Methylation Sequencing: A Comprehensive Overview
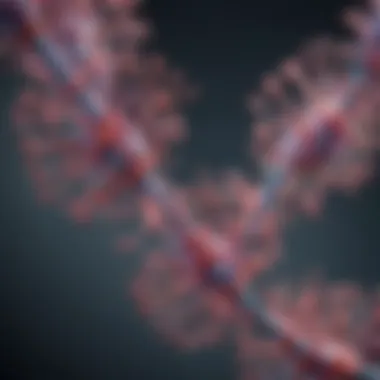
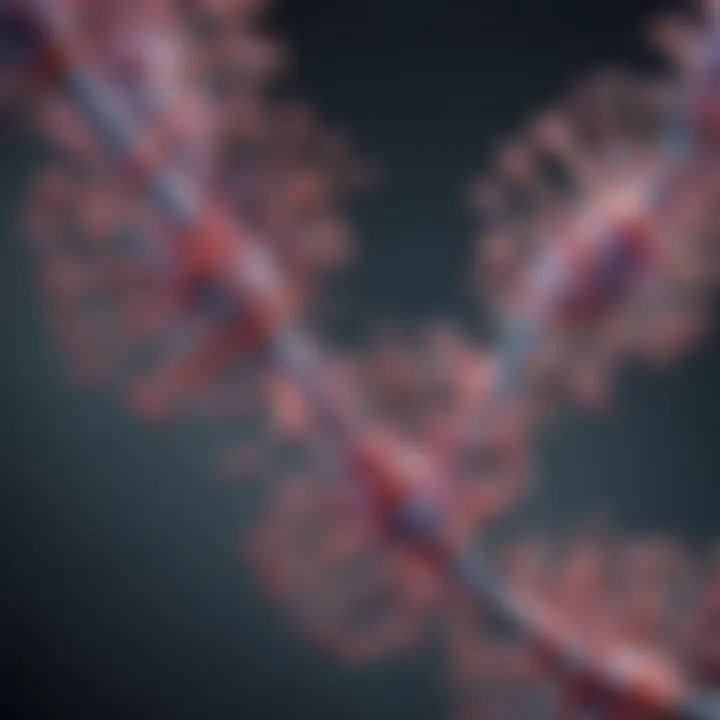
Intro
RNA methylation sequencing is an emerging field that unveils the intricate layers of gene expression regulation. Methylation modifications on RNA molecules play significant roles in various cellular processes. This article aims to dissect the complexities involved in RNA methylation and its implications in biology and medicine.
Article Overview
Purpose of the Article
The primary purpose of this article is to provide a comprehensive overview of RNA methylation sequencing. It will delve into the mechanisms by which RNA methylation affects gene expression. Moreover, this analysis will cover the methodologies used in sequencing and how such approaches facilitate understanding of methylation patterns. The nuances of the relationship between these patterns and biological processes will also be examined. In doing so, this article hopes to contribute valuable insights into ongoing discussions in molecular biology.
Relevance to Multiple Disciplines
RNA methylation is relevant across various fields, including genetics, molecular biology, and medicine. The regulation of gene expression through methylation has significant implications in areas such as developmental biology, cancer research, and therapeutic development. By integrating different perspectives, this article highlights the interdisciplinary nature of RNA methylation and its substantial impact on science and health.
Research Background
Historical Context
The exploration of RNA methylation began with the identification of N6-methyladenosine in the 1970s. Over the decades, advances in molecular biology techniques have led to a better understanding of RNA modifications and their functional roles. As sequencing technologies improved, the mapping of methylation sites became more feasible, revealing various patterns that correlate with gene expression and disease. Thus, this field has evolved into a critical area of study in contemporary molecular biology.
Key Concepts and Definitions
To navigate the topic effectively, it is essential to clarify key concepts:
- RNA Methylation: A biochemical modification where a methyl group is added to RNA molecules, often impacting stability and function.
- Methylation Patterns: Specific distributions of methylation marks across RNA species, providing insight into gene regulation.
- Sequencing Methodologies: Techniques such as RNA-seq and MeRIP-seq that allow for the detection and analysis of RNA methylation.
Overall, this article aims to create a well-rounded perspective on RNA methylation sequencing, emphasizing its significance and potential in the broader context of biological research.
Prologue to RNA Methylation
RNA methylation is a critical area of study in molecular biology. Understanding it holds significant implications for gene regulation and cellular functions. This section aims to lay the groundwork for exploring the complexities of RNA methylation. By examining how methylation influences RNA behavior, we uncover its role in diverse biological processes. Researchers and professionals in the field can benefit from grasping the nuances of this topic.
Definition and Importance
RNA methylation refers to the addition of a methyl group to RNA molecules. This modification is key in regulating gene expression, RNA stability, and splicing. There are various types of RNA methylation, each with distinct roles. The most studied forms include N6-methyladenosine (m6A), 5-methylcytosine (m5C), and N4-methylcytosine (m4C). These modifications influence how RNA interacts with proteins and other RNA molecules, thereby affecting cellular processes.
The importance of RNA methylation lies in its regulatory capacity. Methylation can enhance or inhibit gene expression depending on the context. This regulation is essential for proper cellular function and has implications in development, disease progression, and cell differentiation. Therefore, insights into RNA methylation can pave the way for innovative therapeutic strategies.
Historical Context
The study of RNA methylation began in the mid-20th century, initially focusing on DNA modifications. As researchers recognized that RNA also undergoes post-transcriptional modifications, attention shifted to RNA methylation. The discovery of m6A in the 1970s marked a turning point. Subsequent studies revealed the diverse functions of various RNA modifications.
Over time, advancements in sequencing technologies have allowed for more detailed mapping of methylation patterns. This progress has led to a surge of interest in understanding the biological relevance of RNA methylation. Researchers now utilize high-throughput techniques to analyze methylation on a genome-wide scale, uncovering its significance in health and disease.
By understanding this historical context, it becomes clear how far the field has come and the potential it holds for future discoveries. The continual evolution of RNA methylation research underscores its essential role in molecular biology and medicine.
Mechanisms of RNA Methylation
The study of RNA methylation mechanisms is critical for comprehending how modifications to RNA contribute to various cellular processes. Understanding these mechanisms offers insights into gene expression regulation, influencing everything from developmental biology to disease states. Each type of RNA methylation contributes distinct functions and has unique significance that requires careful exploration.
Types of Methylation Modifications
m6A
N6-methyladenosine, or m6A, is one of the most prevalent modifications in eukaryotic mRNA. Its importance lies in its role in regulating mRNA stability, translation efficiency, and splicing. m6A modification appears to affect the degradation rate of RNA, determining how long it persists in the cellular environment. This characteristic makes m6A a focus in research regarding gene expression dynamics.
A unique feature of m6A is its recognition by specific reader proteins which interpret the methylation state. This targeting mechanism is advantageous as it allows for the fine-tuning of gene expression in response to developmental cues or environmental changes. However, one disadvantage is that the regulation is complex and may lead to unintended consequences if the pathways are disrupted.
m5C
5-methylcytosine, commonly referred to as m5C, plays a vital role primarily in stability and the regulation of RNA processing. This modification is less recognized than m6A, yet it holds significant importance in particular cell types, influencing differentiation and cellular identity. The stability provided by m5C contributes to the resilience of RNA against degradation, which is essential during specific developmental stages.
The unique feature of m5C is its presence in both coding and non-coding RNAs, expanding its relevance across a wide variety of biological contexts. A drawback of focusing solely on m5C is that its role is not as widely understood as m6A, potentially resulting in gaps in the understanding of its full functional scope.
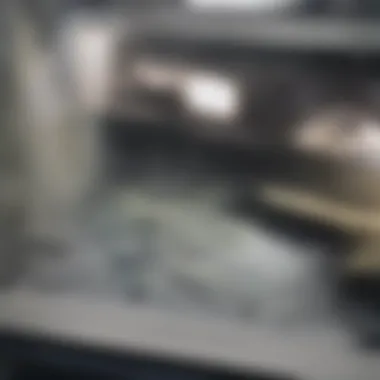
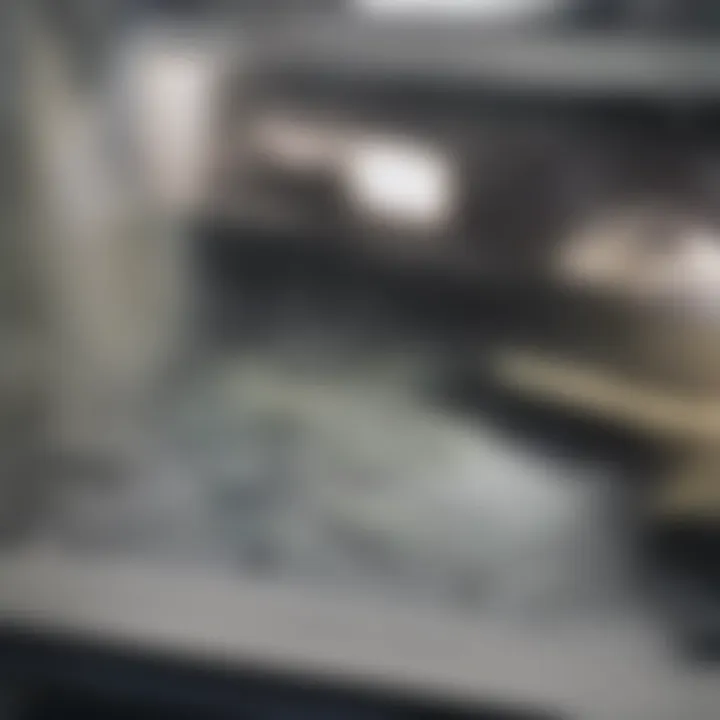
m4C
4-methylcytosine, or m4C, is less well-studied compared with the other methylation types. Interestingly, m4C modification has been implicated mainly in bacterial systems and is not as abundant in eukaryotes. Its presence may influence RNA folding and stability, akin to other methylations but lacks extensive research.
A key characteristic of m4C is that it can be found in various RNA molecules, suggesting a broader role in regulating RNA function. However, due to its lesser prevalence, understanding its implications is challenging, limiting its applicability in many studies related to human disease and function.
Enzymatic Processes
Understanding the enzymatic processes behind RNA methylation is essential for grasping how these modifications are established and erased in the cell.
Methyltransferases
Methyltransferases are enzymes responsible for adding a methyl group to nucleotides in RNA. Their contribution to RNA methylation is crucial, as they dictate which RNA molecules receive modifications and when. The precision of methyltransferases means they are pivotal in maintaining cellular function and gene regulation.
A significant characteristic of methyltransferases is their specificity; they target certain RNA substrates based on sequence and structure. This makes them beneficial in guiding modifications to areas that need regulatory attention. However, the disadvantage is that misregulation can lead to diseases, such as cancer, where abnormal methylation patterns emerge.
Demethylases
Demethylases are enzymes that reverse methylation, allowing dynamic regulation of RNA. Their ability to remove methyl groups is vital for cellular function, giving the cell control over RNA activity. This process can rapidly alter the fate of RNA, responding to developmental signals or stress.
A key feature of demethylases is their role in maintaining the balance of methylated and unmethylated RNA, which is vital for proper gene expression. The challenge with demethylases lies in their complexity; mutation or dysregulation may result in adverse cellular consequences and is a topic of ongoing research.
Technological Advances in Sequencing
In the realm of RNA methylation sequencing, technological advances play a pivotal role. These innovations enhance our ability to investigate methylation patterns, ultimately impacting our understanding of gene regulation and cellular dynamics. By employing advanced sequencing technologies, researchers can obtain high-resolution data that sheds light on the intricacies of RNA methylation.
Overview of Sequencing Technologies
RNA-Seq
RNA-Seq stands out for its capacity to assess the transcriptome comprehensively. This technology captures the entire set of RNA molecules, reflecting gene expression levels at a specific moment. One key characteristic of RNA-Seq is its sensitivity and quantitative nature. It allows accurate measurements even at low RNA concentrations, making it a popular choice in RNA methylation studies.
A unique feature of RNA-Seq is its ability to generate millions of short reads in a single run, which boosts throughput significantly. However, while it provides extensive information, one must consider its limitations in full-length transcript analysis. Consequently, its depth and breadth come with challenges regarding data interpretation and analysis complexity.
SMRT Sequencing
SMRT (Single Molecule, Real-Time) sequencing offers a different approach, emphasizing single-molecule analysis. This technology can read longer RNA sequences, preserving the integrity of entire transcripts. A strong point of SMRT sequencing is its ability to detect methylation modifications during sequencing. This characteristic is particularly beneficial for understanding the influence of RNA methylation on gene expression.
The unique feature of SMRT sequencing lies in its real-time data acquisition, which allows immediate feedback on sequencing results, reducing the time involved in post-sequencing analysis. Nevertheless, this technology can be more expensive and requires complex sample preparation, which can be a drawback for broader application.
Designing RNA Methylation Sequencing Experiments
Sample Preparation
Sample preparation is a critical stage in RNA methylation sequencing. Its importance cannot be overstated, as the quality of RNA extracted can drastically affect downstream results. Proper sample preparation ensures that high integrity and quality RNA can be obtained and utilized for sequencing.
A defining feature of sample preparation is the need for rigorous techniques to isolate RNA from various biological samples. This process must be performed carefully to avoid RNase contamination and degradation, which compromises methylation analysis. While the emphasis on detailed sample preparation can enhance results, it also demands significant time and technical expertise.
Data Collection
Data collection is crucial where meticulous planning is required. It involves organizing the sequencing data so that subsequent analysis can be more efficient and effective. The systematic approach taken during data collection directly influences the ease of data interpretation and can affect the identification of methylation patterns.
A key characteristic of data collection methods is the focus on maintaining accuracy through quality control measures. Implementing these practices allows for improvement in data reliability. However, the complexity of managing large volumes of data can pose challenges. Researchers must continuously refine their collection strategies to ensure consistency and accuracy.
Challenges in Sequencing
The study of RNA methylation sequencing encounters various challenges. Awareness of these issues is necessary for understanding how to optimize methodologies and improve outcomes. Technical limitations and data analysis complications are two primary categories where difficulties arise. Addressing these hurdles ensures that research in RNA methylation can advance efficiently and accurately.
Technical Limitations
RNA methylation sequencing is a complex process that requires advanced technology. There are some technical limitations that researchers face. First, the sensitivity of existing sequencing methods may not always capture low-abundance methylation modifications. Inaccuracies in detection can lead to false negatives or underestimations of methylation levels.
Second, sequencing throughput can sometimes be an issue. Higher throughput is required to analyze larger genomic regions or sample numbers, especially in studies looking for rare or novel methylation marks. The current technologies may restrict researchers from fully exploring their samples, limiting the depth and breadth of their analyses.
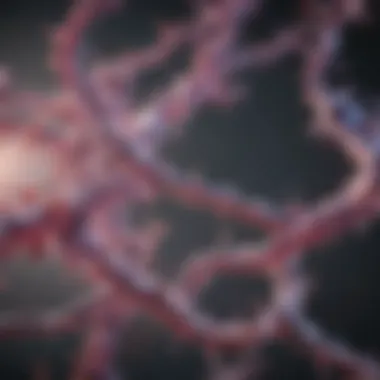
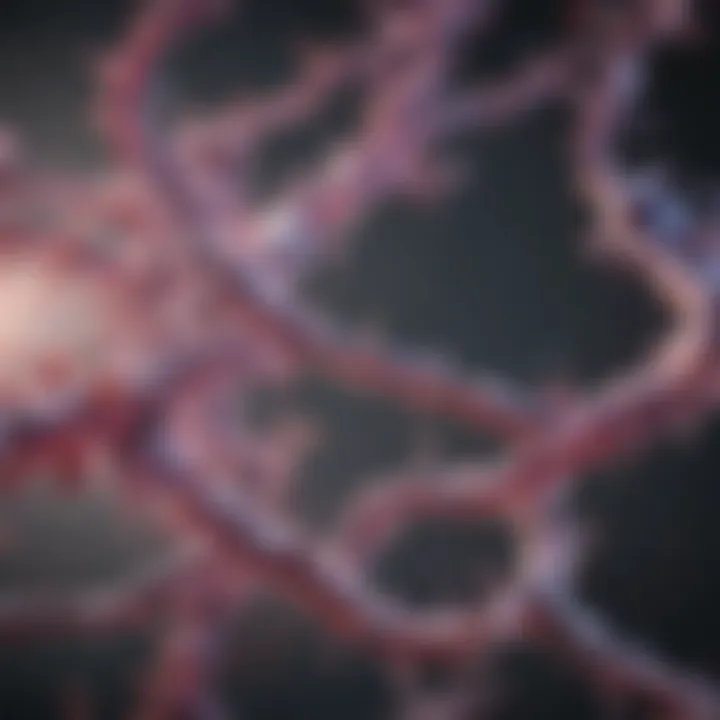
Third, different types of RNA can present compatibility challenges. For instance, messenger RNA (mRNA), long non-coding RNA (lncRNA), and microRNA (miRNA) require distinct approaches for effective sequencing. If the wrong method is applied, crucial modifications could be missed, diminishing the overall quality of the data.
Data Analysis Complications
Once sequencing is completed, the next phase involves analyzing the voluminous data generated. This stage is far from straightforward. There are complexities with data integrity and accuracy that researchers must navigate carefully. With RNA methylation data, variations in read lengths, sequencing errors, and alignment issues can distort results.
Another complication arises from the bioinformatics tools available. Researchers often struggle to choose the right software or method for data processing. Some tools are not optimized for specific methylation marks, leading to gaps in information or misinterpretation of results. Researchers may need substantial computational resources and expertise to handle large datasets effectively.
Moreover, integrating findings from different sources or studies can create additional difficulties. Variability in methodologies among different labs can introduce inconsistency in results. Properly reconciling this data requires careful standardization and validation efforts.
Exhibiting these technical limitations and data analysis complications illuminates a need for improved methodologies within RNA methylation sequencing. By identifying these challenges, ongoing research can focus on refining technology and techniques, ultimately enhancing our understanding of methylation's role in gene expression.
Biological Implications of RNA Methylation
RNA methylation plays a critical role in various biological processes, affecting not only gene expression but also cellular functionality. Understanding its biological implications is essential, as it unveils how cells regulate their activities and respond to environmental cues. In this section, we focus on the importance of RNA methylation in gene regulation and the roles it plays during development and differentiation.
Regulation of Gene Expression
Methylation modifications directly influence how genes are expressed. For instance, m6A methylation, one of the most studied forms, occurs predominantly in messenger RNA (mRNA). This modification impacts mRNA stability, localization, and translation efficiency.
- M6A is associated with destabilizing mRNA, leading to degradation. This process is crucial for controlling the levels of specific proteins within cells.
- The presence of m6A can also promote the binding of particular proteins, thereby enhancing or inhibiting translation.
Moreover, methylation can dictate which genes are active during different life stages. By modulating gene expression, RNA methylation contributes to essential cellular processes such as:
- Cell growth
- Apoptosis (programmed cell death)
- Immune responses
Understanding these mechanisms can help researchers identify potential intervention points for diseases linked to dysregulated gene expression.
Role in Development and Differentiation
During development, RNA methylation serves as a regulatory mechanism that shapes cell fate decisions. Different cell types exhibit distinct methylation patterns that guide their developmental trajectories. The modifications can determine a cell's ability to differentiate into specific lineage types or to maintain its pluripotent state.
For example, studies have demonstrated that alterations in m6A levels can lead to improper signaling pathways, affecting stem cell propagation and differentiation. The implications include:
- Developmental disorders from faulty lineage commitment.
- Cancer; cells may exploit methylation changes to bypass normal growth controls.
Overall, the profound influence of RNA methylation on development underlines its significance in both normal physiology and pathology. As insights into RNA methylation continue to evolve, its role in gene expression regulation and cellular differentiation will likely remain a key focus in molecular biology research.
"Understanding RNA methylation is not just a matter of academic interest; it is fundamental to unraveling the complexities of gene regulation that underpin many diseases."
"Understanding RNA methylation is not just a matter of academic interest; it is fundamental to unraveling the complexities of gene regulation that underpin many diseases."
RNA Methylation and Disease
RNA methylation has emerged as a crucial component in understanding various diseases, particularly cancer and neurological disorders. The ability of methylation modifications to influence gene expression and cellular behavior makes it significant in both pathology and potential treatment options. Through examining the patterns of RNA methylation in disease contexts, we can uncover mechanisms that drive disease progression and identify targets for therapeutic intervention.
Methylation Patterns in Cancer
Cancer is a collection of diseases characterized by uncontrolled cell growth and spread. RNA methylation plays a vital role in regulating gene expression, which can influence tumorigenesis. Abnormal methylation patterns can lead to the silencing of tumor suppressor genes or the activation of oncogenes, impacting cell cycle regulation, apoptosis, and genomic stability.
Examples of RNA methylation linked with cancer include:
- m6A modification: This is one of the most studied types of RNA methylation. Studies indicate that altered levels of m6A methylation are associated with various tumors, including breast cancer and glioblastoma. It affects mRNA stability and translation, which can enhance the aggressiveness of certain cancers.
- m5C modification: Research shows that 5-methylcytosine can also influence cancer cell proliferation and differentiation. Its patterns in certain cancers differ from normal cells, suggesting its potential as a diagnostic biomarker.
- m4C modification: Although less studied, its role is emerging, with implications in the stability and localization of RNA during tumor progression.
Investigating these methylation changes can facilitate the development of targeted therapies. For instance, inhibiting specific demethylases involved in RNA modification could restore function to silenced tumor suppressor genes, providing a novel avenue for treatment.
Neurological Disorders
Neurological disorders, which encompass a wide range of conditions affecting the nervous system, have also been linked to abnormal RNA methylation. Methylation modifications can influence neuronal development, synaptic plasticity, and neuroinflammation.
Key insights into the relationship between RNA methylation and neurological disorders include:
- Alzheimer's Disease: Research indicates that m6A methylation might influence amyloid precursor protein processing and tau phosphorylation. Aberrant regulation of these proteins is critical in Alzheimer's pathogenesis.
- Autism Spectrum Disorders: Certain studies have shown differences in RNA methylation levels in patients with autism. This suggests a potential mechanism where methylation affects neural connectivity and synaptic function.
- Multiple Sclerosis: In this autoimmune disorder, RNA methylation-enriched signaling pathways may modulate immune responses contributing to demyelination and neurodegeneration.
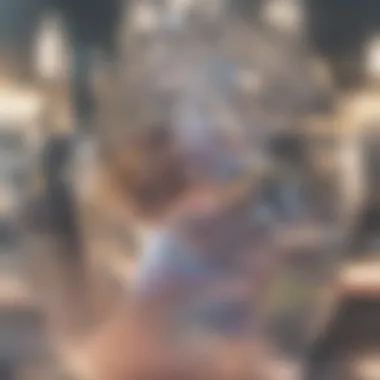
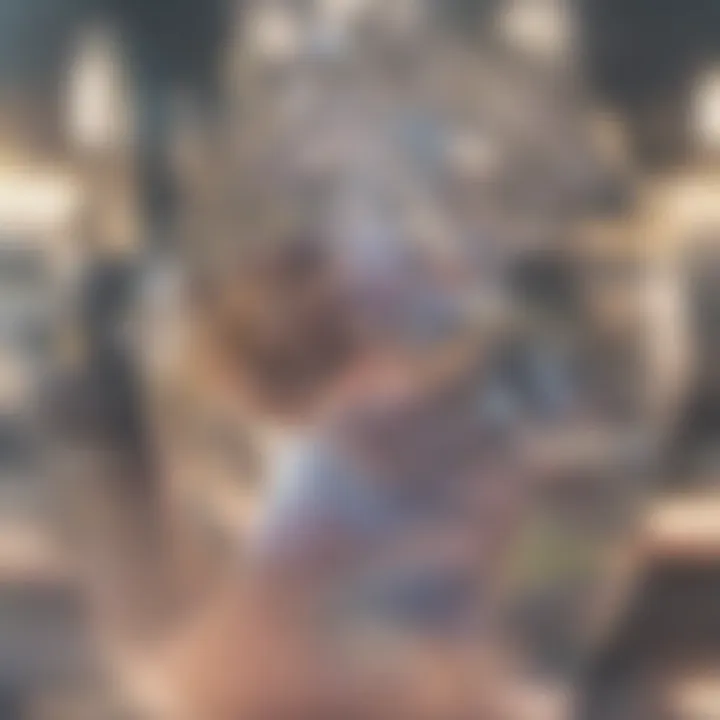
Incorporating the study of RNA methylation in these disorders provides an essential piece to the puzzle. It opens up possibilities for new therapeutic strategies, allowing for early detection and potentially effective treatments to mitigate disease progression.
Understanding the nuances of RNA methylation patterns can revolutionize our approach to treating diseases, enhancing our grasp of their underlying biological mechanisms.
Understanding the nuances of RNA methylation patterns can revolutionize our approach to treating diseases, enhancing our grasp of their underlying biological mechanisms.
Applications of RNA Methylation Sequencing
RNA methylation sequencing has gained significant prominence in recent years due to its vast potential in molecular biology and medicine. Understanding the applications of this sequencing technique reveals its critical role in advancing research on gene expression and various diseases. This section highlights key applications, emphasizing therapeutic targets and the identification of biomarkers for disease, which are essential for improving health outcomes.
Therapeutic Targets
RNA methylation represents an important regulatory layer that influences gene expression, making it a promising avenue for therapy. Numerous diseases result from dysregulation of mRNA methylation patterns. Identifying specific methylation marks can provide insights into new therapeutic targets. Researchers can conduct targeted therapies aimed at correcting abnormal methylation processes. For example, in certain cancers, aberrations in m6A methylation can lead to uncontrolled cell proliferation. By restoring normal methylation levels through targeted interventions, it might be possible to halt tumor growth and improve patient prognosis.
Moreover, small molecules that inhibit or enhance methyltransferases or demethylases are being developed. These compounds have the potential to directly modify the RNA methylation landscape, leading to altered gene expression profiles. Consequently, they could be pivotal in treating diseases related to RNA methylation abnormalities like cancer and autoimmune disorders.
"Target identification through RNA methylation sequencing can lead to revolutionary therapies, transforming how we approach disease treatment."
"Target identification through RNA methylation sequencing can lead to revolutionary therapies, transforming how we approach disease treatment."
Biomarkers for Disease
Another critical application of RNA methylation sequencing lies in its utility for identifying biomarkers for disease. Methylation patterns in RNA can serve as indicators of various health conditions or disease states. By analyzing these patterns, researchers can differentiate between healthy and diseased tissues, leading to earlier diagnosis and more personalized treatment approaches.
For instance, studies have shown that specific m6A modifications correlate with the progression of certain cancers. By developing assays based on these modifications, clinicians could potentially monitor disease progression or response to therapy. Furthermore, since RNA methylation is often tissue-specific, profiling can provide valuable information about the disease context, enhancing both diagnostics and therapeutic targeting.
In summary, applications of RNA methylation sequencing are diverse and impactful. They encompass the identification of therapeutic targets and the discovery of disease biomarkers, both crucial for advancing personalized medicine. As our understanding of RNA methylation deepens, so too will the potential for innovative research and clinical applications.
Future Directions in Research
The consideration of future directions in RNA methylation research is increasingly crucial, given its implications in numerous biological processes and diseases. As research evolves, new pathways and applications are emerging. Understanding these future trends can provide insights into how RNA methylation can influence both basic science and applied medical fields. The focus is not only on refining existing techniques but also on innovating new methodologies that can provide more comprehensive data.
Emerging Techniques
Emerging techniques in RNA methylation sequencing are central to advancing our understanding of this field. Technologies such as CRISPR/Cas9-based methods are being adapted to explore methylation patterns more effectively. Also, advancements in single-cell RNA sequencing (scRNA-seq) are allowing researchers to examine methylation at the individual cell level, revealing subtle variations previously obscured in bulk analyses.
Another promising area is nanopore sequencing. It allows for real-time sequencing and identification of methylation marks as the RNA passes through the nanopore. This could streamline the process of identifying relevant methylation modifications without extensive preprocessing of samples. Furthermore, improvements in bioinformatics tools are essential to interpret vast datasets generated by these new technologies. These advances make it possible to correlate methylation alterations with specific phenotypes or disease states.
Integrating Multi-Omics Approaches
Integrating multi-omics approaches marks a significant leap in RNA methylation research. Combining genomics, transcriptomics, proteomics, and metabolomics can provide a holistic view of cellular functions. This allows researchers to understand not only how RNA methylation influences gene expression but also how those changes translate into protein production and metabolic activity.
For example, linking methylation data with transcriptome information can uncover regulatory networks that are otherwise hard to see. It can highlight the interactions between different molecules and pathways, aiding in disease understanding and drug development.
Collaborative efforts between disciplines—such as biology, computational science, and chemistry—are critical in this integration. These collective insights can lead to more effective therapeutic strategies, particularly in cancer and other complex diseases where multiple molecular factors are at play.
Future research should emphasize the integration of emerging technologies with a multi-omics perspective, aiming to unveil previously obscured layers of biological regulation.
Future research should emphasize the integration of emerging technologies with a multi-omics perspective, aiming to unveil previously obscured layers of biological regulation.
Closure
In this article, we have extensively explored RNA methylation sequencing and its substantial role in understanding the complexities of gene regulation and cellular processes. The conclusion serves as a vital component of our discussion, summarizing key insights while emphasizing the implications of continued research in this dynamic field.
Summary of Insights
RNA methylation has emerged as a crucial mechanism influencing gene expression. We delved into various types of methylation modifications, such as m6A, m5C, and m4C, highlighting their distinct roles and significance. Understanding the enzymatic processes underlying these modifications, including the functions of methyltransferases and demethylases, adds depth to how we perceive RNA biology.
The advancements in sequencing technologies like RNA-Seq and SMRT sequencing have transformed our approach to studying these methylation patterns. The ability to design robust experiments for RNA methylation sequencing can increase reliability and reproducibility in research, providing clearer insights into the biological implications of RNA methylation in areas such as development, differentiation, and disease manifestations.
The Importance of Continued Research
As RNA methylation and its role in various diseases comes into sharper focus, ongoing research is essential. The integration of emerging techniques and multi-omics approaches can facilitate a deeper understanding of the connections between RNA modifications and health outcomes. It is vital for initiatives that investigate methylation patterns, especially in diseases like cancer and neurological disorders, to continue to be pursued.
The potential for therapeutic applications is considerable, with RNA methylation sequencing offering pathways to discover new biomarkers and identify therapeutic targets. Hence, continuous exploration can lead to novel clinical applications that may alleviate disease impacts.
"Understanding RNA methylation is not only pivotal for basic research but also for enhancing diagnostic and therapeutic strategies in modern medicine."
"Understanding RNA methylation is not only pivotal for basic research but also for enhancing diagnostic and therapeutic strategies in modern medicine."
In summation, the conclusions drawn throughout this article underscore the critical need for ongoing studies in RNA methylation sequencing. Staying informed and engaged in this field can lead to breakthroughs that benefit both scientific understanding and human health.