Topo Cloning Protocol: Techniques and Applications
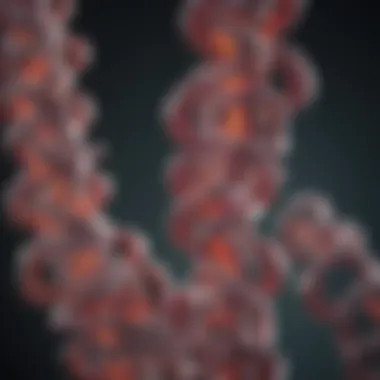
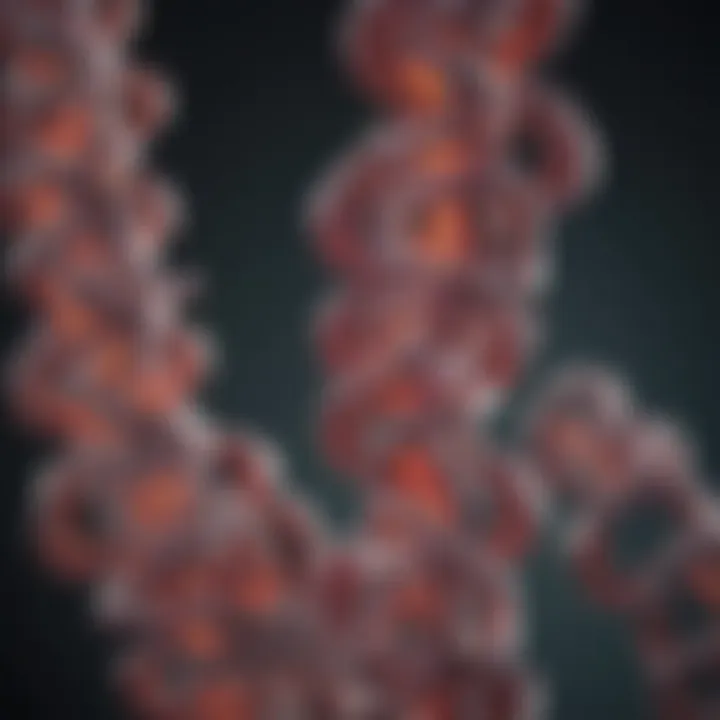
Article Overview
Purpose of the Article
This article aims to provide a comprehensive exploration of topo cloning protocol, emphasizing not only the methodology but also its diverse applications in the fields of genetics and synthetic biology. Understanding this protocol can help researchers and students facilitate their work more effectively, considering its significance in modern molecular biology.
Relevance to Multiple Disciplines
Topo cloning has considerable relevance across various scientific disciplines. In genetics, it aids in gene cloning and expression studies, while in synthetic biology, it is pivotal for constructing complex biological systems. The protocols enhance the speed and efficiency in these areas, making them invaluable for both academic and industrial applications.
Research Background
Historical Context
The development of the topo cloning technique marks a notable advancement in molecular biology. It arises from the discovery of topoisomerases, enzymes that regulate the overwinding or underwinding of DNA. The introduction of topoisomerase-mediated cloning in the late 1990s provided researchers with a faster alternative to traditional methods like restriction cloning. Over the years, its adoption has transformed laboratory practices significantly.
Key Concepts and Definitions
To fully understand topo cloning, several key concepts and definitions are essential:
- Topoisomerase: Enzymes that manage DNA topology by inducing breaks, allowing the DNA strands to unwind and rejoin.
- Vector: A DNA molecule used to transport foreign genetic material into another cell.
- Ligase: An enzyme that facilitates the joining of DNA strands by catalyzing the formation of phosphodiester bonds.
As students, educators, and professionals engage with the topo cloning protocol, they must grasp these fundamental ideas to appreciate the procedure's intricacies.
Intro to Topo Cloning Protocol
The study of topo cloning protocols is fundamental to modern molecular biology. This section helps to frame the narrative of how this technique has evolved and its relevance across various fields. The introduction provides a scaffold for understanding the detailed analyses that will be presented throughout the article. By dissecting the foundational aspects of topo cloning, researchers and practitioners can better comprehend its applications, signaling a deeper awareness of genetic manipulation techniques available today.
Definition and Overview
Topo cloning refers to a method that utilizes topoisomerases to facilitate the insertion of DNA fragments into vectors. Unlike traditional cloning approaches, this technique allows for a more streamlined insertion process with minimal preparation. Topoisomerases are enzymes that manage the topology of DNA. They manipulate the winding and unwinding of the DNA helix, creating a conducive environment for the effective insertion of the desired DNA fragments into plasmids or other vectors. This results in a faster and often more precise cloning method.
The primary focus of topo cloning is on the efficiency and ease of use. This method's ability to handle multiple insertions without restrictions due to incompatible ends is a significant advantage over other traditional methods. Researchers can achieve high cloning efficiency even with minimal manipulation.
Historical Context
The foundational concepts behind topo cloning did not emerge overnight. The science of DNA manipulation began gaining momentum in the mid-20th century. In particular, the discovery of topoisomerases was pivotal. Researchers like James Watson and Francis Crick laid early groundwork regarding the DNA double helix. However, the role of topoisomerases became more pronounced later, as molecular biologists sought methods to streamline cloning protocols.
By the 1990s, significant progress was made in the understanding of DNA topology. Techniques evolved, leading to the development of specialized vectors that utilized topoisomerase activity. The introduction of TOPO® cloning by Invitrogen marked a critical point in molecular biology, making the technique highly accessible for labs around the world. This evolution highlights how historical developments in genetic research have contributed to the current methodologies used in laboratories today.
Mechanism of Action
Understanding the mechanism of action of topo cloning is essential for appreciating its applications and efficacy in genetic engineering. The success of topo cloning hinges on the functionality of topoisomerases, which are crucial enzymes that manage DNA topology during replication, transcription, and repair. Specifically, these enzymes facilitate the cutting, unwinding, and rejoining of DNA strands. This process is vital, as it mitigates the torsional strain that accumulates in DNA when it is unwound. Thus, the role of topoisomerases extends beyond mere structural work; they are integral in ensuring the fidelity of genetic information transfer.
The benefits of utilizing topoisomerases in cloning are manifold. First, the ability to insert DNA fragments into vectors with high precision and without the need for restriction enzymes significantly streamlines the cloning process. Furthermore, topo cloning typically occurs in a single step, where the topoisomerase enzyme facilitates the ligation of the insert to the vector directly. This eliminates some of the common pitfalls associated with traditional cloning methods, such as the requirement for specific overhangs or the potential for incomplete ligation reactions.
However, there are considerations to take into account when relying on these enzymes. The efficiency of the topoisomerase reaction can be influenced by several factors, including the size and complexity of the DNA fragments being ligated. It's important to ensure that the fragments are prepared properly for optimal results. Furthermore, an understanding of the DNA structure and its functional implications is necessary when developing effective cloning strategies.
Role of Topoisomerases
Topoisomerases are categorized into two main classes: Type I and Type II. Type I topoisomerases cut one strand of a DNA molecule, allowing the uncut strand to pass through before resealing the break. In contrast, Type II topoisomerases cut both strands of the DNA, facilitating more complex maneuvers such as supercoiling.
In topo cloning, Type I topoisomerases are extensively used. These enzymes make a transient break in one strand of DNA, thereby allowing it to entwine with the target strand. This creates a covalent bond between the DNA and the enzyme through a phosphotyrosyl linkage. Once the strand is cleaved and the DNA is inserted, the enzyme can reattach the strands, effectively integrating the new fragment into the desired location.
One interesting aspect of topoisomerases is their ability to relax supercoiled DNA. This action is important during the cloning process because supercoiling can hinder both the accessibility of DNA sequences and enzyme interactions. Therefore, topoisomerases not only facilitate cloning but also help maintain the structural integrity and functional accessibility of the DNA.
DNA Structure and Function Considerations
DNA's structure is paramount when discussing the mechanism of action in topo cloning. Each strand of DNA is composed of a sugar-phosphate backbone and nitrogenous bases that determine the genetic code. The antiparallel arrangement of the strands contributes to the overall stability and higher-order structures such as supercoils and loops. For this reason, understanding the nuances of DNA topography is essential when preparing fragments for cloning.
There are several key considerations regarding DNA structure in the context of topo cloning. The presence of secondary structures can influence how effectively an insert can ligate into a vector. Complex folds or hairpins may obstruct the enzyme's ability to cut and rejoin DNA. Thus, ensuring a linear, accessible form of the DNA fragment is critical for success.
Furthermore, the orientation and compatibility of the ends of the DNA fragment with the vector must be taken into account. Improper alignment could lead to unsuccessful ligation, thus reducing cloning efficiency. It's wise to assess these structural elements before initiating the cloning process. Understanding these considerations enhances the ability to design a robust cloning strategy.
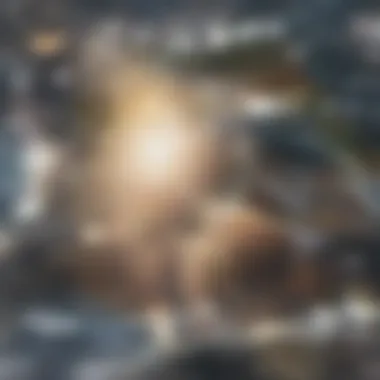
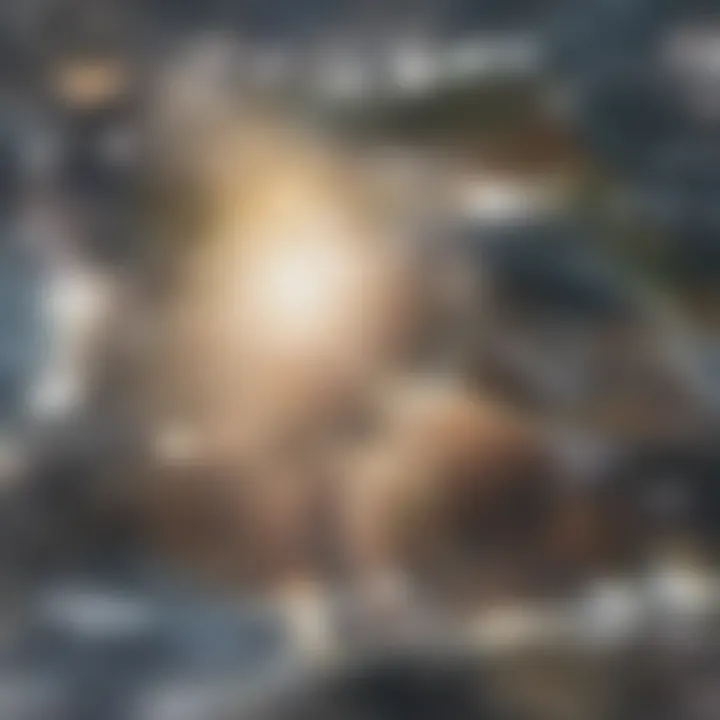
"The efficiency of topo cloning is heavily influenced by the structural elements of DNA utilized in the process. Careful design and consideration yield better outcomes."
"The efficiency of topo cloning is heavily influenced by the structural elements of DNA utilized in the process. Careful design and consideration yield better outcomes."
Essential Components of Topo Cloning
The process of topo cloning relies on specific elements that ensure its effectiveness and precision. Understanding these core components is vital for any researcher or student seeking to utilize this technique in their work. This section delves into two fundamental aspects: vector selection and insert design and preparation. Each subcomponent plays a crucial role in the overall success of the cloning protocol.
Vector Selection
Selecting an appropriate vector is crucial for the topo cloning process. Vectors serve as carriers for the DNA fragment intended for cloning. They come in various forms, including plasmids, cosmids, and BACs. The choice of vector profoundly influences the ease of the subsequent steps in the cloning process, such as transformation and expression. Specific factors to consider in vector selection include:
- Compatibility with Host System: It is important that the vector is compatible with the host cell type, often E. coli species. Each vector has its unique origin of replication (ori), which can affect its ability to replicate within the host.
- Selection Markers: Vectors typically contain antibiotic resistance genes, allowing only those cells that have successfully taken up the vector to grow in selective media. This drastically simplifies the screening process of colonies.
- Multiple Cloning Sites (MCS): It is essential that the vector has an MCS to facilitate the easy insertion of the DNA fragment. The presence of various restriction sites allows for a flexibility when incorporating the insert.
Selecting the right vector contributes significantly to the overall efficiency and success of the cloning process. When vectors are chosen judiciously, downstream applications such as protein expression or functional assays can proceed with fewer complications.
Insert Design and Preparation
The design and preparation of the DNA insert is equally important. The insert refers to the DNA fragment that is to be cloned into the vector. Its design directly impacts how well the fragment integrates with the vector. Various considerations should be made during this phase:
- Length of the Insert: Optimal insert lengths usually range between 100 bp to 3 kb, depending on the vector's capabilities. Longer inserts may present challenges during cloning and transformation.
- End Compatibility: It is necessary to ensure that the ends of the insert are compatible with the ends of the vector. Specific design can be made to create overhangs that facilitate the ligation process.
- Purification: After amplification through methods like PCR, the insert must be purified to remove enzymes, nucleotides, and other impurities. This ensures a cleaner ligation process.
Ultimately, the insert design and preparation phase sets the stage for successful cloning. Taking the time to optimize this aspect can lead to better cloning efficiency and higher yields.
"Without proper vector selection and insert preparation, even the best protocols can produce unsatisfactory results."
"Without proper vector selection and insert preparation, even the best protocols can produce unsatisfactory results."
By focusing on these essential components, researchers can enhance the robustness of their topo cloning protocols, leading to more efficient and reliable outcomes.
Step-by-Step Protocol
The step-by-step protocol is fundamental in the process of topo cloning. It serves as a structured guide that outlines each critical phase involved in achieving successful cloning results. Breaking down the process allows researchers to identify potential pitfalls and implement best practices to optimize outcomes. Understanding the protocol is essential for ensuring reproducibility and efficacy in experiments. This section will delve into the distinct stages of the protocol, focusing on preparation, ligation, and transformation.
Preparation of DNA Fragments
The initial step involves the meticulous preparation of DNA fragments. This stage is crucial, as the quality and specificity of these fragments directly impact the overall success of the cloning process. Typically, DNA fragments are generated through PCR or restriction enzyme digestion. It is essential to optimize the conditions for these methods to enhance yield and accuracy.
The following elements should be considered during preparation:
- Template Quality: The source DNA must be pure and intact. Contaminants can inhibit the efficiency of the cloning process.
- Primer Design: When using PCR, primers should be designed to flank the region of interest, ensuring that the amplified product matches the desired sequence.
- Length of Inserts: Generally, a fragment length between 100 bp and 4 kb is ideal for topo cloning. Longer fragments might cause complications in ligation.
After the fragments are generated, they should be purified to eliminate residual enzymes and nucleotides. A purification step enhances the reliability of subsequent stages, minimizing undesirable outcomes.
Ligation Process Explained
Once the DNA fragments are ready, the next step is the ligation process. This is where the topoisomerase enzyme facilitates the joining of the insert into the vector. The efficiency of this step is paramount; low ligation efficiency can lead to reduced cloning success.
During the ligation, specific considerations come into play:
- Enzyme Choice: T4 DNA Ligase is commonly used, but it's essential to choose a ligase appropriate for the ends of the insert and vector.
- Reaction Conditions: The buffer composition, temperature, and duration affect enzyme activity and, consequently, ligation success. A common recommendation is to perform the reaction at room temperature or 16°C for 30 minutes to a few hours, depending on the enzyme used.
- Vector and Insert Molar Ratios: Optimal ratios can significantly enhance ligation rates. A typical starting point might be a 3:1 or 5:1 ratio of insert to vector.
"The ligation process is the heart of cloning; improper execution can lead to failed experiments."
"The ligation process is the heart of cloning; improper execution can lead to failed experiments."
Transformation into Host Cells
The final stage of the topo cloning protocol is the transformation of the ligation mixture into host cells, often Escherichia coli. This process allows the introduction of the recombinant DNA into a living organism, facilitating subsequent analysis or expression studies.
Key considerations during transformation are:
- Competent Cell Preparation: Competent cells must be prepared to maximize uptake efficiency. Heat-shock or electroporation methods can be used, depending on the specific protocol.
- Recovery Phase: After transformation, a recovery period in a non-selective medium is critical. This allows the cells to express the necessary plasmid genes before they are subjected to antibiotic selection.
- Selection: Following recovery, cells are plated onto selective media that contain an antibiotic corresponding to the resistance gene in the plasmid. Monitoring colony formation yields insights into cloning success.
In summary, the step-by-step protocol for topo cloning integrates meticulous preparation, precise ligation, and effective transformation processes. Each phase is interlinked, contributing to the overall effectiveness and reproducibility of the technique in various applications.
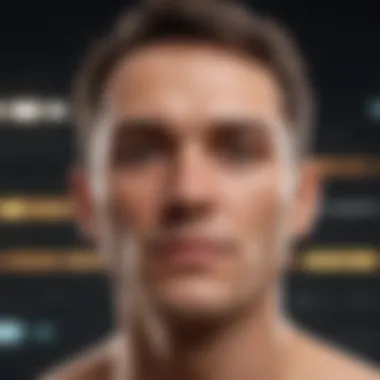
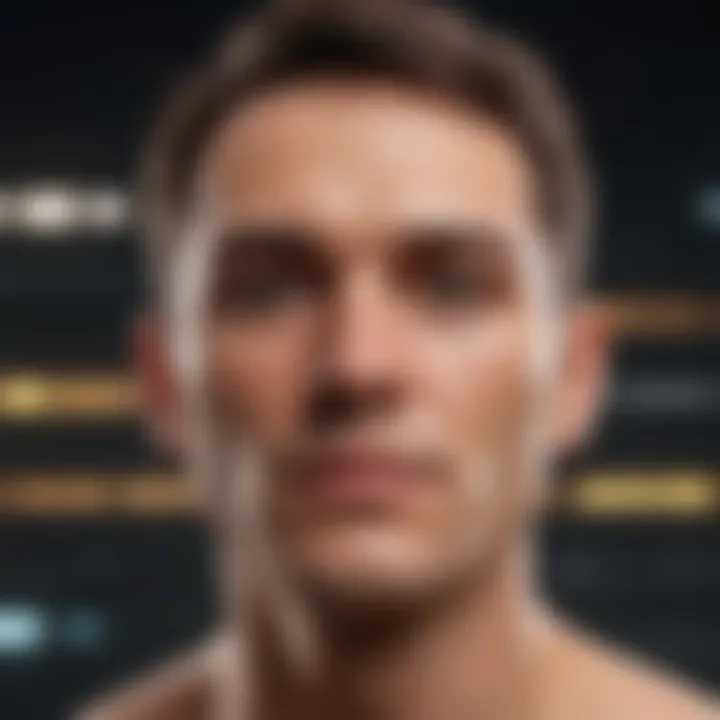
Applications of Topo Cloning
Topo cloning has emerged as a pivotal technique in molecular biology, particularly due to its ability to simplify the cloning process. This method leverages the properties of topoisomerases, which facilitate the insertion of DNA fragments into vectors with high efficiency. The applications of topo cloning span various fields, each underscoring its value in advancing scientific research and biotechnological development.
Gene Cloning and Expression Studies
One primary application of topo cloning is in gene cloning and expression studies. Researchers utilize this technique to amplify specific genes, allowing for extensive analysis of gene function and regulation. The high efficiency of topo cloning reduces the time and resources necessary for generating clones, enabling quicker assessment of gene expression levels in a variety of systems.
When combined with expression vectors, topo cloned genes can be expressed in host cells, leading to the production of proteins for further study. This has significant implications for areas like protein structure analysis, enzyme function characterization, and even vaccine development. The rapid nature of this technique supports high-throughput studies, making it invaluable for laboratories engaged in genetic research.
Synthetic Biology Ventures
In synthetic biology, topo cloning plays a critical role in the design and assembly of new genetic constructs. The flexibility of this method allows scientists to create complex genetic circuits by easily inserting multiple DNA fragments into a single vector. This ease of use promotes innovation in designing engineered organisms with specified functionalities.
Moreover, the combination of topo cloning with sequencing tools facilitates the verification of synthetic constructs. Researchers can quickly confirm the presence and correct orientation of inserted genes, reducing the chances of unexpected outcomes during experimental processes. This has dramatically accelerated the pace at which new synthetic pathways and organisms can be created, pushing the boundaries of what synthetic biology can achieve.
Metagenomics Research Applications
Topo cloning is also pivotal in metagenomics research. This area involves analyzing genetic material from environmental samples, often unculturable organisms. Using topo cloning techniques, scientists can clone DNA fragments from such samples easily, allowing for the characterization of microbial communities without the need for cultivation.
This application is crucial for understanding ecological interactions and tracking genetic diversity within complex ecosystems. For instance, cloning of large DNA fragments facilitates the identification of novel genes with potential biotechnological applications. The ability to explore and manipulate genetic material from diverse sources highlights the adaptability and significance of topo cloning in both environmental and health-related research.
"Topo cloning streamlines the process of gene insertion, offering high efficiency and reliability, which are crucial for advancing research in various fields."
"Topo cloning streamlines the process of gene insertion, offering high efficiency and reliability, which are crucial for advancing research in various fields."
In summary, the applications of topo cloning are vast and varied. From gene cloning to synthetic biology and metagenomics, its role in modern biotechnology cannot be overstated. The benefits it brings to research methodology and experimental design further solidify its position as a fundamental technique in molecular biology.
Advantages of Topo Cloning
The advantages of topo cloning are significant, making it a favored technique among researchers. Understanding these benefits is crucial for anyone engaged in genetic engineering or molecular biology. The following sections highlight particular advantages that set topo cloning apart.
Speed and Efficiency
One of the most compelling advantages of topo cloning is its speed. This technique allows for the rapid insertion of DNA fragments into vectors. Traditional cloning methods often involve lengthy steps like restriction enzyme digestion and ligation. In contrast, topo cloning simplifies this process substantially.
The speed is linked to the action of topoisomerases, which facilitate the direct ligation of the insert into the vector without the need for prior enzyme manipulation. As a result, the time from initial fragment preparation to successful transformation can be reduced to mere hours.
Moreover, efficiency is a critical factor. Topo cloning consistently yields higher rates of successful cloning compared to other methods. This reliability is valuable in experiments where time is of the essence and resources are limited. Researchers spend less time troubleshooting and more time on downstream applications, maximizing their productivity.
"The rapid nature of topo cloning can lead to significant advancements in research timelines and project outcomes."
"The rapid nature of topo cloning can lead to significant advancements in research timelines and project outcomes."
Versatility in Use
The versatility of topo cloning cannot be understated. This method is adaptable to various applications, making it suitable for a wide range of research contexts. From basic gene cloning to the complex tasks involved in synthetic biology, topo cloning accommodates different types of DNA fragments without significant modifications to the protocol.
For instance, researchers can employ topo cloning for constructing expression vectors for protein production or for generating plasmids designed for gene editing. Its flexibility ensures that it remains relevant across different domains of molecular biology, including metagenomics and environmental studies.
Additionally, topo cloning lends itself to high-throughput applications. This characteristic is particularly advantageous in today's biotechnology landscape, where researchers often deal with multiple samples simultaneously. The straightforward nature of the protocol allows for easy scaling.
Limitations and Challenges
The study of limitations and challenges related to topo cloning is essential for a nuanced understanding of the protocol. Awareness of these factors enables researchers and practitioners to make informed decisions about its application in various contexts. While topo cloning has several benefits, it is not without its drawbacks. Here, we will delve into specific issues that may arise during the process, examining their significance and implications.
Specificity Issues
One of the primary limitations of topo cloning lies in its specificity. The method primarily relies on topoisomerases, which have specific binding affinities and capabilities. This means that certain DNA fragments may not bind effectively to the vectors used, leading to incomplete or erroneous cloning. Specificity issues can stem from several factors:
- Sequence Compatibility: The insert DNA must be compatible with the vector, as specific sequences can hinder the efficiency of the cloning process.
- Vector Design: Certain vectors may have inherent limitations that restrict the types of inserts they can accommodate.
- Reaction Conditions: Environmental factors such as temperature, pH, and ionic strength can influence the activity of topoisomerases, impacting specificity.
These issues can result in low cloning rates or even the absence of desired clones altogether, thus affecting downstream applications.
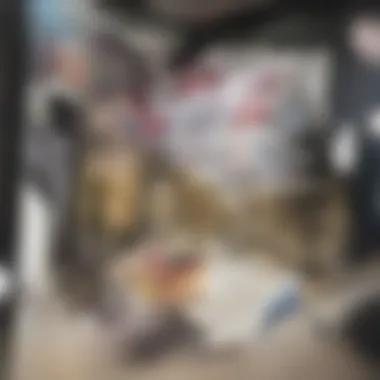
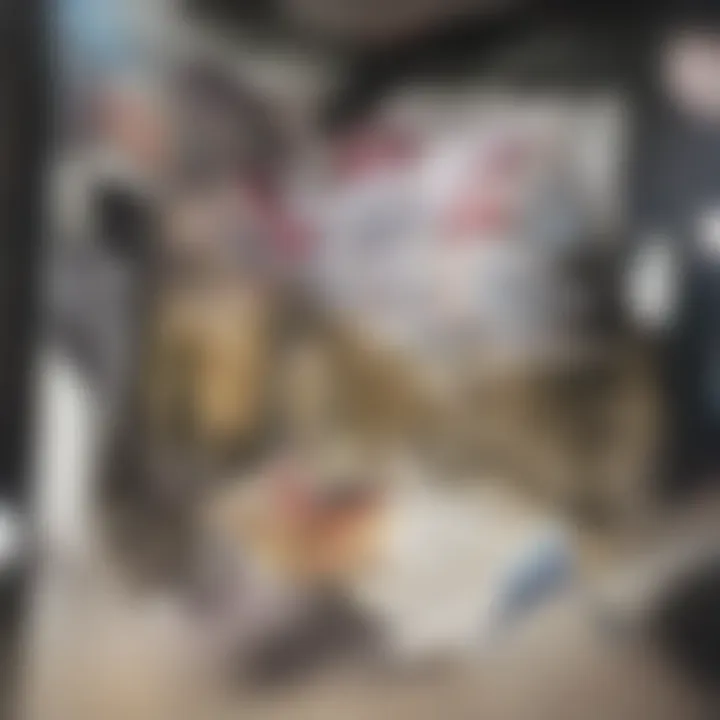
Cost Considerations
Cost is another significant factor that researchers must consider when employing topo cloning. The expenses associated with this technique can be broken down into several categories:
- Reagents and Kits: Commercially available topo cloning kits can be relatively expensive compared to traditional cloning methods. The costs relate to the quality of reagents provided, which often impact performance and reproducibility.
- Equipment Investment: Adequate laboratory facilities and equipment may be necessary, which may require additional financial input.
- Time Investment: While topo cloning is designed to be faster than traditional methods, troubleshooting and refining the process can still consume valuable time.
Consequently, smaller laboratories or those with limited budgets may find these costs prohibitive, limiting access to the advantages provided by topo cloning.
Competitor Techniques
The challenge of evolving technologies means that researchers aren’t limited to topo cloning alone. Several alternative methods exist that can rival or complement this protocol, including:
- Restriction Enzyme Cloning: A more traditional method that involves the use of restriction enzymes to cut DNA at specific sites. While this method allows more control over the process, it is often more time-consuming.
- Gateway Cloning: This technique utilizes specific recombination sequences, which can facilitate the transfer of DNA between different vectors with high efficiency.
- Golden Gate Cloning: A method that allows for the simultaneous assembly of multiple fragments of DNA, providing versatility and facilitating high-throughput applications.
Each of these competitor techniques comes with its own set of advantages and limitations, and the choice of method ultimately depends on the specific goals of the research project.
Understanding the limitations and potential challenges of topo cloning can significantly improve the overall effectiveness of your research endeavors. By balancing these factors against the advantages, researchers can make well-informed choices.
Understanding the limitations and potential challenges of topo cloning can significantly improve the overall effectiveness of your research endeavors. By balancing these factors against the advantages, researchers can make well-informed choices.
Troubleshooting Common Problems
Addressing common issues in topo cloning protocols is essential to ensure the success of experiments. Understanding these challenges allows researchers to develop effective strategies to overcome them, ultimately improving the quality and reliability of their results. This section focuses on two prominent problems that can arise during cloning: low cloning efficiency and contamination issues.
Low Cloning Efficiency
Low cloning efficiency occurs when the desired DNA insert does not efficiently ligate into the vector. This problem can significantly impact overall success rates in experiments. Factors that contribute to low efficiency include the quality of the DNA, the concentration of the insert and vector, and the reaction conditions.
To improve cloning efficiency, consider the following:
- DNA Quality: Ensure that the DNA fragments are of high purity and free from inhibitors. Using a gel extraction kit can help purify DNA from agarose gels.
- Concentration Optimization: Adjust the ratio of the vector to insert. Usually, a molar ratio of 1:3 gives optimal results, but this can vary depending on the fragments used.
- Reaction Conditions: Optimize ligation conditions, such as temperature and time. Running the ligation at a lower temperature for longer periods can sometimes increase efficiency.
Careful attention to these factors can lead to a higher success rate in generating recombinant clones.
Contamination Issues
Contamination is another pressing concern in topo cloning. This can arise from various sources, including reagents, vectors, or even during handling. Contamination can lead to false positives, decreased cloning efficiency, and compromised experimental integrity.
Here are several steps to mitigate contamination risks:
- Use Fresh Reagents: Always use freshly prepared reagents and check for sterility. Avoid reusing opened reagents whenever possible.
- Proper Lab Techniques: Employ standard lab techniques to minimize risk. This includes using sterile pipette tips, working in a sterile environment, and ensuring that all glassware and instruments are decontaminated.
- Regular Monitoring: Routinely culture negative controls alongside experimental samples to detect potential contamination early. This can help identify contamination sources and prevent larger issues in the cloning process.
In summary, tackling these common issues in topo cloning protocols enhances the likelihood of successful experimentation. Understanding the roots of low cloning efficiency and contamination can lead to more robust and reliable results.
Future Perspectives in Topo Cloning
The landscape of molecular biology is constantly evolving, and topo cloning plays a significant role in this transformation. As researchers push the boundaries of genetics and synthetic biology, the future perspectives in topo cloning are crucial to understanding how this technique can adapt and thrive in new environments.
Several specific elements contribute to the importance of future developments in topo cloning. Firstly, the integration of advanced technologies improves the precision, speed, and ease of execution of cloning procedures. Moreover, as knowledge of genetic sequences expands, the efficiency of topo cloning will become essential for the rapid development of gene therapies and synthetic organisms.
Integration with New Technologies
As technology progresses, integrating it with topo cloning will enhance the capabilities of this protocol. For example, the use of next-generation sequencing (NGS) technology allows for faster and more accurate sequencing of cloned DNA fragments. This integration can improve the confidence researchers have in their results. Moreover, automation in laboratories will streamline the cloning process, reducing human error and increasing throughput.
Recent advancements in CRISPR technology also show promise in conjunction with topo cloning. Researchers can use CRISPR for gene editing and subsequently utilize topo cloning for further manipulation and analysis of the target genes. This combination could lead to faster iterations in creating genetically modified organisms or studying gene functions.
Emerging Trends in Research
The future of topo cloning will also be shaped by the emerging trends in research. As synthetic biology becomes more popular, integrating topo cloning into synthetic pathways for metabolite production or gene circuit construction will be of increasing importance. Researchers are actively exploring how to insert multiple genes into a single vector more efficiently, which is made easier by lado technologies and enhanced topo cloning protocols.
Additionally, the demand for metagenomics and environmental genomics will likely direct research towards applying topo cloning in diverse ecosystems. By fully characterizing organisms in varied environments, scientists can leverage the strengths of topo cloning to understand complex microbial communities better.
Ending
The conclusion of this article serves as a pivotal summary that synthesizes the insights derived from the various discussions on topo cloning protocol. Understanding the key elements of the protocol, along with its advantages and limitations, is essential for researchers and practitioners in the field.
Summation of Findings
Through the course of this article, several critical findings were established. First, the mechanism of action involving topoisomerases and the choice of vectors significantly influence the success of cloning efforts. The step-by-step protocol provided clarity on the preparation of DNA fragments, ligation processes, and transformation into host cells.
Moreover, the versatility of topo cloning across various applications, including gene cloning, synthetic biology, and metagenomics, highlights its relevance in modern scientific inquiries. Although the technique boasts speed and efficiency, it also presents challenges, such as specificity issues and cost considerations. Ultimately, a balanced consideration of these elements is necessary for successful application.
Final Thoughts
In summary, topo cloning is more than just a methodological tool; it is an integral part of molecular biology research that continues to evolve. As new technologies emerge and methodologies become refined, the importance of staying informed about advancements in topo cloning cannot be overstated. The future promises great possibilities, including integration with technologies like CRISPR and broader applications in fields such as therapeutics and environmental science. Understanding these dynamics will empower the next generation of scientists and researchers to make informed decisions in their experimental designs and applications. In turn, this understanding can bolster the impact of scientific research on society as a whole.