Understanding Genetic Engineering: Concepts and Ethics
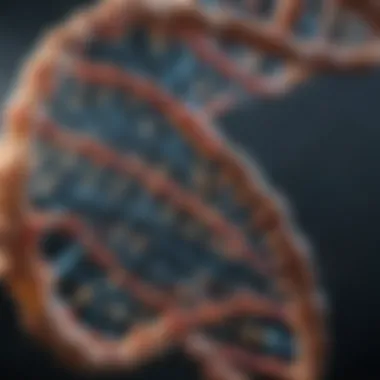
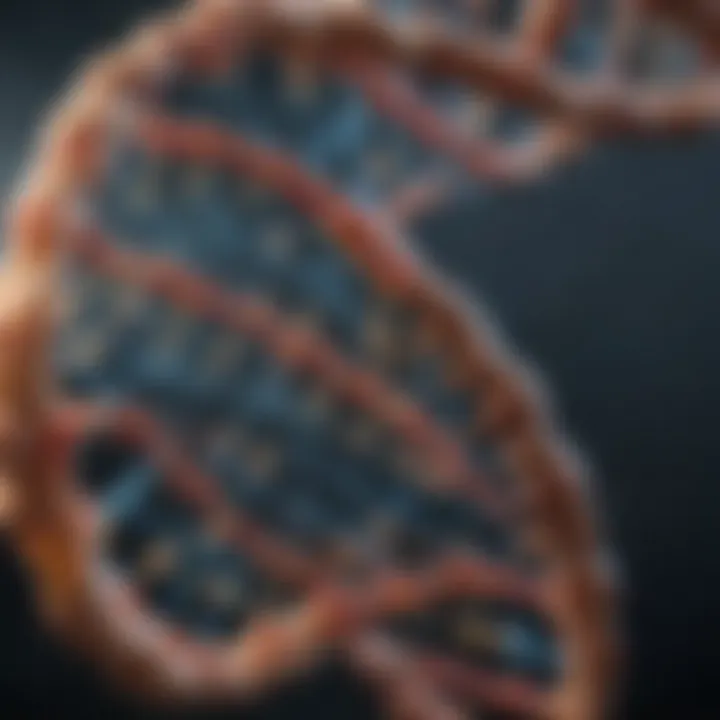
Intro
Genetic engineering stands as a pivotal development in the field of modern science. Its capability to alter the genetic makeup of organisms has led to profound changes in agriculture, medicine, and various other sectors. This ongoing advancement raises numerous questions about its implications, both beneficial and harmful. The goal of this article is to provide a thorough examination of the core concepts, applications, and ethical considerations surrounding genetic engineering. This exploration aims to inform students, researchers, educators, and professionals who seek to deepen their understanding of this complex field.
Article Overview
Purpose of the Article
The main objective of this article is to elucidate the numerous facets of genetic engineering. By outlining its principles and technologies, the article attempts to inform readers about the practical applications of genetic engineering and the ethical dilemmas it poses. The discussions presented will not only focus on current practices but will also be directed toward future possibilities in genetic manipulation.
Relevance to Multiple Disciplines
Genetic engineering resonates across various disciplines. It is crucial to biologists, geneticists, and medical professionals, but its implications extend to law, philosophy, and public policy. The incorporation of genetic engineering in agriculture, where crops are modified for better yields, highlights its importance in addressing food security. In healthcare, gene therapy represents a significant leap toward treating genetic disorders. Moreover, the socio-ethical discussions surrounding genetic modification underline its interdisciplinary relevance.
Research Background
Historical Context
The origins of genetic engineering date back to the early 20th century with the discovery of DNA as the hereditary material. However, it was not until the 1970s that practical techniques began to emerge. The first successful recombinant DNA technology experiments marked the start of a new era in biotechnology. Since then, tools such as CRISPR-Cas9 have dramatically accelerated the ability to edit genes.
Key Concepts and Definitions
Understanding genetic engineering requires clarity on several key terms and concepts:
- Genetic Modification: The deliberate alteration of an organism's genetic material.
- Gene Therapy: The introduction of genetic material into a person's cells to treat or prevent disease.
- CRISPR-Cas9: A powerful tool for editing genomes, allowing for modifications with precision.
- Transgenic Organisms: Organisms that contain genes from other species, often created to enhance specific traits.
As these terms suggest, genetic engineering operates at the intersection of technology and biology, shaping the future of numerous fields.
Prelude to Genetic Engineering
Genetic engineering is a crucial field that reshapes our understanding of biology and its applications. It involves manipulating an organism's DNA, offering the potential to enhance agricultural practices, improve medical treatments, and create industrial innovations. As technology evolves, the scope and implication of genetic manipulation continue to expand, which necessitates a comprehensive discussion on its fundamentals and impacts.
This section serves to orient readers with foundational aspects of genetic engineering, establishing why this topic merits attention. The principles of genetic engineering hold significance not only in scientific research but also in how society interacts with these advancements.
Definition and Scope
Genetic engineering is the direct manipulation of an organism’s genes using biotechnology. This could involve altering DNA sequences or modifying gene expressions. The scope of genetic engineering covers a wide array of areas, including plant and animal breeding, human genetics, and microbial engineering. The applications range from increasing crop yield to developing gene therapies or producing synthetic biological materials.
The field encompasses both techniques and applications, making it a versatile area of study. It is essential for students, researchers, and professionals to grasp the breadth of genetic engineering, as it holds the key to solving various challenges in health, food security, and environmental sustainability.
Historical Context
The history of genetic engineering is marked by significant milestones that have paved the way for modern practices. Early endeavours in genetics began with Mendel's work on inheritance patterns in the 19th century. However, it was not until the mid-20th century that DNA's structure was unveiled, allowing for more precise understandings of genetic material.
In 1973, the first successful gene transfer occurred, laying the groundwork for recombinant DNA technology. This breakthrough allowed scientists to splice genes from one organism into another, leading to the development of genetically modified organisms (GMOs). Since then, genetic engineering has continued to advance rapidly. The introduction of techniques such as CRISPR-Cas9 has made genetic modifications more efficient and accessible. Understanding this historical progression is vital, as it contextualizes current practices and ongoing debates about genetic engineering's ethical implications.
"Genetic engineering is not just a technical modification; it reflects our evolving relationship with nature and the potential consequences of altering life itself."
"Genetic engineering is not just a technical modification; it reflects our evolving relationship with nature and the potential consequences of altering life itself."
This brief look at the introduction, definition, and historical context of genetic engineering highlights the importance of this field. Its developments reflect human ingenuity and open up discussions on implementation and morality.
Basic Principles of Genetic Engineering
The principles of genetic engineering establish the foundation for understanding how genetic material can be manipulated to yield desirable traits in organisms. These fundamental concepts are essential for students, researchers, educators, and professionals engaging with modern biotechnologies. Understanding these principles helps clarify the potential applications and implications that arise from modifying genetic structures.
DNA Structure and Function
DNA, or deoxyribonucleic acid, is the carrier of genetic information in living organisms. Its structure is often described as a double helix, comprising two strands coiled around each other. Each strand consists of a sequence of nucleotides, which are made up of a sugar, a phosphate group, and a nitrogenous base. There are four types of nitrogenous bases in DNA: adenine, thymine, cytosine, and guanine.
The specific order of these nucleotides encodes genetic information, dictating how organisms develop and function. Misunderstandings in this area can lead to significant implications in genetic engineering; for instance, altering a single nucleotide can lead to mutations that can either confer advantages or cause disorders. Furthermore, understanding DNA's role in heredity and protein synthesis is crucial in the context of genetic modifications.
Gene Isolation Techniques
Gene isolation is the process of extracting a specific gene from the DNA of an organism. This technique is significant because it allows researchers to study individual genes in detail or employ them in genetic engineering projects. Common techniques include polymerase chain reaction (PCR), restriction enzyme digestion, and gel electrophoresis.
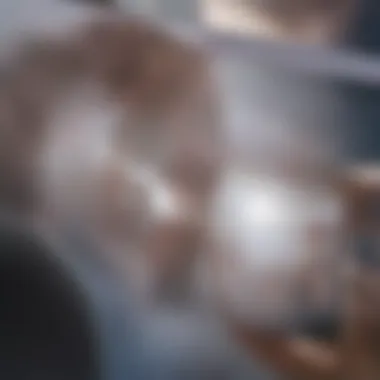
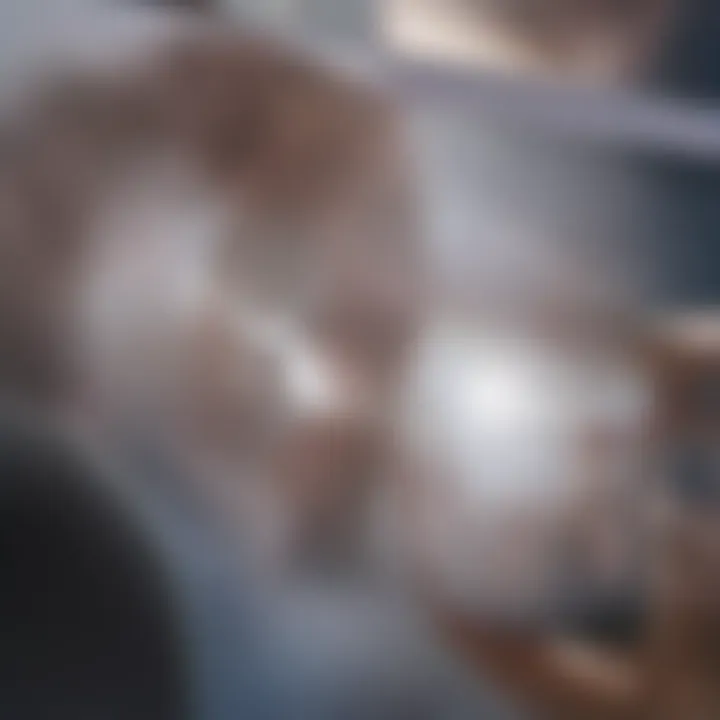
- Polymerase Chain Reaction: PCR amplifies small segments of DNA, making it easier to work with and analyze. It is widely used in various applications, including cloning, genotyping, and sequencing.
- Restriction Enzymes: These proteins cut DNA at specific sequences. By isolating genes, researchers can insert them into vectors for study or for engineering purposes.
- Gel Electrophoresis: This technique separates DNA fragments based on their size, allowing for visualization and purification of specific genes.
These techniques revolutionize biological research by enabling precise manipulation of genes, providing pathways for advances in therapies and agricultural improvements.
Gene Cloning and Sequencing
Gene cloning involves creating copies of a particular gene, often using vectors such as plasmids. This process enables researchers to produce large quantities of specific proteins or study gene functions without the complexities of an entire organism.
Sequencing, on the other hand, determines the exact order of nucleotides in a DNA fragment. Advances in sequencing technology, particularly next-generation sequencing, have made it possible to analyze entire genomes rapidly. This ability to clone and sequence genes has numerous applications – from creating genetically modified organisms to developing gene therapies for various diseases.
Understanding gene isolation, cloning, and sequencing strengthens the foundation on which genetic engineering stands, supporting innovations in both research and industry. As a result, these principles are crucial for navigating the ethical considerations and societal impacts of genetic alterations that follow.
Techniques in Genetic Engineering
The field of genetic engineering is fundamentally shaped by the various techniques utilized to manipulate genetic material. Understanding these techniques is essential for grasping how genetic engineering operates and its applications in different sectors. Each method has its unique characteristics, advantages, and ethical considerations. By examining these techniques thoroughly, readers can appreciate both the complexity and potential of genetic engineering in addressing contemporary challenges.
CRISPR-Cas9 Technology
CRISPR-Cas9 is one of the most advanced and revolutionary techniques in genetic engineering. It leverages a natural defense mechanism found in bacteria to edit genes with remarkable precision. The CRISPR system consists of two main components: the guide RNA, which directs the Cas9 enzyme to the specific segment of DNA that needs alteration, and the Cas9 protein, which acts as molecular scissors to cut the DNA.
The efficiency of CRISPR-Cas9 makes it a favored choice among scientists. It is not only more accurate but also cost-effective when compared to older methods like TALENs or zinc-finger nucleases. Its applications stretch from creating genetically modified organisms to potential therapeutic uses in human medicine, such as targeting genetic disorders.
However, there are concerns regarding off-target effects, which may introduce unintended mutations. This has sparked extensive research into refining the technique to ensure its safety and efficacy. Therefore, while CRISPR-Cas9 holds tremendous promise, it involves careful consideration to mitigate risks associated with gene editing.
Transgenic Organisms
Transgenic organisms are defined as those that have had a gene from another species introduced into their genetic makeup. This method has proven highly effective in agriculture and medicine. In agriculture, crops such as Bt cotton and Golden Rice have been developed to resist pests and improve nutritional value, respectively.
The process typically involves several steps, including the isolation of the desired gene, insertion into the host organism, and selection of successful integrations. This technique allows scientists to introduce specific traits, improving yield and enhancing resilience against environmental stressors.
Despite their benefits, transgenic organisms raise significant ethical issues. Public concern over biodiversity impacts and long-term ecological consequences must be addressed. Moreover, the potential for genetic discrimination and monopolization of food resources are critical considerations as we expand the use of transgenic organisms.
Gene Editing Tools
Beyond CRISPR-Cas9, other gene editing tools have emerged, expanding the toolkit available to genetic engineers. These include technologies like TALENs (Transcription Activator-Like Effector Nucleases) and zinc-finger nucleases. Each of these tools allows for targeted editing of specific genes, but they come with their own complexities.
TALENs utilize engineered proteins that bind to specific DNA sequences, whereas zinc-finger nucleases use zinc-finger motifs for DNA binding. While both techniques can edit genes effectively, they tend to be more time-consuming and expensive than CRISPR-Cas9.
The choice of gene editing tool often depends on the application. For instance, gene therapy methods might favor one technique over another based on how they interact with human cells. Understanding the nuances of each tool is critical for researchers aiming to leverage genetic engineering in innovative ways.
Utilizing diverse genetic engineering techniques enables scientists to push the boundaries of what is possible in agriculture, medicine, and industrial applications.
Utilizing diverse genetic engineering techniques enables scientists to push the boundaries of what is possible in agriculture, medicine, and industrial applications.
Applications of Genetic Engineering
The applications of genetic engineering encompass a broad range of fields that touch upon fundamental aspects of human life, economy, and ecological balance. Understanding these applications is key because they demonstrate the potential for genetic engineering to address pressing global issues, including food security, human health, and sustainable industrial practices. The benefits of these applications are extensive, as they offer innovative solutions that were previously unattainable with traditional methodologies. However, considerations regarding the ethical implications, risks, and societal perceptions of these applications remain critical in navigating the future of genetic engineering.
Agricultural Enhancements
Agricultural enhancements have emerged as one of the most significant applications of genetic engineering. Techniques such as genetically modified organisms (GMOs) allow for the introduction of specific traits into crops. These traits can include resistance to pests, tolerance to herbicides, and improved nutritional profiles. The benefits are evident; genetically engineered crops can increase yield production and reduce the need for chemical pesticides. However, they also raise concerns regarding biodiversity and the potential impact on non-target species. The integration of genetic engineering in agriculture must balance productivity with ecological responsibility.
Medical Therapies
Medical therapies utilize genetic engineering to create treatments that can change the course of various diseases. This area is rich with advancements and encompasses several distinct categories:
Gene Therapy
Gene therapy is a groundbreaking approach that seeks to treat disease by altering the genes inside a person's cells. It primarily addresses genetic disorders, cancers, and certain viral infections. The key characteristic of gene therapy lies in its precision; it targets the underlying causes of diseases rather than just symptoms. This makes gene therapy a particularly appealing option for those conditions that currently lack effective treatments. For example, by delivering corrected genes into a patient’s cells, gene therapy can potentially cure genetic disorders like cystic fibrosis or muscular dystrophy. However, there are significant challenges, including the risk of immune responses or unintended effects in other genes.
Vaccination Advances
Vaccination advances are another critical application of genetic engineering. The development of recombinant vaccines—vaccines produced from genetically engineered microorganisms—demonstrates how genetic modifications can enhance immunization processes. The key characteristic of these vaccines is their ability to provoke a strong immune response without causing the disease. This method provides a safer option than traditional vaccines. For instance, the recombinant Hepatitis B vaccine has significantly reduced the incidence of this virus worldwide. However, public hesitance towards genetically engineered vaccines sometimes poses challenges in achieving widespread immunization.
Bioengineering
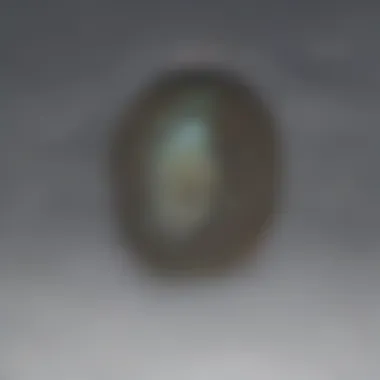
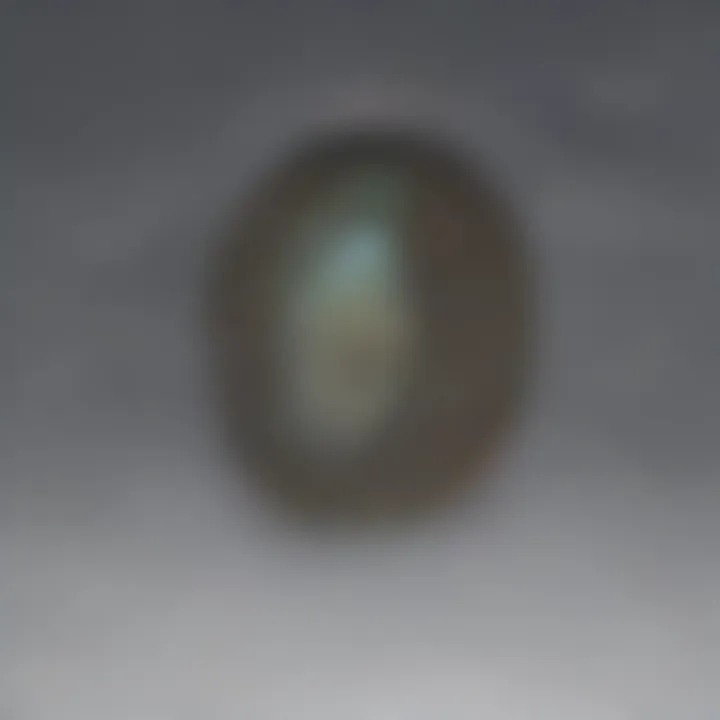
Bioengineering spans a broad range of applications within the medical field, including the development of biologically engineered tissues and organs. One of the key advantages of bioengineering is its potential to address the critical shortage of organ donors. By utilizing genetic engineering principles, scientists are exploring the possibility of growing organs in vitro that are tailored to match the recipient’s immune system. This notion of engineered organs presents groundbreaking advantages, such as reduced rejection rates and enhanced availability. The unique challenge, however, involves ensuring that these engineered solutions are functional and can integrate seamlessly within the human body.
Industrial Biotechnology
Industrial biotechnology applies concepts of genetic engineering to produce bio-based products. This can include things like enzymes for biofuels, biodegradable plastics, and bio-based chemicals. Utilizing microorganisms, scientists can manipulate their genetic structure to enhance production processes. This application is significant as it supports sustainability by offering alternatives to processes reliant on fossil fuels. The benefits of this approach are manifold, combining efficiency with reduced environmental impact. Nonetheless, industrial biotechnology must navigate regulatory and public scrutiny, particularly from those concerned about environmental impacts or ethical implications of such developments.
In summary, the applications of genetic engineering span multiple fields, offering considerable benefits while raising essential ethical and societal questions. Balancing these elements will be essential as advancements continue.
Ethical Considerations in Genetic Engineering
The study of ethical considerations in genetic engineering highlights a crucial aspect of this field. As advances in technology enable the manipulation of genetic material, it is imperative to evaluate the associated ethical implications. The integration of these considerations into genetic research affects public trust and scientific integrity.
One of the principal concerns is biosafety and biosecurity. These terms refer to the measures taken to prevent accidental release of genetically modified organisms and to protect individuals and the environment from potential harm. As genetic engineering techniques become more accessible, the importance of establishing strict safety protocols is magnified. Failure in biosafety could result in unintended ecological consequences or health risks. Therefore, regulatory frameworks must evolve to keep pace with scientific developments, ensuring safety in applications like agricultural biotechnology or gene editing therapy.
Biosafety and Biosecurity
Biosafety involves practices, procedures, and policies that mitigate risks associated with biotechnological work. In the context of genetic engineering, biosafety scrutinizes how modified organisms might impact biodiversity. Adverse effects may include the unintended spreading of engineered traits to wild relatives or non-target organisms.
Biosecurity, on the other hand, focuses on preventing misuse of biotechnological research. Threats range from biological warfare to bioterrorism, potentially exploiting vulnerabilities in genetic technologies. Thus, it's essential for scientific communities to implement comprehensive training, awareness, and readiness strategies to address these risks adequately.
Moral and Ethical Dilemmas
Genetic engineering not only presents technical challenges but also introduces moral and ethical questions that society must confront. These dilemmas often shape public perceptions and acceptance of genetic technologies.
Playing God Debate
The "Playing God" debate centers on the argument that altering genetic material encroaches upon natural processes. Critics argue that this manipulation raises questions about human responsibility and ethical boundaries. The key characteristic of this debate lies in the perception of unnatural interference with life.
Such views contribute to a broader discourse on whether human intervention should extend into fundamental aspects of life. Proponents of genetic engineering argue that the technology can lead to beneficial outcomes, such as eliminating genetic diseases or enhancing food security. The unique feature of the Playing God argument is its appeal to emotional and philosophical reasoning, provoking discussions about the limits of human innovation.
Genetic Discrimination
Genetic discrimination entails treating individuals differently based on their genetic information. This can manifest in various sectors, including insurance, employment, and healthcare. Genetic discrimination raises serious concerns about equity and access. The problem is pressing as more genetic testing becomes available, increasing the risk of individuals facing prejudice or exclusion based on genetic traits.
The key characteristic of this issue lies in the potential for unequal treatment, which can lead to a wider societal divide. Addressing genetic discrimination is essential for fostering a fair environment where individuals are not penalized for factors beyond their control. Thus, policies that protect against such discrimination are increasingly crucial in the age of biotechnology.
Access to Technologies
Access to genetic engineering technologies is not universally equitable. Disparities can exist based on geography, socio-economic status, and education. This raises ethical considerations about who benefits from advancements in genetic engineering. The unique feature of this topic involves the need for equitable distribution of technology, especially regarding life-saving therapies and agricultural enhancements.
It is pertinent to foster global cooperation to ensure that access is not limited to wealthier nations or privileged groups. Collaborations can contribute to shared knowledge and resources in the field, benefitting a broader population. Ensuring equitable access reflects the ethical obligation scientists have toward society.
Regulatory Framework and Oversight
The regulatory framework and oversight of genetic engineering are crucial in ensuring that scientific advancements are made responsibly, ethically, and safely. These regulations help govern the manipulation of genetic material, particularly in sensitive areas like agriculture, medicine, and biotechnology. Establishing clear guidelines helps mitigate potential risks associated with genetic modifications while promoting innovation. Understanding the regulatory landscape also assists stakeholders in complying with laws and policies, fostering public trust.
International Regulations
International regulations play a vital role in shaping genetic engineering practices across borders. Various global organizations, such as the World Health Organization and the Food and Agriculture Organization, set guidelines and standards for member countries. These regulations address safety protocols, environmental impacts, and ethical considerations in genetics.
One significant aspect of international regulations includes the Cartagena Protocol on Biosafety. This document addresses the transboundary movements of living modified organisms (LMOs). It aims to ensure that countries have the necessary measures in place to prevent adverse effects on biodiversity and human health. Compliance with such treaties promotes responsible use of biotechnological advancements on a global scale.
Additionally, international collaboration between nations enhances research efforts. For example, the OECD develops consensus documents which create a shared understanding of genetically engineered organisms. This cooperation aids in harmonizing regulatory processes, making it easier for companies and researchers to navigate the complex international landscape.
National Policies
National policies guide genetic engineering practices tailored to local contexts and conditions. Every country adopts its approach towards regulating genetic interventions, often influenced by public opinion, cultural values, and scientific advancements. These policies provide a framework for monitoring research, ensuring safety, and conducting ethical assessments.
In the United States, agencies such as the Environmental Protection Agency and the Food and Drug Administration oversee regulation regarding genetically modified organisms. These organizations assess the safety of new biotechnologies before they can be released into the market. They establish guidelines for research, field trials, and market approvals to protect public health and the environment.
Conversely, the European Union has a more stringent regulatory framework for genetically engineered products. The EU employs a precautionary principle, emphasizing safety assessments and transparency in the approval process. This approach often leads to longer approval times but aims to protect consumer interests and environmental sustainability.
Effective regulations can balance innovation and public safety, fostering confidence in genetic technologies while enabling scientific progress.
Effective regulations can balance innovation and public safety, fostering confidence in genetic technologies while enabling scientific progress.
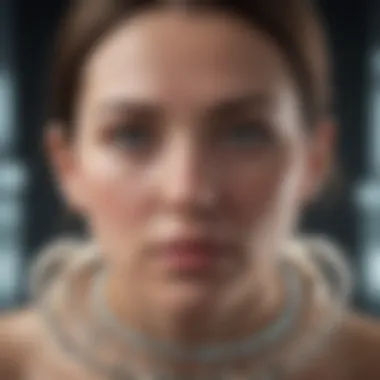
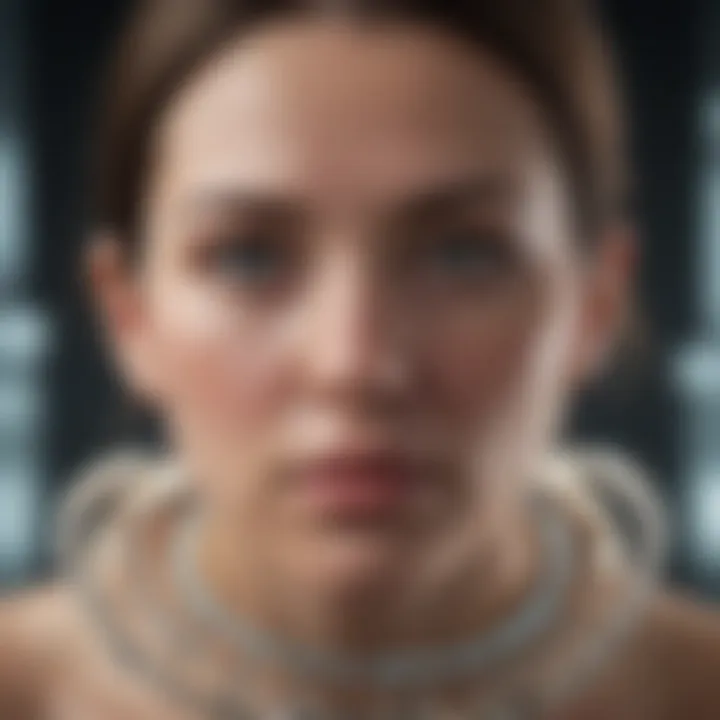
In summary, a robust regulatory framework is essential for the responsible advancement of genetic engineering. International regulations set the standard for cross-border collaboration, while national policies ensure that local contexts are also considered. Together, these components create a comprehensive oversight system essential for ensuring the benefits of genetic engineering are maximized while mitigating potential risks.
Societal Implications of Genetic Engineering
The impact of genetic engineering on society is profound, influencing various aspects of daily life, policy, and the environment. Understanding these implications is crucial. Genetic engineering is not just a scientific pursuit; it reshapes how we think about health, food security, and ethical boundaries. We must navigate the benefits and risks it presents carefully.
Public Perceptions and Acceptance
Public perception plays a significant role in the advancement of genetic engineering. Acceptance varies widely across cultures and age groups. Factors influencing these perceptions include:
- Media Representation: How genetic engineering is portrayed in films and news outlets can shape public sentiment. Sensationalist accounts often cause fear and misunderstanding.
- Education: The level of understanding among the public about genetic technologies affects their acceptance. Better education usually leads to informed opinions and greater acceptance.
- Ethical Concerns: People often fear the unknown. Issues such as genetic modification in humans often spark debates about morality and playing God.
- Trust in Scientists: Public trust in scientists and governing bodies can impact acceptance levels. Controversies surrounding previous scientific practices have heightened skepticism.
Ultimately, fostering a positive dialogue about genetic engineering can help mitigate fears and enhance public acceptance. More public engagement is necessary in discussions, as it leads to a more informed society.
Impact on Biodiversity
Genetic engineering can both positively and negatively affect biodiversity.
- Positive Aspects:
- Negative Aspects:
- Genetically engineered crops can be designed for resilience against pests and diseases. This can reduce the need for chemical pesticides.
- Enhancements in nutritional value may contribute to fighting hunger and malnutrition globally.
- There are concerns over genetic monocultures. Relying heavily on a few engineered strains can reduce genetic diversity in agriculture, which is vital for adaptability.
- The introduction of genetically modified organisms can lead to unintended consequences in ecosystems. For example, the escape of engineered traits into wild populations may alter natural selection processes.
"The challenge is to ensure that biotechnology benefits humanity without compromising ecological integrity."
"The challenge is to ensure that biotechnology benefits humanity without compromising ecological integrity."
We need a balanced discourse around these implications as we continue to explore what genetic engineering means for future generations.
Future Directions in Genetic Engineering
The field of genetic engineering is evolving rapidly, with new discoveries and technologies emerging regularly. Understanding these future directions is crucial for grasping the potential benefits and implications of advancements in this area. As the capabilities and tools available to researchers expand, so does the scope of what can be achieved through genetic manipulation. The implications of this rapid advancement are profound, affecting various sectors such as healthcare, agriculture, and environmental conservation.
Emerging Technologies
One of the most anticipated areas of growth in genetic engineering involves emerging technologies that promise to revolutionize the field. These innovations include not only refined versions of existing methods but also entirely new approaches.
- Gene Editing Advancements: Techniques like CRISPR-Cas9 are expanding beyond their initial applications. New variants are being developed for increased precision and efficiency in editing genes. This includes tools such as CRISPR-Cas13, which targets RNA instead of DNA, offering potential in treating diseases that are caused by RNA viruses.
- Synthetic Biology: This area of science combines engineering principles with biology to design and construct new biological parts. Products like synthetic insulin and engineered microbes for biofuel production illustrate its promise. Future developments may lead to entirely synthetic organisms crafted for specific tasks.
- Gene Drives: These are genetic systems that increase the likelihood of a particular trait being passed on to offspring. They hold potential in controlling vector-borne diseases by modifying populations of mosquitoes to resist malaria, thereby impacting public health significantly.
These technologies highlight the diversifying tools that researchers will employ to advance the understanding and manipulation of genetic materials.
Potential Challenges and Opportunities
While the future of genetic engineering holds substantial promise, it also presents considerable challenges that need to be addressed.
- Ethical Concerns: With greater power comes greater responsibility. The ability to edit the human genome raises ethical questions about designer babies and the implications for genetic inequality. There is a risk of creating a societal divide between those who can afford genetic enhancements and those who cannot.
- Regulatory Hurdles: As new technologies emerge, existing regulatory frameworks may not adequately address the unique challenges they pose. There is a need for comprehensive policies that can assess the risks and benefits associated with these new technologies while promoting innovation.
- Unintended Consequences: Manipulating complex biological systems can lead to unpredictable outcomes. The potential for ecological disruption from genetically modified organisms requires careful consideration and rigorous testing before wide-scale release.
On the other hand, these challenges also present opportunities for interdisciplinary collaboration. By bringing together experts from genetics, ethics, law, and public policy, the field can develop strategies to mitigate risks while enhancing the positive impact of genetic engineering.
"The future of genetic engineering holds both promise and peril, demanding a balanced approach to harness its full potential while safeguarding against negative outcomes."
"The future of genetic engineering holds both promise and peril, demanding a balanced approach to harness its full potential while safeguarding against negative outcomes."
Culmination
In discussing genetic engineering, we reach a critical juncture in understanding its multifaceted nature. The concluding section serves to encapsulate key insights from our exploration of this complex field. Genetic engineering is not merely a scientific endeavor; it influences agriculture, medicine, and our ethical landscape. Its significance lies in the advancements it offers and the profound ethical dilemmas it raises.
Summary of Key Points
- Definition and Evolution: Genetic engineering has evolved significantly since its inception. From the simple classification of genes to advanced technologies like CRISPR, the field has seen remarkable growth.
- Applications Across Sectors: Various sectors benefit from genetic engineering. Agriculture sees crops engineered for resilience; medicine leverages gene therapy to address genetic disorders; and biotechnology uses engineered organisms for industrial applications.
- Ethical Considerations: The power to manipulate genetic materials raises moral questions. Issues such as biosecurity, genetic discrimination, and equitable access to technology are at the forefront of ethical debates.
- Regulatory Frameworks: Policy responses must evolve alongside technological advancements. Both international and national regulations seek to manage biosafety, yet challenges persist in their implementation.
- Future Prospects: Keeping an eye on emerging technologies is vital. Innovations hold promise, but they also present challenges that require careful navigation by scientists and policymakers alike.
Final Thoughts
Ultimately, understanding genetic engineering is essential for informed discourse. The implications of genetic manipulation permeate many layers of society. As this technology grows, so will public interest and concern.
It is vital to encourage discussions around its applications and ethical considerations. Educational initiatives must foster a balanced understanding of the potential benefits alongside the risks.
"The development of genetic engineering demands a continuous dialogue among scientists, ethicists, and society to ensure accountable progress."
"The development of genetic engineering demands a continuous dialogue among scientists, ethicists, and society to ensure accountable progress."
In summary, our exploration of genetic engineering reveals its potential to reshape our world significantly while urging us to reflect on our responsibilities as we embrace these scientific advancements.