Understanding Thermoelectric Chargers: An In-Depth Review
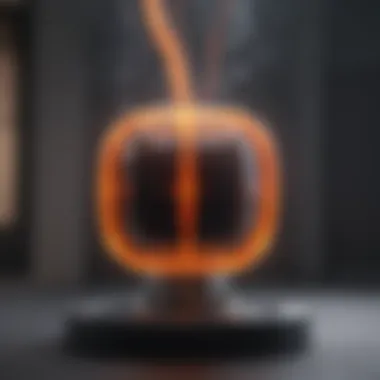
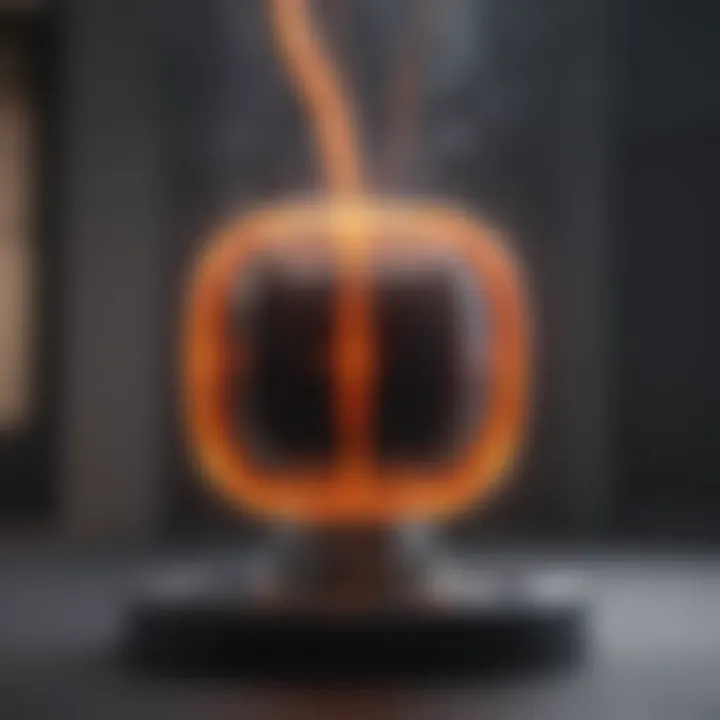
Intro
Thermoelectric chargers represent an innovative solution to energy generation by utilizing temperature gradients. They have the capacity to convert heat into electricity, providing an accessible means of harnessing energy from waste heat and other temperature differentials. Understanding how these devices operate is increasingly relevant in today’s world, where energy efficiency and sustainable practices are vital priorities across multiple industries. This overview seeks to elucidate the principles, materials, and applications surrounding thermoelectric technology, offering insights for students, researchers, and professionals.
Article Overview
Purpose of the Article
The purpose of this article is to provide a thorough exploration of thermoelectric chargers. By dissecting their functionalities and operational principles, this article showcases how these technologies can play a significant role in various domains. Attention will also be given to recent advancements in materials, efficiency improvements, and novel applications that highlight the future potential of thermoelectric solutions.
Relevance to Multiple Disciplines
Thermoelectric chargers intersect various fields such as physics, engineering, and environmental science. This cross-disciplinary relevance underscores their importance. They are not limited to one sector; rather, they hold promise in:
- Renewable energy generation
- Waste heat recovery
- Space exploration
- Consumer electronics
The implications extend to sustainability efforts globally. Various industries can benefit from understanding and implementing these technologies.
Research Background
Historical Context
The foundation of thermoelectric materials can be traced back over a century. The discovery of the Seebeck effect by Thomas Johann Seebeck in 1821 marked the inception of thermoelectric research. In the ensuing decades, scientists developed materials that could efficiently convert thermal energy into electrical energy. Throughout the 20th century, advancements in material science opened avenues for enhancing the thermoelectric efficiency of various materials.
Key Concepts and Definitions
To fully grasp thermoelectric technology, a few fundamental concepts need to be defined:
- Seebeck Effect: The phenomenon where a temperature difference between two different materials generates an electric potential.
- Peltier Effect: The reverse process, where an electric current creates a temperature difference across materials.
- Thermoelectric Efficiency: Often discussed in terms of the dimensionless figure of merit (ZT), a measure that evaluates the performance of thermoelectric materials.
Understanding these concepts lays the groundwork for delving deeper into how thermoelectric chargers function and their potential applications.
"Thermoelectric technology not only focuses on energy conversion but also aims to enhance sustainability by utilizing ambient heat sources."
"Thermoelectric technology not only focuses on energy conversion but also aims to enhance sustainability by utilizing ambient heat sources."
This overview sets the stage for a comprehensive discussion on thermoelectric chargers, their workings, materials, advantages, and future prospects.
Prolusion to Thermoelectric Chargers
Thermoelectric chargers represent a fascinating intersection of energy conversion and sustainable technology. These devices harness temperature differences to generate electrical energy, making them a crucial element in the modern quest for efficient energy solutions. Given the rising demands for portable power sources and renewable energy options, understanding thermoelectric chargers becomes ever more pertinent.
Definition and Functionality
Thermoelectric chargers operate based on the principles of thermoelectricity, a field that focuses on the direct conversion between thermal energy and electrical energy. When two different materials are subjected to a temperature differential, an electric voltage is produced. This principle is primarily founded on the Seebeck effect, which is central to the functionality of thermoelectric devices. Such chargers can be applied in multiple environments, ranging from small personal electronics to larger industrial systems. The appeal of these devices lies not only in their functionality but also in their potential for promoting more sustainable energy practices.
Historical Context
The origins of thermoelectric technology trace back to the early 19th century, with significant contributions from scientists such as Thomas Johann Seebeck and Jean Charles Athanase Peltier. These foundational discoveries paved the way for the development of thermoelectric materials and devices that would see practical applications throughout the 20th and into the 21st century. The need for alternative energy sources, especially in remote areas or during energy crises, has driven increasing interest in this sector. Over the years, advancements in material science have led to improved efficiency and scalability of thermoelectric chargers, solidifying their role in the ongoing transition towards renewable energy sources.
"Thermoelectric devices offer a pathway to harness wasted heat, turning it into usable energy, thus supporting a circular economy in energy usage."
"Thermoelectric devices offer a pathway to harness wasted heat, turning it into usable energy, thus supporting a circular economy in energy usage."
The combination of historical development and the growing demands for sustainable energy solutions make thermoelectric chargers a significant topic of study and application.
Principles of Thermoelectricity
Thermoelectricity is a field that centers on the conversion of temperature differences directly into electrical energy and vice versa. This principle underpins the functionality of thermoelectric chargers and is fundamental to understanding their operation, benefits, and limitations. In this section, we will break down the three key effects that demonstrate thermoelectric phenomena: the Seebeck effect, the Peltier effect, and the Thomson effect. Each of these effects plays a critical role in the efficiency and application of thermoelectric devices, influencing how energy is harnessed from temperature gradients.
Seebeck Effect
The Seebeck effect is the foundational principle behind thermoelectric generators. When two different conductive materials are joined at two junctions, a temperature difference between these junctions generates a voltage. This voltage generation occurs because charge carriers in the materials tend to diffuse from the hot area to the cold area, creating an electric potential. The greater the temperature differential, the higher the voltage produced. This effect is crucial for applications in energy harvesting and converting waste heat into usable electricity.
Key Points of the Seebeck Effect:
- It is the primary mechanism for converting heat into electrical energy.
- Utilized in various applications, like powering small devices from waste heat.
- The efficiency depends on the materials used and the temperature gradient.
Peltier Effect
In contrast to the Seebeck effect, the Peltier effect involves passing an electric current through two different conductors. This results either in cooling or heating at the junctions, depending on the direction of the current. The Peltier effect is commonly leveraged in thermoelectric cooling devices, where one side of the junction absorbs heat, while the other dissipates it. This effect allows for compact refrigeration applications without moving parts, making it advantageous in portable electronic devices and precise temperature control systems.
Key Points of the Peltier Effect:
- It is used primarily in cooling applications.
- Offers significant design flexibility in thermal management.
- Effectiveness is influenced by the material’s thermoelectric properties.
Thomson Effect
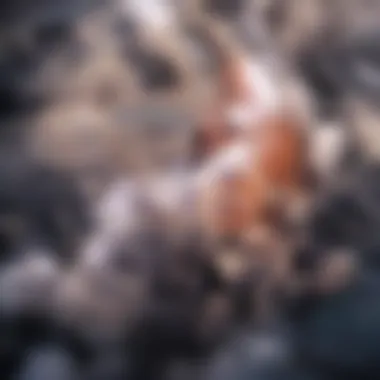
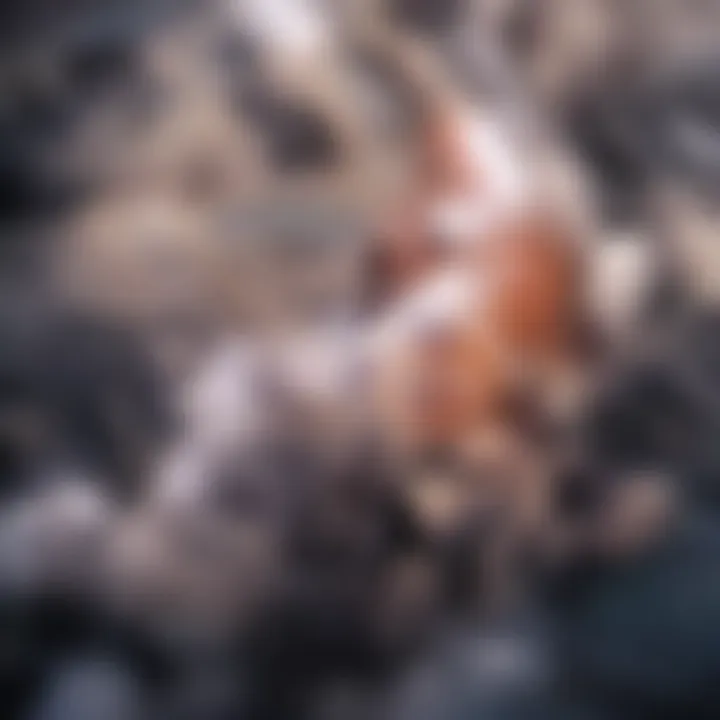
The Thomson effect is another critical phenomenon that describes how a current flowing through a conductor can either absorb or release heat depending on the direction of the current flow and the temperature gradient within the material. This effect is often less emphasized compared to the Seebeck and Peltier effects, but it is significant in understanding the thermal behavior of thermoelectric materials under operational conditions. The implications for thermoelectric applications include improving efficiency and predicting thermal losses in devices.
Key Points of the Thomson Effect:
- It involves heating or cooling along a conductor due to electrical current in the presence of a temperature gradient.
- Helps in refining the design of thermoelectric devices for optimal performance.
- Useful in applications where precise temperature control is necessary.
In summary, the principles of thermoelectricity not only define the operational framework of thermoelectric chargers but also highlight the potential for innovative applications in energy conversion and management. By understanding these principles, researchers and engineers can better harness the power of thermoelectric technologies for sustainable energy solutions.
In summary, the principles of thermoelectricity not only define the operational framework of thermoelectric chargers but also highlight the potential for innovative applications in energy conversion and management. By understanding these principles, researchers and engineers can better harness the power of thermoelectric technologies for sustainable energy solutions.
Core Materials Used in Thermoelectric Chargers
The materials used in thermoelectric chargers play a critical role in determining their efficiency and performance. Understanding the properties and behaviors of these materials is essential in both design and application. The effectiveness of a thermoelectric charger depends largely on its core materials, which directly influence the device's ability to convert heat into electrical energy.
Thermoelectric Materials Overview
Thermoelectric materials possess unique properties that enable them to convert temperature differences into electrical energy through the Seebeck effect. These materials are characterized by their thermoelectric figure of merit, denoted as ZT. A higher ZT value indicates better efficiency. Several factors contribute to this figure of merit, including electrical conductivity, thermal conductivity, and Seebeck coefficient.
- Electrical Conductivity: Materials with high electrical conductivity allow better flow of electric current.
- Thermal Conductivity: Low thermal conductivity maintains a temperature gradient, which is necessary for thermoelectric operation.
- Seebeck Coefficient: A high Seebeck coefficient means that a material can produce a significant voltage per unit temperature difference.
Each material shows specific properties that can make them suitable for particular applications in thermoelectric devices.
Bismuth Telluride
Bismuth telluride is one of the most studied thermoelectric materials, especially for room temperature applications. It has a high Seebeck coefficient and a suitable balance of electrical and thermal conductivity that yields a favorable ZT value. In practical use, it is often found in portable chargers and cooling devices.
This material is typically produced in a compound form, which enhances its thermoelectric performance. Bismuth telluride can operate efficiently in temperatures ranging from -10°C to 150°C, which makes it versatile for various commercial applications. Additionally, it's relatively affordable compared to other thermoelectric materials.
Lead Telluride
Lead telluride is another important thermoelectric material. It shines in high-temperature applications, making it suitable for power generation in industrial processes. Its figure of merit can be significantly improved by alloying, leading to better performance at elevated temperatures.
The material exhibits good electrical conductivity but presents a challenge with thermal conductivity. This is often mitigated by introducing nanostructuring, which helps reduce heat transfer while maintaining effective charge transport. Lead telluride devices can perform well in conditions up to 600°C, expanding their range of applications.
Silicon-Germanium Alloys
Silicon-germanium alloys are distinguished for their performance in high-temperature environments. They have been utilized primarily in automotive and aerospace applications, where durability and efficiency are critical. The mechanical properties of these alloys are also noteworthy, as they withstand various stresses without significant degradation.
In terms of thermoelectric efficiency, silicon-germanium alloys lag compared to bismuth telluride but excel in stability and reliability over extended periods. This makes them ideal for long-term applications in demanding conditions.
In summary, the choice of materials fundamentally shapes the capabilities of thermoelectric chargers. Each material comes with its specific advantages and applications, and ongoing research is constantly enhancing their efficiencies. The future outlook for these materials indicates a trend towards innovative alloying techniques and nanostructures that promise to improve performance metrics.
Design and Configuration of Thermoelectric Chargers
The design and configuration of thermoelectric chargers are critical elements that influence their performance, efficiency, and applicability in various scenarios. As these devices rely on temperature differentials to generate electricity, precise design choices can optimize their output. This section will cover key design considerations as well as effective thermal management techniques that enhance the functionality of thermoelectric chargers.
Design Considerations
When it comes to designing thermoelectric chargers, several factors should be taken into account:
- Material Selection: The choice of thermoelectric materials significantly affects efficiency. Bismuth telluride is commonly used for low-temperature applications. Lead telluride, on the other hand, performs better at higher temperatures. Choosing the right material can optimize energy conversion.
- Module Configuration: The arrangement of thermoelectric modules impacts overall performance. Series or parallel configurations can be evaluated based on voltage and current needs. Engineers must assess requirements carefully to determine the best setup.
- Heat Exchange Mechanism: Efficient heat exchange is essential. The design should facilitate heat absorption on one side and heat dissipation on the other. Fin designs or heat sinks can be employed to maximize surface area, improving heat transfer rates.
Additionally, the mounting arrangement is vital. Factors such as exposure to heat sources and ambient conditions should guide the placement of thermoelectric chargers.
Thermal Management Techniques
Thermal management is key to enhancing the operational effectiveness of thermoelectric chargers. A few effective techniques include:
- Active Cooling Systems: Incorporating active cooling methods, like fans or liquid cooling, can help maintain temperature differentials. Extreme hot or cold environments can significantly influence the performance of thermoelectric devices.
- Insulation Materials: Using insulating materials around the charger assists in retaining heat on the hot side. This improves the efficiency and lifespan of the device.
- Heat Spreading: Designing the charger with materials that can spread heat uniformly can counteract hotspot formation. This spreading maintains a consistent temperature across the thermoelectric materials, promoting optimal performance.
In summary, the design and configuration of thermoelectric chargers are complex and multifactorial. By taking into consideration the materials, configurations, heat exchange strategies, and thermal management techniques, designers can create efficient and robust systems suited for various applications.
Applications of Thermoelectric Chargers
Thermoelectric chargers find relevance across diverse fields due to their unique ability to convert thermal energy into electrical energy. This is particularly important as the demand for energy-efficient solutions continues to grow. The applications of these chargers can markedly enhance technology and the environment, paving the way for more sustainable practices. Each application carries distinctive benefits and considerations that contribute to the overall discussion of thermoelectric technology.
Portable Electronics
Portable electronics represent one of the most significant markets for thermoelectric chargers. The demand for charging solutions that do not rely on traditional power sources has increased. Thermoelectric chargers can harness heat generated by devices themselves or ambient temperature differences to generate power. This means that smartphones or tablet devices can stay charged without needing to plug in. For users who are constantly on the move, this leads to greater convenience.
- Energy autonomy: Users can operate devices longer without reliance on external power sources.
- Reduced e-waste: Longer device lifespan decreases the frequency of battery replacements and contributes to environmental sustainability.
In addition, thermoelectric chargers can be integrated into power banks, allowing users to charge their devices using heat whenever available.
Space Missions
In the context of space missions, thermoelectric chargers have critical applications. They provide a reliable energy source, particularly in lifeless areas where conventional power generation is impractical. Spacecraft such as the Mars rover use radioisotope thermoelectric generators to convert heat from radioactive decay into electrical energy.
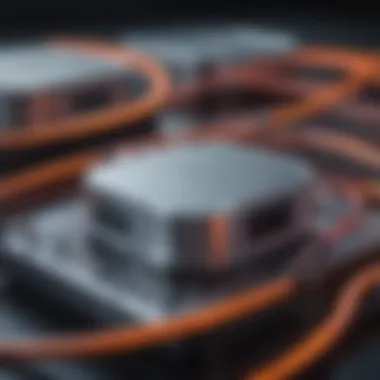
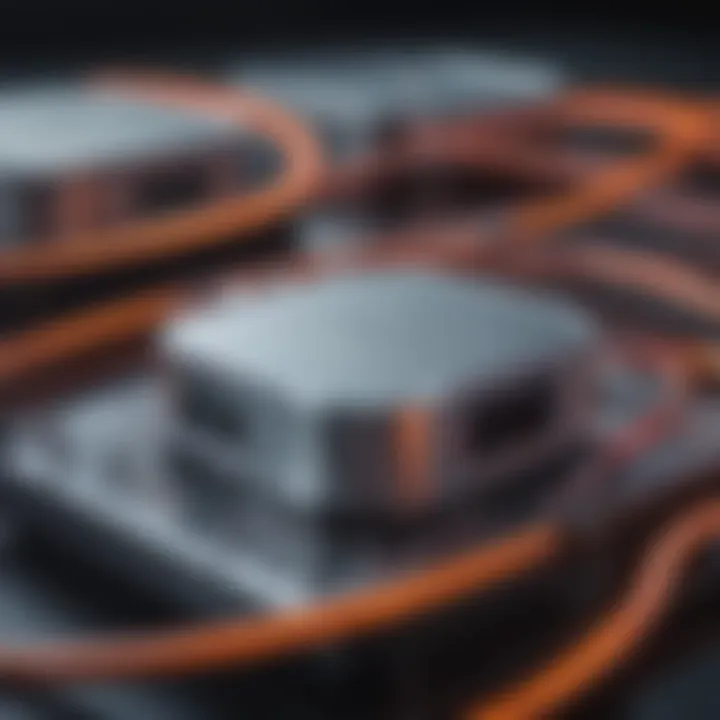
- Reliability in extreme conditions: Unlike solar panels, thermoelectric devices can function regardless of sunlight availability, making them ideal for missions in shadowed areas or on planetary bodies with long nights.
- Long operational life: These systems can operate for years without maintenance, offering consistent power over extended periods.
Implementing thermoelectric chargers in space technology reflects humanity's advancement in harnessing energy in hostile environments, thereby enhancing mission success rates.
Waste Heat Recovery
In industries, waste heat recovery has become a vital area of focus. Many industrial processes generate significant amounts of waste heat, which is typically lost to the environment. Thermoelectric chargers can convert this wasted thermal energy into usable electrical energy, improving overall energy efficiency. This is particularly relevant in sectors such as manufacturing and power generation.
- Energy conservation: Utilizing waste heat reduces the need for additional power generation, lowering operational costs.
- Environmental impact: By capturing and reusing waste heat, industries can minimize their carbon footprint and contribute to greener practices.
The effective integration of thermoelectric technology into waste heat recovery systems highlights its potential for driving industrial energy transformations.
Automotive Systems
Automotive systems are also benefiting from the unique attributes of thermoelectric chargers. As fuel efficiency and vehicle sustainability gain importance, integrating thermoelectric devices in cars can help convert excess heat from engines into electrical energy. This additional power can be used to reduce the load on the vehicle battery, ensuring better overall performance.
- Improved fuel efficiency: By converting waste heat into usable power, vehicles can consume less fuel, promoting fuel economy.
- Integration with other technologies: Thermoelectric systems can work alongside hybrid and electric systems, enhancing the overall efficiency of future vehicular designs.
As automakers increasingly focus on sustainable technologies, thermoelectric chargers will play a pivotal role in the evolution of automotive energy systems.
"The use of thermoelectric technology in various applications makes strides toward a more sustainable future while optimizing energy efficiency in our daily lives and industries."
"The use of thermoelectric technology in various applications makes strides toward a more sustainable future while optimizing energy efficiency in our daily lives and industries."
Exploring the applications of thermoelectric chargers demonstrates their value across sectors, contributing to advancements in technology, energy conservation, and environmental sustainability.
Advantages of Thermoelectric Chargers
Thermoelectric chargers offer several advantages that set them apart from other energy conversion technologies. Understanding these benefits can highlight the significance of thermoelectric systems in modern energy applications.
Energy Efficiency
One of the primary advantages of thermoelectric chargers is their energy efficiency. They can convert heat differentials into electrical energy with relatively high efficiency, especially when operated in environments where temperature gradients exist. For instance, systems designed to harvest waste heat from industrial processes can achieve efficiencies that are comparable to more traditional forms of power generation. This characteristic is particularly valuable in the context of energy sustainability because it allows for the utilization of heat that would otherwise be lost.
Moreover, thermoelectric chargers are capable of producing power without the need for moving parts, thus reducing energy losses commonly associated with mechanical systems. This electromechanical simplicity not only enhances efficiency but also reduces the total energy footprint of the energy conversion process.
Compact and Lightweight
Another noteworthy advantage of thermoelectric chargers is their compact and lightweight construction. Unlike traditional generators that require substantial space and heavy components such as turbines and engines, thermoelectric devices can be designed to be smaller and lighter. This makes them particularly favorable for applications in portable electronics and remote installations where space and weight are constraints.
The small size of thermoelectric chargers enables easier integration into existing systems without the need for extensive modifications. For example, they can be embedded in portable gadgets or used in vehicles to harness waste heat. As a result, their compactness not only leads to enhanced versatility across applications but also minimizes installation complexities.
Low Maintenance Requirements
Thermoelectric chargers have the advantage of low maintenance requirements. Due to their solid-state nature and lack of moving parts, these systems experience less wear and tear compared to traditional energy conversion technologies. This reduced physical degradation results in longer operational lifespans and lower overall maintenance costs. Users can benefit from lower downtime and improved reliability, especially in critical applications where consistent energy supply is essential.
Additionally, the simplicity of thermoelectric systems means that they often require fewer resources for operation. Regular maintenance tasks are typically minimal, which allows for more efficient resource allocation and operational focus. Thus, their durability and minimal upkeep make thermoelectric chargers an attractive option for industries and consumers alike.
"Thermoelectric chargers exemplify a blend of efficiency, compactness, and maintenance simplicity that could redefine energy harvesting methodologies across various sectors."
"Thermoelectric chargers exemplify a blend of efficiency, compactness, and maintenance simplicity that could redefine energy harvesting methodologies across various sectors."
Limitations and Challenges
Understanding the limitations and challenges of thermoelectric chargers is crucial for anyone interested in their practical applications and potential. These issues can affect performance and adoption in various sectors. Addressing these challenges can lead to more efficient designs and broader acceptance of this technology.
Material Limitations
Thermoelectric chargers rely heavily on the materials used to convert heat into electricity. While certain materials like bismuth telluride are effective, they often have narrow operating temperature ranges. This limits their usability in extreme conditions. Additionally, the search for cheaper and more abundant materials poses a significant challenge. For example, lead telluride has excellent thermoelectric properties but it is expensive and less abundant. The field is actively researching new materials to overcome these limitations, but progress can be slow and complicated.
Cost Implications
The overall cost of thermoelectric chargers is a significant barrier to their widespread adoption. Although they can provide energy in remote locations, the initial investment can be high. This is particularly true for high-performance materials that are necessary for efficiency. The manufacturing processes can also be complex and costly. Therefore, to improve the attractiveness of thermoelectric devices, researchers are focusing on cost-effective methods of production and ways to utilize less expensive materials.
Efficiency Relative to Other Technologies
In comparison to other energy conversion technologies, thermoelectric chargers often lag in efficiency. Traditional generators, such as combustion engines, generally convert thermal energy to mechanical energy with higher efficiency. Also, newer technologies like solar panels have made significant advances in both efficiency and cost-effectiveness. Therefore, thermoelectric chargers must evolve to compete. Developer need to work on improving their conversion ratios and addressing efficiency losses at different temperature ranges.
"While thermoelectric chargers have great potential, understanding their limitations helps inform the design and application in real-world scenarios."
"While thermoelectric chargers have great potential, understanding their limitations helps inform the design and application in real-world scenarios."
Addressing these limitations and challenges is essential not only for enhancing existing technology but also for paving the way for future innovations in this energy sector.
Recent Advancements in Thermoelectric Charging Technology
The field of thermoelectric charging technology has seen significant growth and innovation in recent years. Researchers and developers are exploring new materials and design approaches to improve efficiency, reduce costs, and expand applications. The importance of these advancements lies in their potential to enhance sustainable energy solutions and meet the increasing demand for portable energy.
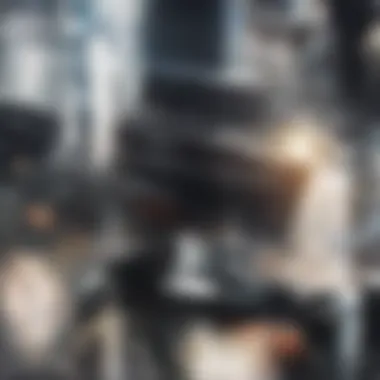
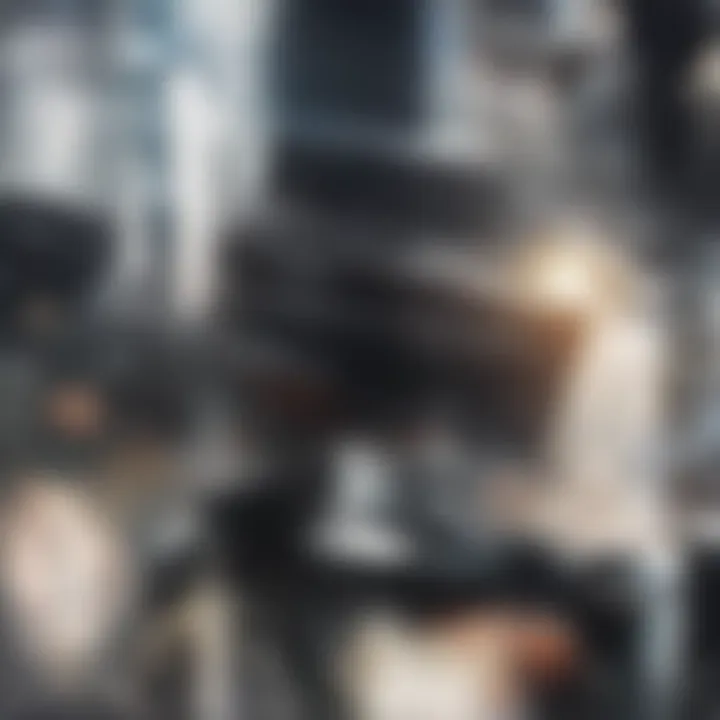
Emerging Materials
Material science plays a crucial role in the performance of thermoelectric chargers. Traditional materials like bismuth telluride have served their purpose well, but the search for alternatives continues. Emerging materials are being examined for their thermoelectric properties, which can lead to better efficiency and lower production costs.
One notable class of materials is skutterudites. These materials have a complex crystal structure that can trap low-energy vibrations, which enhances their thermoelectric performance. Additionally, perovskite oxides are gaining attention due to their high thermoelectric figure of merit at elevated temperatures, making them suitable for various applications. Furthermore, organic thermoelectric materials offer the potential for flexible, lightweight chargers, which could revolutionize portable electronics.
The exploration of these new materials could dramatically change the landscape of thermoelectric charging technology. Not only may they offer improved performance, but they also tend to be more environmentally friendly and potentially cheaper to produce.
Innovative Design Approaches
As thermoelectric charging technology develops, innovative design approaches are emerging simultaneously. These approachs focus on optimizing the devices' functionality to increase energy conversion efficiency. For one, modular designs are being favored. Such configurations allow for easier scaling and integration into various systems. This flexibility makes it possible for manufacturers to customize units according to specific application needs.
Furthermore, researchers are implementing advanced thermal management techniques in device designs. These techniques help in maintaining optimal temperature gradients, essential for enhancing the performance of thermoelectric chargers. The use of heat exchangers and advanced insulation methods can significantly improve the efficiency of thermoelectric systems.
"The ongoing evolution in materials and designs could pave way for a paradigm shift in how we harness and utilize thermoelectric energy."
"The ongoing evolution in materials and designs could pave way for a paradigm shift in how we harness and utilize thermoelectric energy."
Next, further exploration into future directions and the implications of these advancements can be considered.
Future Directions and Potential Developments
Thermoelectric technology is evolving, and its future holds significant promise. Understanding the potential developments is crucial for various stakeholders involved, including researchers, manufacturers, and consumers. The continual search for improved thermoelectric materials and devices is a key driver in enhancing the performance of thermoelectric chargers. This section emphasizes significant areas of focus that will shape the trajectory of thermoelectric technology.
Research Initiatives
Research initiatives play a vital role in the advancement of thermoelectric chargers. Scientists and engineers are currently exploring new materials that offer better efficiency and cost-effectiveness. Research at institutions like MIT and Stanford University has focused on nanostructured materials, which can significantly enhance thermoelectric performance by optimizing electrical and thermal properties. Moreover, initiatives aimed at understanding the underlying physics of thermoelectric effects are critical in developing more effective devices. Some notable research endeavors include:
- Nanomaterials: Researchers are investigating the use of nanostructured bismuth telluride and lead telluride. The modifications at the nanoscale can improve the Seebeck coefficient, which relates to the voltage generated due to temperature differences.
- Hybrid Materials: The combination of organic and inorganic materials is being explored to enhance flexibility and reduce manufacturing costs. Such innovations can lead to more adaptable applications in flexible electronics.
- Machine Learning: The application of artificial intelligence in material synthesis and property prediction can accelerate the discovery of high-performing thermoelectric materials. This approach may shorten the timeline for bringing new materials into commercial applications.
Commercialization Prospects
Commercialization prospects for thermoelectric chargers are growing, driven by increasing demand for sustainable energy solutions. Several industries are keen on integrating thermoelectric technology due to its potential for converting waste heat into usable energy. The automotive sector, for instance, is exploring mechanisms to harvest waste heat from engines, thereby improving fuel efficiency. Additionally, portable electronics manufacturers are looking at thermoelectric chargers as a way to enhance user convenience and reduce reliance on traditional power sources.
Challenges remain in scaling up production and reducing costs. However, as research initiatives yield results, it is likely that:
- Market Growth: The market for thermoelectric devices is expected to expand, particularly in applications related to renewable energy and resource management. This includes scenarios like urban environments where heat is often wasted.
- Investment: Increased funding from government and private sectors towards sustainable energy initiatives can bolster research. This may lead to more collaborations between startups and established manufacturers to speed up commercialization.
- Diverse Applications: The versatility of thermoelectric chargers can cater to multiple sectors, from consumer technology to aerospace, promoting a wider acceptance of this technology.
"The shift towards sustainable technologies is more critical than ever. Future developments in thermoelectric charging can play a pivotal role in this transition.”
"The shift towards sustainable technologies is more critical than ever. Future developments in thermoelectric charging can play a pivotal role in this transition.”
Environmental Considerations
The role of environmental considerations in thermoelectric chargers is critical for both their acceptance and effectiveness in a rapidly advancing energy landscape. As the world increasingly directs its focus toward sustainable energy solutions, understanding how these chargers impact the environment becomes significant. This analysis covers two main aspects: the sustainability of materials utilized in these devices and the lifecycle assessment of their overall environmental footprint.
Sustainability of Materials
Materials used in thermoelectric chargers must exhibit a balance of efficient energy conversion and minimal environmental impact. Bismuth telluride, a prominent thermoelectric material, presents both advantages and challenges. On one hand, it provides excellent performance in converting heat to electricity. On the other hand, the extraction and processing of its components can be resource-intensive and potentially harmful to local ecosystems.
Moreover, alternatives like silicon-germanium alloys and lead telluride are being investigated for their sustainability. They hold potential for reducing the ecological footprint while maintaining performance effectiveness. Thus, ongoing research aims to discover new materials that strike this balance better than existing options.
Lifecycle Assessment
Lifecycle assessments (LCA) offer a systematic approach to evaluate the environmental impacts associated with thermoelectric chargers throughout their entire lifespan, from raw material extraction to disposal. This analysis provides insights into the overall energy usage and emissions generated at each stage.
• Raw Material Extraction: Assessing the mining of raw materials required for production, focusing on energy consumed and emissions produced.
• Manufacturing Process: Evaluating the energy efficiency of processes employed in creating the thermoelectric devices.
• Usage Phase: Calculating how much energy the charger generates in relation to the energy consumed in its production.
• End-of-Life Disposal: Considering the waste produced from the chargers and the potential for recycling and reuse of materials.
Tackling these factors through LCAs enables stakeholders to improve existing processes and materials and encourages the development of new, sustainable methodologies to minimize adverse environmental impacts. By emphasizing environmental considerations, the thermoelectric charger industry can enhance its role in promoting sustainable energy solutions.
"Understanding how materials can be sustainably sourced and how their entire life from creation to disposal can be improved is essential for a cleaner future."
"Understanding how materials can be sustainably sourced and how their entire life from creation to disposal can be improved is essential for a cleaner future."
Through rigorous environmental assessments, the technological advancements in thermoelectric chargers can align more closely with the global movement towards greener energy practices.
End
The conclusion of this article serves a critical role in summarizing the insights gained about thermoelectric chargers. It ties together the various elements discussed, emphasizing the significance of understanding how these devices work and their impact on future energy systems. Throughout the article, we have explored the fundamental principles underlying thermoelectric technology, the advantages it offers, the challenges that persist, and the promising directions for future advancements.
Summary of Key Points
In summation, thermoelectric chargers convert temperature differences into usable electrical energy. This specialization enables them to harness heat waste in a variety of applications, from portable electronics to automotive systems. The materials used, such as bismuth telluride and silicon-germanium alloys, play a key role in the efficiency and effectiveness of these systems. While the energy efficiency and lightweight design are significant advantages, the article also highlights notable drawbacks, including material limitations and cost implications. Recent technological advancements hold promise for improving the performance of these chargers, thus expanding their potential application across different industries.
Implications for Future Energy Systems
As we consider future energy systems, thermoelectric chargers present exciting opportunities. They offer a pathway to sustainable energy solutions by allowing for the conversion of waste heat into usable power. This can potentially reduce dependency on traditional energy sources and mitigate environmental impact. The ongoing research initiatives and innovations in material science signify hope that these technologies will not only become more efficient but also more affordable in the coming years. Therefore, the integration of thermoelectric charging solutions could play a pivotal role in advancing renewable energy practices, and shaping a more sustainable energy landscape.