Thermal Conductivity in Silicon Wafers: Key Insights
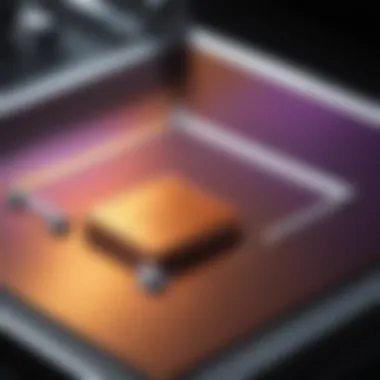
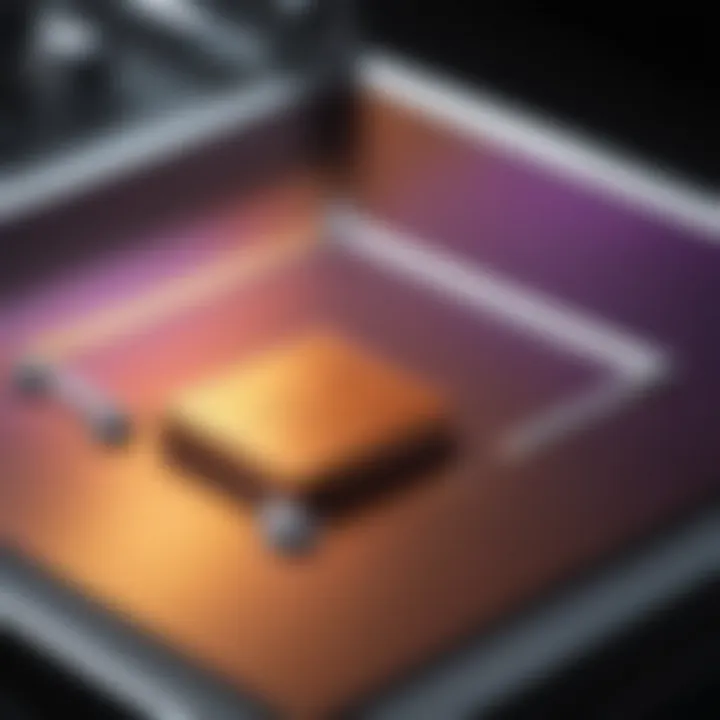
Intro
Understanding thermal conductivity in silicon wafers is not just an academic exercise; it has real-world implications across a variety of fields. This dynamic property of materials dictates how heat transfers through substances, and in the case of siliconāan essential backbone of modern electronicsāits influence cannot be overstated. Silicon wafers, which form the cornerstone of semiconductor devices, play a pivotal role in heat management. From microprocessors to solar cells, the capability of these wafers to manage heat affects performance and longevity of devices.
Siliconās conductivity is determined by numerous factors, including purity, temperature, and structural quality. These elements combine in intricate ways to dictate how effectively heat moves through a wafer. For instance, an increase in temperature may enhance lattice vibrations, thus increasing thermal conductivity to a certain threshold before reaching saturation.
Conversely, as ions or defects introduce variability, they alter the predicted behavior, creating complexities for engineers to navigate. As semiconductor technology progresses, the demand for better thermal management increases, prompting ongoing studies into the thermal properties of silicon wafers.
In this article, we shall embark on a comprehensive journey exploring these properties, starting from the fundamental principles, through historical contexts, to current applications and future trends. Our aim is to provide a nuanced understanding that connects theory with practice in the rapidly advancing world of materials science and electronics.
Preface to Silicon Wafers
Silicon wafers are fundamental to modern electronics, acting almost like the backbone of the devices we engage with daily. Without a good grasp of their structure, functionality, and the role they play, it's hard to appreciate the innovation powering our technology. In this article, we will explore the significance of silicon wafers, particularly in the context of thermal conductivity, which has far-reaching implications in the realm of electronics and materials science.
Understanding silicon not only involves its sheer utility but also the characteristics that define its use in various applications. As components continue to shrink and efficiency demands increase, comprehending how thermal conductivity influences silicon wafers is vital for researchers and professionals in physics and engineering.
Overview of Silicon as a Semiconductor
Silicon itself is the second most abundant element in the Earthās crust, making it relatively easy to source. More importantly, its properties as a semiconductor are unparalleled. Semiconductors are materials that can conduct electricity under certain conditions while acting as an insulator under others, allowing them to be transformed into āswitches.ā
Here are some standout features of silicon as a semiconductor:
- Band Gap Property: Silicon has a band gap of 1.1 eV, which means that at room temperature, it can easily transfer electrons when excited by heat or light.
- Doping Potential: By incorporating impurities such as phosphorus or boron, one can effectively modify its electrical characteristics, tailoring the performance for specific applications.
- Thermal Stability: Silicon remains chemically stable across a wide range of temperatures, allowing its use in various electronic applications.
These inherent properties make silicon a prime candidate in the manufacturing of complex devices like transistors, diodes, and integrated circuits. Being familiar with these aspects gives us a solid foundation to understand how thermal conductivity behaves and its necessity in silicon wafers.
Role of Silicon Wafers in Electronics
At its core, the role of silicon wafers stretches from serving as the substrate for integrated circuits to functioning as the base for solar cells. The transition of raw silicon into wafers involves an intricate and precisely controlled process, with the end product being a circular slice of silicon, measuring just a few millimeters in thickness yet critically important in functionality.
Some key roles of silicon wafers include:
- Foundation for Integrated Circuits: The wafers provide a platform where components like capacitors and resistors can be fabricated, ultimately leading to complex chips.
- Photovoltaic Applications: In solar cells, silicon wafers convert sunlight into energy; thus, their thermal characteristics can directly influence efficiency and performance.
- Heat Dissipation: Effective heat management is crucial in semiconductor devices: silicon wafers' thermal conductivity assists in dissipating heat generated during operation, preventing overheating.
"The excellence of silicon as a semiconductor derives from its ability to manage both electricity and heat efficiently, a duality necessary in the tiny devices that power our lives."
"The excellence of silicon as a semiconductor derives from its ability to manage both electricity and heat efficiently, a duality necessary in the tiny devices that power our lives."
In summary, silicon wafers hold profound importance not just as basic components in electronics but also across various innovative technologies. Their role, dictated by intrinsic properties and external factors, makes them indispensable in our ever-evolving tech landscape.
Understanding Thermal Conductivity
Thermal conductivity is a pivotal property that significantly influences the performance and applications of silicon wafers. This property dictates how effectively heat moves through materials, and in the context of silicon, it is critical for ensuring optimal function in electronic devices. This section aims to explore the definition of thermal conductivity, its importance, and the fundamental principles of heat transfer, framing aluminum's conductivity characteristics within various applications.
Definition and Importance of Thermal Conductivity
Thermal conductivity refers to the ability of a material to conduct heat. It is quantified by the thermal conductivity coefficient, expressed in watts per meter kelvin (W/mĀ·K). In simpler terms, it tells one how well a substance can transfer thermal energy. For silicon wafers, a high thermal conductivity translates into efficient heat dissipation, which is essential for maintaining performance and prolonging the lifespan of electronic components.
Some key points to consider include:
- Efficiency: High thermal conductivity means better heat dissipation in semiconductor devices. This efficiency is especially crucial in high-performance applications like processors, where excess heat can lead to performance degradation or failure.
- Material Selection: Knowledge of thermal conductivity is vital in selecting materials for fabrication processes. Materials with suitable thermal properties reduce thermal resistance, which is a considerable factor in device performance.
- System Integration: Understanding thermal conductivity can optimize thermal management systems, leading to better integration of silicon wafers into electronic devices.
This insistence on the significance of thermal conductivity isnāt just academic; industry professionals often cite it as a crucial parameter shaping the evolution of semiconductor technology.
Basic Principles of Heat Transfer
Heat transfer involves three main mechanisms: conduction, convection, and radiation. Each plays a role in how heat moves through silicon wafers and other materials, impacting thermal conductivity:
- Conduction: This is the primary mode of heat transfer in solids, including silicon. Heat travels through the lattice structure of the material, where atoms collide and transfer energy to neighboring atoms. Siliconās crystalline structure is adept at facilitating this energy transfer, contributing to its favorable conductivity.
- Convection: This mechanism comes into play when heat is transferred through fluids (liquids or gases). While not pertinent to silicon itself, understanding convection is beneficial in the context of heat management systems wherein silicon wafers operate. For example, heat sinks designed to dissipate heat need to account for airflow around silicon devices.
- Radiation: Although less common compared to the other two in semiconductor applications, it still deserves mention. Heat can also flow via electromagnetic wavesāparticularly relevant in scenarios involving high temperatures or vacuum environments.
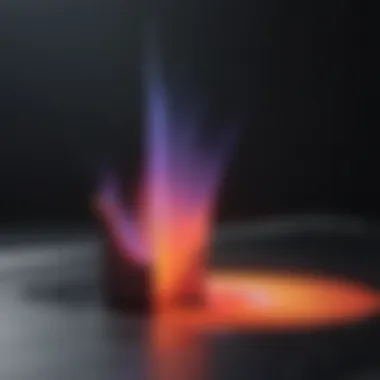
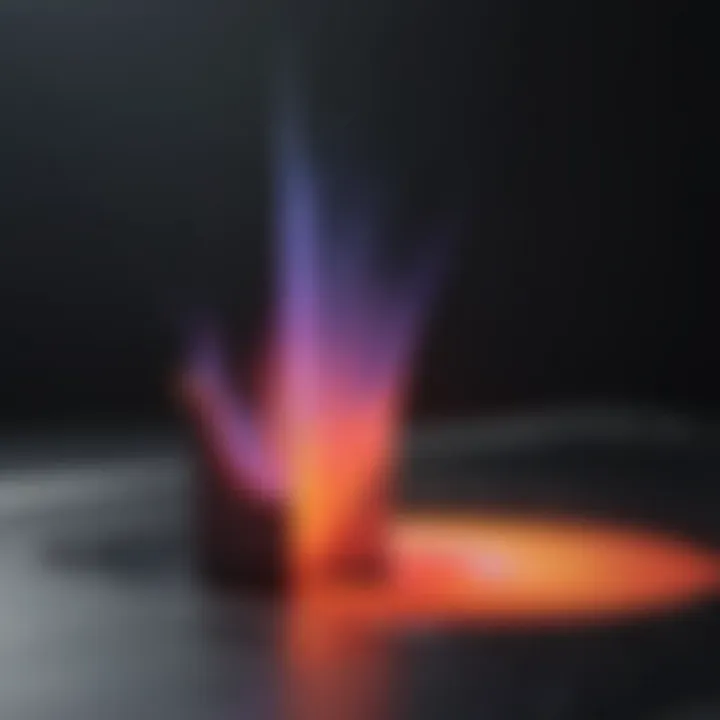
"Understanding the interplay of these heat transfer mechanisms is essential for engineers and scientists working with thermal management in electronics."
"Understanding the interplay of these heat transfer mechanisms is essential for engineers and scientists working with thermal management in electronics."
In summary, grasping thermal conductivity and the principles behind heat transfer is vital for optimizing the performance and durability of silicon wafers. It opens the door to a myriad of applications in electronic devices, enhancing both their operation and efficiency. This foundational knowledge forms a backdrop for deeper exploration into how these properties affect real-world device functionality and application.
Thermal Conductivity in Silicon
Understanding thermal conductivity in silicon wafers is crucial for several reasons. Silicon, as a commonly used semiconductor material, plays a significant role in numerous electronic devices ranging from smartphones to complex computational servers. Given the vital function of thermal management in ensuring the performance and longevity of these devices, comprehending how heat is transferred through silicon becomes a key focus area for researchers and engineers alike.
One of the primary elements contributing to thermal conductivity is the intrinsic properties of silicon itself. Knowledge about its atomic structure and inherent characteristics paves the way for better designs and more efficient devices. Additionally, recognizing the various factors that influence thermal conductivity helps in optimizing processes where heat management is paramount.
Intrinsic Properties of Silicon
Silicon possesses unique intrinsic properties that directly contribute to its high thermal conductivity. The crystal lattice structure of silicon allows for efficient phonon transport, which is essential in thermal conduction. Phonons are essentially quantized modes of vibrations that carry heat through materials. In silicon, the availability of silicon atoms arranged in a tetrahedral structure significantly promotes energy transfer.
Moreover, the bond strength in silicon is particularly notable; the strong covalent bonds between silicon atoms enable it to withstand high temperatures while effectively conducting heat. This property makes silicon a preferred choice in a range of applications that call for temperature stability coupled with efficient heat dissipation.
Factors Affecting Thermal Conductivity in Silicon Wafers
The thermal conductivity of silicon wafers isn't constant and is influenced by several key factors. Understanding these factors is essential for improving device efficiency and performance.
Crystal Structure
The crystal structure of silicon plays a pivotal role in its thermal conductivity. The crystalline arrangement allows for orderly atomic vibrations, facilitating the smooth flow of heat. For silicon, a diamond cubic crystal structure creates ample pathways for phonon interaction.
A noticeable characteristic of this structure is its high degree of symmetry, which aids in maximizing thermal transport across the wafer. This quality positions silicon as a popular choice in the production of integrated circuits, where efficient heat management is integral to performance.
Advantages:
- Efficient Heat Transfer: The symmetry of the structure aids in faster heat conduction.
- Low Thermal Resistance: Minimizes heat build-up in electronic devices.
Disadvantages:
- Anisotropy: Variation in thermal conductivity can occur depending on the crystallographic direction, which can complicate certain applications.
Doping Effects
Doping is the process of intentionally introducing impurities into silicon to modify its electrical and thermal properties. The doping effects have a direct correlation with thermal conductivity. When certain atoms are addedāsuch as phosphorus or boronāthis can either enhance or impede thermal transport.
A key characteristic here is how the dopants affect carrier concentrations. While moderately doped silicon can exhibit improved thermal conductivity due to increased phonon scattering, excessive doping might inhibit heat flow. This balance is crucial to maintaining optimal thermal performance in devices.
Advantages:
- Customization: Allows fine-tuning of silicon properties for specific electronic applications.
- Enhanced Performance: Appropriate doping facilitates better heat management in semiconductors.
Disadvantages:
- Potential Degradation: Over-doping can lead to increased defects and reduced thermal conductivity.
Temperature Dependency
The temperature dependency of silicon's thermal conductivity is another critical factor to consider. In general, thermal conductivity tends to decrease as temperature increases due to enhanced scattering of phonons at elevated temperatures.
Silicon exhibits a characteristic trend where, at lower temperatures, the conductivity is higher. This happens because the lattice vibrations are minimal, allowing phonons to transfer heat with fewer disruptions. Understanding this dependency helps engineers predict how a silicon wafer will behave in different thermal environments.
Advantages:
- Predictable Behavior: Easier to model device performance under varying thermal conditions.
- Optimized Design: Allows developers to tailor systems based on thermal thresholds.
Disadvantages:
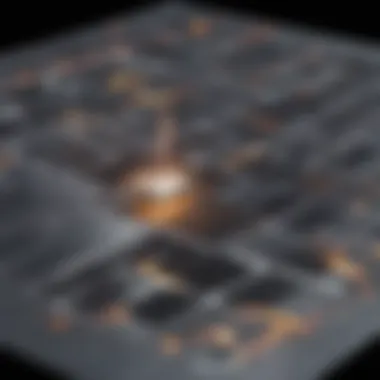
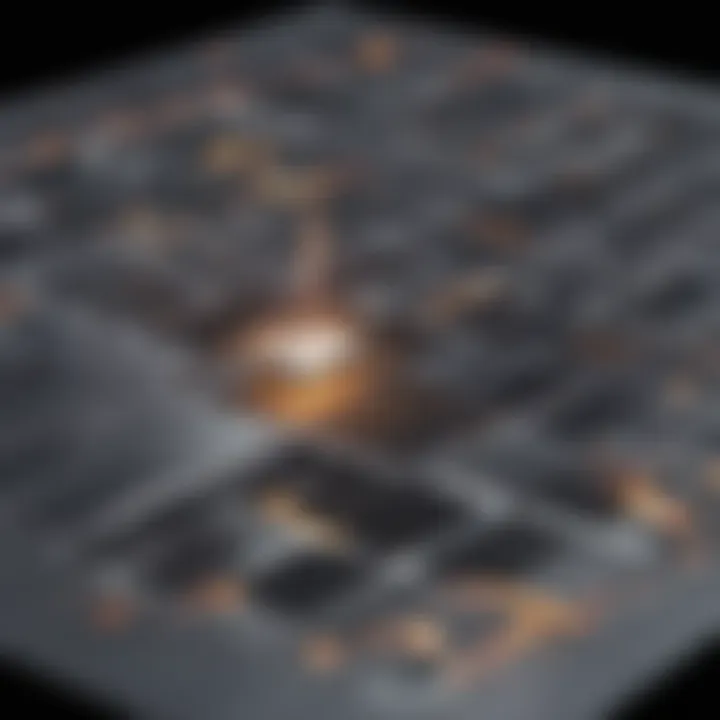
- Performance Variability: At higher temperatures, poor thermal conductivity can limit the performance of electronic devices.
Measuring Thermal Conductivity of Silicon Wafers
Measuring the thermal conductivity of silicon wafers is crucial for understanding their performance in electronic applications. As silicon serves as the backbone of modern electronicsāfrom microprocessors to solar cellsāaccurate thermal conductivity measurements can lead to better designs and innovations within the industry.
The importance of measuring these properties does not lie solely in theoretical knowledge; it has practical implications too. For instance, in power devices, managing heat dissipation is a key factor in improving efficiency and lifespan. Therefore, knowing how silicon wafers transfer heat allows engineers and researchers to innovate better thermal interface materials and cooling systems.
Techniques for Measurement
Laser Flash Analysis
Laser Flash Analysis (LFA) is a widely adopted technique for assessing thermal conductivity, particularly in the semiconductor realm. This method involves a brief laser pulse that heats one side of a sample. Sensors on the opposite face measure the temperature rise over time, allowing for calculations of thermal conductivity based on the sample's response to the heat.
The key characteristic of LFA is its speed; it can provide results in a matter of seconds, making it a go-to option for labs needing rapid assessments. One of the unique features of LFA is that it requires minimal sample preparation, making it a practical choice for many forms of silicon wafersāregardless of their thickness. However, its limitations include the need for transparent or semi-transparent materials, which can restrict its application in certain types of silicon samples.
Hot Disk Method
The Hot Disk Method involves inserting a thin sensor in thermal contact with the silicon wafer being measured. This sensor acts as both a heat source and a thermometer. As it heats up, the thermal conductivity of the material is inferred from the speed at which the heat spreads.
What stands out about the Hot Disk Method is its versatility; it can measure thermal conductivity in solids, liquids, and gases. Plus, this method is particularly beneficial for working with small samples. However, the challenge here lies in achieving optimal contact between the sensor and the wafer, which can skew results if not done properly.
Fourier Transform Infrared Spectroscopy
Fourier Transform Infrared Spectroscopy (FTIR) is another technique gaining attention for measuring thermal properties. Unlike the earlier methods, FTIR leverages infrared light to analyze the material responses and can detect thermal conductivity indirectly by examining vibrational characteristics.
A notable advantage of FTIR is its ability to provide molecular-level insights, helping researchers understand how siliconās structure correlates with thermal conductivity. However, this method requires sophisticated equipment and can be time-consuming compared to simpler techniques like LFA. Furthermore, interpreting the results might demand expertise in spectroscopy.
Challenges in Measurement
Despite advances in measurement techniques, specific challenges persist that can complicate the assessment of thermal conductivity in silicon wafers.
Sample Preparation
Proper sample preparation is vital for accurate measurement, yet it often poses significant hurdles. Flaws such as surface irregularities, improper dimensions, or contamination can lead to inaccurate thermal conductivity readings.
The primary challenge here lies in maintaining consistency across samples. High-quality, uniform samples ensure that measurement results reflect true thermal conductivity rather than variations due to physical defects or environmental conditions. On the other side, this meticulous preparation can be resource-intensive, demanding both time and effort.
Environmental Factors
Environmental factors also play a crucial role in how effectively thermal measurements can be made. Temperature fluctuations, humidity, and ambient pressure can all cause variations in thermal readings.
This sensitivity to surroundings often reduces the reliability of results unless controlled lab environments are employed. The challenge is that achieving such tuned conditions can be costly, which may not always be feasible for smaller labs or academic institutions. Thus, researchers must navigate this landscape carefully, balancing the need for precise environmental control against available resources.
Measuring thermal conductivity is not just a technical necessity; it's a crucial ingredient for improving performance in semiconductor technology.
Measuring thermal conductivity is not just a technical necessity; it's a crucial ingredient for improving performance in semiconductor technology.
By addressing these challenges, researchers can refine their techniques for measuring thermal conductivity in silicon wafers, leading to enhanced applications and innovations across various electronic devices.
Applications of Thermal Conductivity in Silicon Wafers
In the realm of electronics, thermal conductivity in silicon wafers plays an essential role. It serves as the backbone for heat management in various devices, ensuring these components function optimally without overheating. High thermal conductivity ensures efficient performance and reliability in semiconductor devices, which is why understanding its applications is paramount.
Heat Management in Semiconductor Devices
To keep semiconductor devices running smoothly, effective heat management is a must. This particularly includes the use of thermal interface materials and heat sinks. These components work together to reduce thermal resistance, thereby facilitating efficient heat dissipation. Without them, we could see a drastic decrease in device lifespan and overall performance.
Thermal Interface Materials
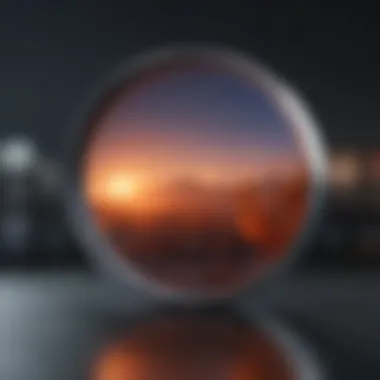
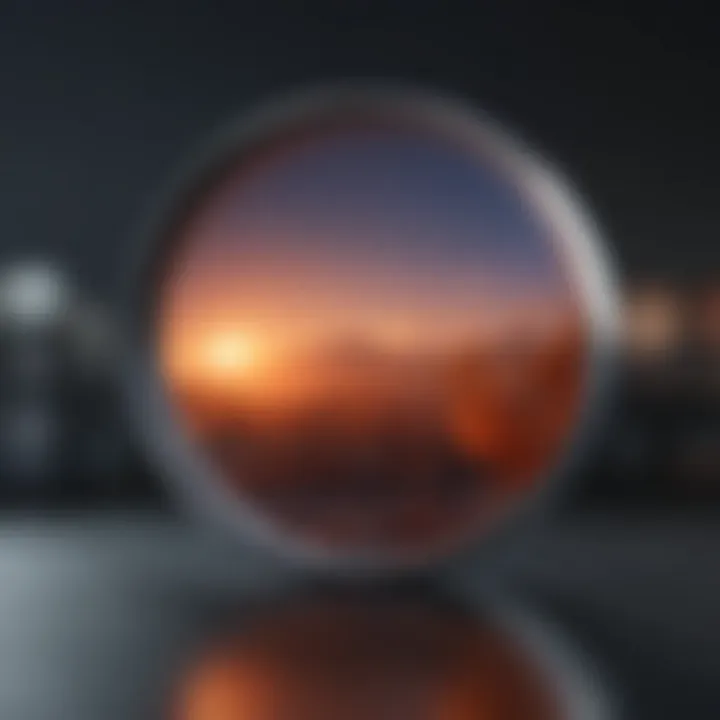
Thermal interface materials (TIMs) are fundamental in transferring heat between semiconductor chips and heat sinks. Their key characteristic lies in their ability to minimize the thermal boundary resistance. TIMs are often made of materials like silicone, which is both effective and flexible, allowing them to fill microscopic air gaps that would otherwise hinder heat flow.
Thereās a reason why TIMs are popular in this field. They boost thermal conductivity significantly more than air alone could ever do. The unique feature of TIMs is their adaptability to various substrates, which provides versatility across different electronic applications. However, one might argue they can be a bit expensive and prone to degradation over time, which is something to keep in mind when selecting them for a specific application.
Heat Sinks and Spreaders
Heat sinks and spreaders are also critical in managing heat produced by semiconductor devices. These components are designed to absorb and dissipate excess heat, which is crucial for maintaining performance levels. Typically, these are constructed from materials like aluminum or copper, known for their excellent thermal conductivity.
The primary advantage of heat sinks is their ability to prolong device life by maintaining optimal operating temperatures. A unique feature of advanced heat sinks is their design, which often incorporates fins or other configurations to enhance airflow and thus thermal dissipation efficiency. However, the downside could be size and weight, which might not fit compact device layouts.
Impact on Device Performance
How does thermal conductivity affect device performance? The short answer: significantly. If thermal management isn't handled properly, it can lead to issues like thermal throttling, where a device slows down to avoid overheating. This is where transistor functionality and integrated circuit efficiency come into play.
Transistor Functionality
Transistors are crucial for regulating the flow of electric current, and their performance heavily relies on effective thermal management. A lesser-known characteristic of transistors is their sensitivity to temperature variations. When they get too hot, their functionality can drop, causing failures in the circuit. This is why maintaining proper thermal conductivity is essential; it allows transistors to operate within their designed specifications, ensuring modest performance and reliability. An advantage of managing thermal conditions around transistors is the potential for higher frequencies and faster operations, which are increasingly essential in modern electronics.
Integrated Circuit Efficiency
Integrated circuits (ICs) showcase a similar relationship with thermal conductivity. These complex assemblages of circuits rely on efficient heat management to maintain optimal performance. A key notable aspect is that as power density increases in modern designs, the need for superior thermal management becomes even more vital.
With improved thermal conductivity, integrated circuits can handle greater loads without self-destructing under high temperatures. However, the unique challenge lies in balancing cooling requirements with size constraintsāsomething that engineers wrestle with in design.
Recent Advancements in Silicon Wafer Technology
Recent advancements in silicon wafer technology have ushered in a new era in electronics and materials science. Innovations in this domain not only enhance the efficiency of electronic devices but also optimize their thermal performance, especially as the industry continues to push for smaller, faster, and more powerful components.
Innovations in Material Composition
The composition of silicon wafers has undergone significant changes over the years, driven by the need for better thermal conductivity and performance. Here are some crucial innovations:
- Doped Silicon: By carefully introducing impurities such as phosphorus or boron, manufacturers can alter the thermal properties of silicon wafers. This doping process can boost thermal conductivity, while also tailoring electrical properties to suit specific applications.
- Silicon Carbide and Other Alloys: Silicon carbide (SiC) is emerging as a favored alternative to traditional silicon. Its higher thermal conductivity makes it invaluable for high-power, high-temperature applications. Furthermore, research into silicon-germanium (SiGe) alloys is uncovering potential pathways for better heat management in advanced devices.
- Composite Materials: Recent developments show an increased use of composite materials that blend silicon with polymers or metals. This combination can lead to materials that possess desirable mechanical properties while maintaining effective thermal management capabilities.
These innovations are crucial for solving thermal issues in increasingly miniaturized devices, enabling engineers to design configurations that manage heat without sacrificing performance or creating thermal bottlenecks.
Future Trends in Thermal Management Solutions
The landscape of thermal management in silicon wafer technology is continuously evolving. Here are some trends that are expected to shape its future:
- Advanced Heat Spreader Technologies: Thereās a rising trend to integrate more advanced heat spreaders which utilize novel materials for improved thermal conductivity. These can enhance the reliability of devices in demanding environments.
- 3D Packaging Solutions: As electronics shrink in size, 3D packaging solutions are gaining traction. This approach enables the stacking of multiple chip layers, which presents new challenges for thermal management. Designers are now looking for adaptive materials and techniques to dissipate heat effectively within these compact systems.
- Integration of Monitoring Systems: Future silicon wafers are expected to incorporate embedded thermal sensors that track temperature changes in real-time. This would allow for dynamic thermal management, automatically adjusting system operations to prevent overheating.
"In the race toward miniaturization of semiconductor devices, heat management must be as innovative as the chips themselves."
"In the race toward miniaturization of semiconductor devices, heat management must be as innovative as the chips themselves."
These movements in silicon wafer technology underscore the industry's commitment to not only pushing the boundaries of performance but also ensuring the longevity and resilience of electronic devices. Each innovation brings us closer to achieving high-performance systems that withstand the rigors of modern demands.
The End
In any exploration of thermal conductivity in silicon wafers, drawing a conclusion offers a crucial opportunity to consolidate knowledge acquired throughout the discussion. The investigation into this topic elucidates not only the scientific foundations that govern thermal conductivity but also its practical implications in the modern technological landscape.
Understanding thermal conductivity in silicon wafers holds significant relevance for multiple areas, primarily within electronics, where performance is critically tied to thermal management. Silicon wafers act as essential components in semiconductor devices, and their ability to transfer heat efficiently can impact device lifespan, reliability, and overall functionality. As industries push the envelope in terms of performance and miniaturization, the ability to effectively manage heat becomes paramount.
Additionally, the insights garnered through recent advancements in measuring techniques and material properties enhance our ability to design next-generation devices. The scope of research highlighted, such as the exploration of innovative materials and new applications for thermal interface materials, suggests avenues that could lead to breakthroughs benefitting various sectors of technology and engineering. The ongoing commitment to improving our understanding of thermal conductivity can potentially offer avenues for significant efficiency gains in existing processes or even pave the way for entirely new applications.
Summation of Key Points
- Intrinsic Importance: The thermal conductivity of silicon wafers is integral for efficient semiconductor device performance.
- Technology Impact: Better heat management translates to enhanced operational efficiency and longevity in electronics.
- Innovative Measurement Techniques: New methodologies contribute to more accurate assessment of thermal properties, improving our understanding and application of these materials.
- Future Applications: Research into novel materials and improved designs can lead to better heat management strategies, further optimizing device performance in various fields.
Future Directions in Research
Potential lines of inquiry present exciting possibilities for researchers in this area. Here are some future research directions:
- Composite Materials: Investigating new composite silicon materials that could enhance thermal properties beyond those of standard wafers.
- Nano-Engineering: Exploring the role of nanostructures in thermal management performance in silicon wafers.
- Sustainability: Researching more sustainable production methods for silicon wafers, which not only meet the technical specifications but also reduce environmental impact.
- Thermal Interface Innovations: Developing advanced thermal interface materials that can bridge gaps more effectively than existing options, thereby improving overall efficiency.
In light of these considerations, it becomes clear that the field is ripe for exploration. As professionals and academics continue to examine thermal conductivity in silicon wafers, they find opportunities to contribute meaningfully to advancements within the industry while addressing broader societal and environmental concerns.