Understanding Green Hydrogen Production Methods
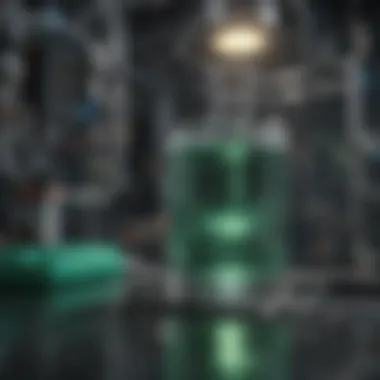
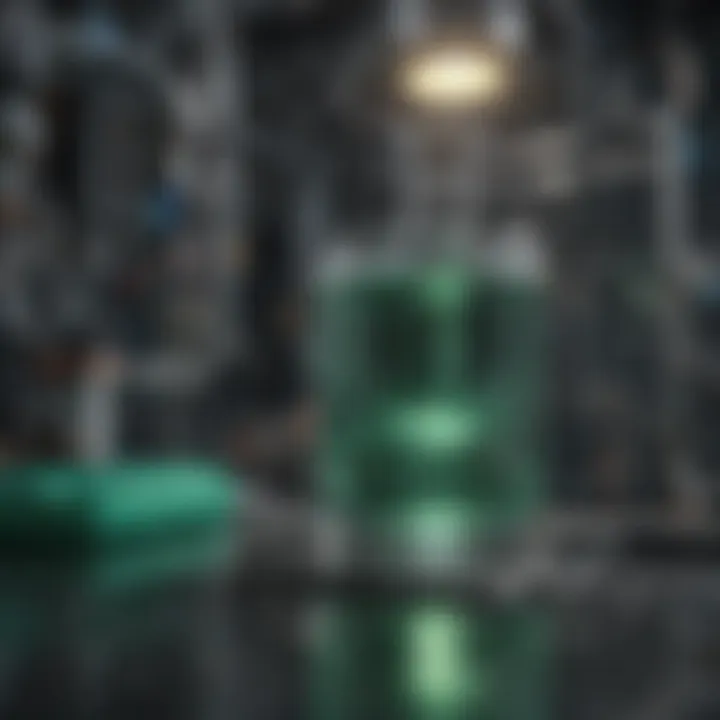
Intro
Green hydrogen has emerged as a cornerstone of sustainable energy initiatives. This type of hydrogen is generated from renewable resources, presenting a cleaner alternative to hydrogen from fossil fuels. By harnessing the power of water, sunlight, and organic matter, green hydrogen plays a critical role in reducing carbon emissions and combating climate change. Its varied production methods soon follow trends toward greater energy independence and reliability.
This article will provide an in-depth analysis of green hydrogen production. A thorough examination of the methods employed, such as electrolysis, thermochemical cycles, and biological processes, will be presented. Additionally, the discussion will highlight the challenges and prospects as the industry gears towards large scale implementation.
Article Overview
Purpose of the Article
The goal of this article is to inform and educate the target audience, which include students, researchers, educators, and professionals in the energy sector. It aims to outline the complexities and innovations surrounding green hydrogen production. By understanding the various methodologies, readers can better appreciate the implications for energy systems and the potential impact on multiple sectors.
Relevance to Multiple Disciplines
Green hydrogen intersects with numerous fields such as environmental science, engineering, and economics. Its production methods require interdisciplinary approaches, integrating scientific research with practical engineering solutions. By promoting sustainable alternatives, green hydrogen contributes to policies in energy management, climate change mitigation, and resource conservation.
Research Background
Historical Context
The concept of hydrogen as an energy carrier is not new. It has been explored since the late 19th century. However, the drive to produce green hydrogen gained momentum with rising concerns about fossil fuel dependency and environmental degradation. The evolution of renewable technologies, especially solar and wind power, has made green hydrogen production more feasible in recent years.
Key Concepts and Definitions
Green hydrogen is produced using processes that do not release carbon into the atmosphere. Two key processes are:
- Electrolysis: The process of using electricity to split water into hydrogen and oxygen. This method can utilize electricity generated from renewable sources like wind or solar power.
- Thermochemical cycles: A series of chemical reactions that use high temperatures to produce hydrogen from water. It often relies on solar thermal energy.
In addition to these, biological methods such as photofermentation and dark fermentation also play a role in green hydrogen production.
"Green hydrogen is considered a neutral energy carrier. It releases only water when used, making it key for a sustainable future."
"Green hydrogen is considered a neutral energy carrier. It releases only water when used, making it key for a sustainable future."
The following sections will further dissect these methods, their implications for energy systems, and their role in achieving net-zero emissions targets. Understanding these aspects will equip readers with the necessary knowledge for navigating the future of energy production.
Prelude to Green Hydrogen
Green hydrogen has gained attention as a crucial component of sustainable energy solutions. Its production involves the use of renewable energy sources to create hydrogen, which serves as a versatile fuel. This section will explore the fundamentals of green hydrogen, its significance, and why understanding its production methods is essential in today’s energy landscape.
Defining Green Hydrogen
Green hydrogen refers to hydrogen produced through processes that are entirely powered by renewable energy, such as solar, wind, or hydroelectric power. Unlike conventional methods, which often rely on fossil fuels, green hydrogen generation is associated with minimal or no greenhouse gas emissions. This distinction makes it a key player in strategies aimed at achieving carbon neutrality. Hydrogen generated this way can be used in various applications, such as fuel cells for vehicles, industrial processes, and energy storage.
Understanding green hydrogen is vital for students, researchers, and professionals who wish to contribute effectively to energy advancements. As countries strive to meet their climate goals, the production and utilization of green hydrogen could redefine energy systems across the globe.
Importance of Renewable Energy Sources
The significance of renewable energy sources cannot be overstated when discussing green hydrogen. They provide the necessary energy for the electrolysis process, which is the most common method of producing green hydrogen. The transition from fossil fuels to renewables not only supports environmental objectives but also enhances energy security and economic sustainability.
Several benefits of renewable energy sources include:
- Environmental Impact: Using renewable energy dramatically reduces the carbon footprint.
- Energy Independence: Countries can rely more on domestic energy sources, decreasing dependence on imported fuels.
- Technological Innovation: The growth of renewable energy fuels advancements in other technologies, including hydrogen production.
Incorporating renewable energy into hydrogen production methods positions green hydrogen as a vital element in modern energy solutions.
"The integration of renewable sources into hydrogen production represents a pivotal shift toward sustainable energy systems."
"The integration of renewable sources into hydrogen production represents a pivotal shift toward sustainable energy systems."
Thus, grasping the connection between green hydrogen and renewable energy will aid in the development of effective strategies for reducing global carbon emissions.
Production Methods
The production methods for green hydrogen are fundamental to understanding its potential and viability as an alternative energy source. These methods vary widely and each has unique characteristics that impact their efficiency, scalability, and environmental benefits. It is essential to assess these methods to determine their practicality in a broader energy system. Ultimately, the effectiveness of green hydrogen hinges on these varied production techniques and their integration into current energy frameworks.
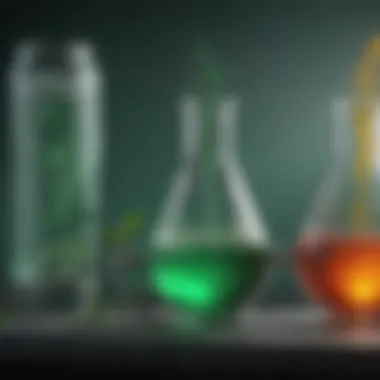
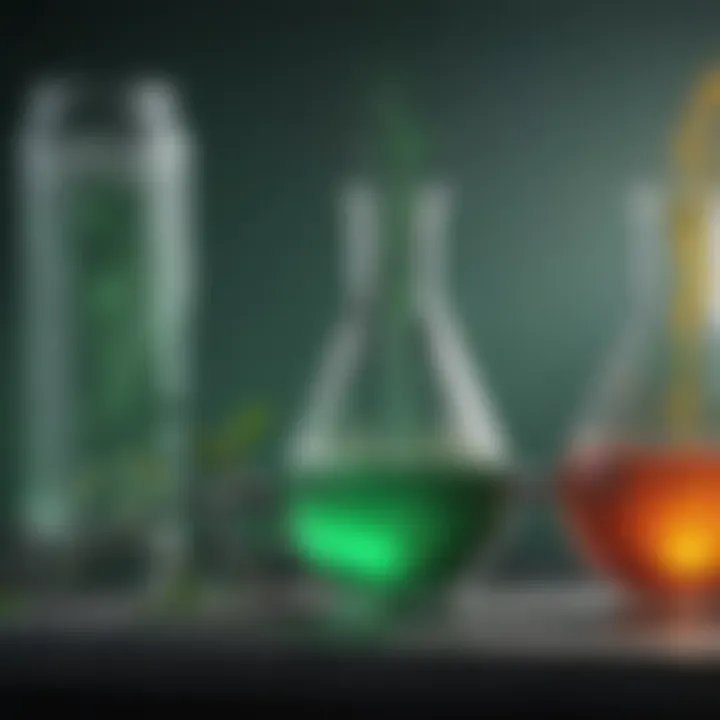
Electrolysis of Water
Overview of the Electrolysis Process
Electrolysis is a well-established technique for producing hydrogen by splitting water into hydrogen and oxygen using electricity. This process usually employs electrolyzers, which consume electricity to drive the chemical reaction. A key characteristic of electrolysis is its ability to utilize renewable energy, making it a preferred method for generating green hydrogen. Because it does not rely on fossil fuels, the process significantly reduces greenhouse gas emissions. Additionally, the method offers flexibility; it can be deployed at various scales, from small installations to large plants. However, electrolysis still faces challenges such as high operational costs and limited efficiency compared to some alternative methods.
Types of Electrolysis Technologies
Different types of electrolysis technologies exist, each with distinct processes and efficiencies. The two primary types are alkaline electrolysis and proton exchange membrane (PEM) electrolysis. Alkaline electrolysis, being the more mature technology, is widely used due to its lower costs and reliability. PEM electrolysis, although generally more efficient, has higher capital costs. The choice between these technologies often depends on specific applications and the availability of resources. Each technology has its own advantages such as alkaline's proven track record, and PEM's rapid response times to changes in input power.
Role of Renewable Energy
The role of renewable energy is central to the electrolysis of water. By using energy from sources such as wind or solar, we can produce hydrogen sustainably. This characteristic is beneficial in reducing reliance on fossil fuels in hydrogen production. Furthermore, renewable energy availability can drive the operating costs down of setups designed for electrolysis. However, one unique feature is the intermittent nature of some renewable sources, which can pose challenges to the consistent operation of electrolysis systems. Proper energy storage solutions may be required to handle supply fluctuations.
Efficiency Considerations
Efficiency is a critical factor when assessing electrolysis methods as it directly impacts the cost of hydrogen production. The efficiency of water splitting varies significantly across different technologies and conditions. Generally, the best systems achieve over 70% efficiency, although this is contingent on numerous factors like temperature and the quality of the electrolyzer. A notable aspect is that even slight improvements in efficiency can result in significant economic benefits at scale. The major challenge here is to continue improving these efficiencies while also preserving the sustainability of the energy sources used to power the electrolysis process.
Thermochemical Water Splitting
High-Temperature Processes
High-temperature processes represent an innovative approach within thermochemical water splitting. They leverage heat, often from concentrated solar power, to drive the chemical reactions necessary for hydrogen production. This method can achieve higher efficiencies than traditional electrolysis methods due to their dependence on heat. One significant advantage is that such processes can capitalize on existing thermal energy systems. However, the requirement for high-temperature materials and systems may impose safety challenges and increase costs.
Chemical Cycles Employed
Chemical cycles are essential elements of thermochemical water splitting. The most common cycles include the sulfur-iodine cycle and the copper-chlorine cycle. These cycles help maximize hydrogen yield while utilizing heat efficiently. A crucial benefit is that they can operate at different scales suited for various applications. However, specific materials for these cycles can be expensive and require significant research for development.
Temperature and Material Challenges
The temperature and material challenges in thermochemical water splitting are significant. The high temperatures required for optimal reactions can cause material degradation, limiting the operational lifespan of the systems. Additionally, finding cost-effective materials that can withstand these conditions remains a pressing issue. These limitations may hinder widespread adoption despite the method's potential advantages.
Biological Hydrogen Production
Microbial Electrolysis Cells
Microbial electrolysis cells utilize microorganisms to catalyze hydrogen production. This method is fascinating due to its capacity to operate under ambient conditions. Microbial electrolysis is gaining popularity for its lower energy input compared to traditional electrolysis. However, scalability and sustaining microbial populations over extended timeframes present challenges that must be addressed.
Dark Fermentation
Dark fermentation is a biological process that produces hydrogen through the breakdown of organic matter in the absence of light. This process is appreciated for its simplicity and ability to utilize waste materials as feedstock. The unique feature of dark fermentation is its ability to operate in a continuous system, making it appealing for large-scale applications. Challenges remain with regards to optimizing the hydrogen yield, which requires further research and development.
Light-Driven Processes
Light-driven processes utilize solar energy to facilitate hydrogen production via photosynthetic organisms. This approach holds promise due to its dual benefit of carbon dioxide sequestration and hydrogen production. However, the efficiency of light-driven processes often falls short compared to other methods. The ongoing advancements in this area can enhance its viability and attractiveness.
Advancements in Biotechnology
Recent advancements in biotechnology have opened new avenues for biological hydrogen production. Enhanced strains of microorganisms and synthetic biology techniques are being explored to improve yield and efficiency. This creates a more dynamic landscape for hydrogen production, promising greater capabilities and lower costs. Nevertheless, it is important to validate these technologies for commercialization to ensure they can meet energy demands effectively.
Comparative Analysis of Production Methods
The examination of different green hydrogen production methods is essential to determine the most effective and viable options. Each method presents unique characteristics, efficiencies, and challenges that can greatly influence the overall effectiveness in practical applications. By understanding these distinctions, stakeholders can make informed decisions about investments, technologies, and strategies for scaling hydrogen production. This section highlights significant aspects such as cost, scalability, and industrial applicability, all of which are critical to advancing green hydrogen as a leading solution in the energy transition.
Cost Analysis of Various Techniques
Cost is a pivotal factor when assessing hydrogen production methods. The financial implications determine not only feasibility but also the potential for widespread adoption in various sectors. Here, we will break down the costs associated with the main production techniques:
- Electrolysis of Water: This method’s costs include the price of electricity, electrolyzers, and water purification. Electrolysis efficiency rates can influence the total cost of hydrogen production. The price of renewable energy sources can fluctuate, further affecting the cost balance.
- Thermochemical Water Splitting: The expenses here are primarily related to high-temperature reactors and the materials required to maintain these systems. Investment in research can lead to lowering future costs, but current technologies may still present high initial financial barriers.
- Biological Hydrogen Production: This method often requires lower initial capital investment compared to others but can face challenges related to operational efficiency and scalability. Ongoing research is necessary to streamline costs associated with biotechnological approaches.
Understanding these cost dynamics is crucial, as they directly relate to the economic viability of implementing green hydrogen production at a larger scale. In turn, this influences policies and funding opportunities aimed at fostering the development of cleaner energy solutions.
Scalability and Industrial Application
The capacity for scaling production methods is a fundamental consideration for implementing green hydrogen on a commercial scale. Each production technique offers varying degrees of scalability:
- Electrolysis: While it can be deployed in small-scale projects, large-scale hydrogen production is achievable with appropriate renewable energy systems in place. However, as demand increases, it becomes vital to optimize electrolyzer efficiency and reduce costs through technology advancements.
- Thermochemical Processes: These may provide high output in controlled environments. However, challenges with materials and safety regulations can impede swift scalability. Moreover, extensive research is needed to develop practical applications suitable for industrial settings.
- Biological Hydrogen Production: This method shows potential, particularly in agricultural applications where organic waste can be leveraged. Nonetheless, the pace of scalability remains uncertain given current technology limitations. Ongoing innovations in biotechnology may help bridge this gap.
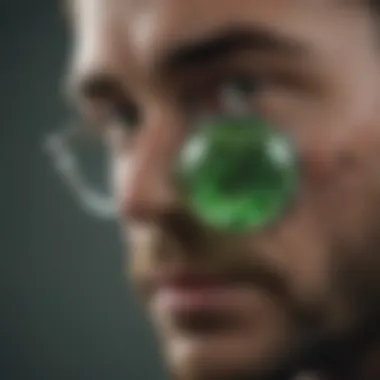
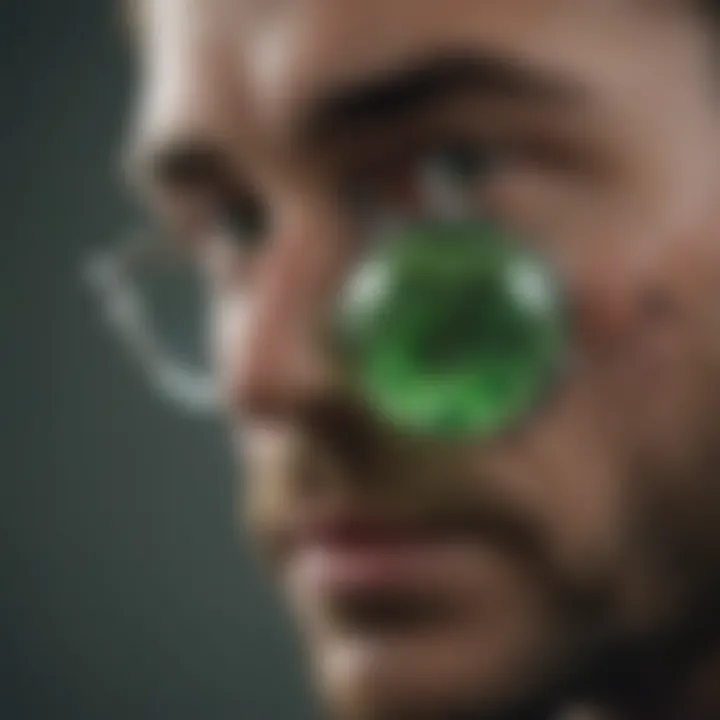
Challenges Facing Green Hydrogen Production
The journey towards a sustainable energy future through green hydrogen is fraught with challenges. Understanding these challenges is crucial for assessing the viability and optimization of green hydrogen production methods. Addressing economic, technological, and infrastructure-related obstacles can make a significant difference in the role green hydrogen would play in future energy systems.
Economic Viability
One of the main considerations in green hydrogen is its economic viability. Currently, the production costs associated with green hydrogen remain high. This is largely due to the relatively high price of renewable energy inputs and the complexity of production technologies. Many experts argue that lowering costs can enhance competitiveness against traditional hydrogen sources, primarily derived from fossil fuels.
Several approaches could improve economic viability:
- Incentives and subsidies: Governments can promote investment through financial incentives to boost green hydrogen projects.
- Economies of scale: As demand increases, larger facilities can spread fixed costs over more units of production, reducing per-unit costs.
- Technological improvements: Advancements in electrolysis and other technologies might lead to increased efficiency and reduced costs.
In summary, achieving economic viability in green hydrogen is essential for its adoption and long-term sustainability.
Technological Barriers
Technological barriers are another significant issue facing green hydrogen production. While the adoption of technologies such as electrolysis is crucial, challenges remain in optimizing processes. Efficient electrolysis systems, for instance, require significant advancements in membranes and electrodes. These advancements can lead to higher efficiency and lower energy input requirements.
Furthermore, various technologies under development may offer alternative methods for hydrogen production:
- High-temperature electrolysis: This method leverages heat to improve efficiency.
- Thermochemical cycles: Innovations in this area could open new pathways for hydrogen generation from heat and chemical reactions.
- Biological systems: While promising, biological methods still face scalability issues.
Working through these technological barriers is imperative to make green hydrogen production efficient and practical.
Infrastructure Requirements
Infrastructure signifies another critical challenge for green hydrogen production and deployment. For green hydrogen to become a significant part of the energy landscape, robust infrastructure must be in place. This includes facilities for production, storage, and distribution. However, current hydrogen infrastructure is limited and often reliant on existing fossil fuel systems.
Key aspects of infrastructure requirements include:
- Production plants: There are not enough dedicated facilities for large-scale green hydrogen production.
- Storage solutions: Storing hydrogen is complex. Innovative materials and techniques are needed to handle the gas safely and efficiently.
- Transport systems: Infrastructure to transport hydrogen to end-users is still underdeveloped. Pipeline networks and transport logistics need significant advancements.
Developing strong infrastructure for green hydrogen can enable widespread distribution and accessibility.
Addressing these challenges represents not just a hurdle, but an opportunity to innovate and rethink current energy paradigms.
Addressing these challenges represents not just a hurdle, but an opportunity to innovate and rethink current energy paradigms.
The successful resolution of these economic, technological, and infrastructure-related challenges can pave the way for a more viable and widespread adoption of green hydrogen in the global energy mix.
Environmental Impacts
The environmental impact of green hydrogen production is crucial in assessing its viability as a sustainable energy source. Green hydrogen, derived from renewable sources, offers a pathway to reduce greenhouse gas emissions and minimize dependency on fossil fuels. The production methods, from electrolysis to biological processes, each have unique implications on the environment. It is vital to examine how these methods contribute to a greener future and what measures can be implemented to ensure that the benefits outweigh any potential drawbacks.
Life Cycle Assessments of Green Hydrogen
Life cycle assessments (LCAs) of green hydrogen are instrumental in understanding its overall environmental impact. An LCA evaluates every stage of hydrogen production, from feedstock extraction to end-use. This method provides insights into energy consumption, resource use, and emissions throughout the hydrogen's lifecycle.
- Resource Extraction: Renewable energy sources used in green hydrogen production must be assessed for sustainability. The materials needed for technologies like electrolyzers and solar panels should have minimal ecological footprint.
- Production Process: The efficiency of different production methods influences the environmental impact. For example, water electrolysis powered by wind or solar energy typically has lower emissions than traditional methods using fossil fuels.
- Distribution and Usage: The logistics of transporting green hydrogen and its final utilization in various sectors—like transportation and industry—also factor into its environmental footprint. Efficient distribution systems can prioritize minimizing emissions.
"A comprehensive life cycle assessment is key to understanding the true sustainability of green hydrogen."
"A comprehensive life cycle assessment is key to understanding the true sustainability of green hydrogen."
Through LCAs, stakeholders can make informed decisions about adopting green hydrogen technologies while addressing concerns about ecological impacts. The findings can direct investments toward more sustainable practices in production and ensure better regulatory frameworks are established.
Potential for Carbon Footprint Reduction
One of the primary advantages of green hydrogen is its potential to significantly reduce carbon footprints across multiple sectors. The typical hydrogen production techniques contribute heavily to CO2 emissions. In contrast, green hydrogen has the potential to operate not only without producing carbon emissions but also to displace carbon-heavy processes.
- Transportation Sector: The shift toward hydrogen-powered fuel cells in vehicles presents a notable opportunity for reducing emissions compared to petrol and diesel vehicles. When green hydrogen is used, the only by-product is water vapor, marking a substantial cut in pollutants associated with conventional fuels.
- Industrial Applications: Industries such as steel and cement manufacturing are among the largest contributors to global carbon emissions. Utilizing green hydrogen as a substitute for fossil fuels in these processes can lead to a measurable reduction in greenhouse gases.
- Power Generation: Hydrogen can serve as a form of energy storage and a bridge to intermittent renewable sources like solar and wind. It can be converted back to electricity, potentially replacing coal and natural gas in power plants, thereby lowering emissions.
The integration of green hydrogen into these sectors illustrates its role as a catalyst for decarbonization. As policies and technologies develop alongside growing awareness of climate change, the transition to green hydrogen can play a salient role in shaping a low-carbon future.
Role of Green Hydrogen in Energy Transition
Green hydrogen plays a critical role in the energy transition to a more sustainable and low-carbon future. The rise in attention towards hydrogen stems from its potential to help decarbonize various sectors that are otherwise challenging to electrify. In this article, we explore the significance of green hydrogen as a versatile energy carrier and its impactful contributions across various energy landscapes.
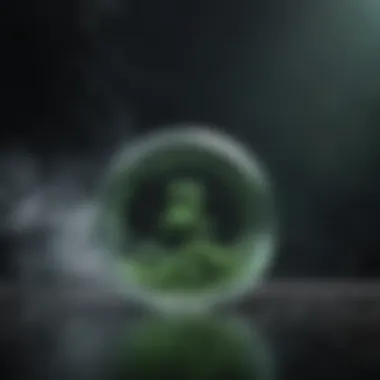
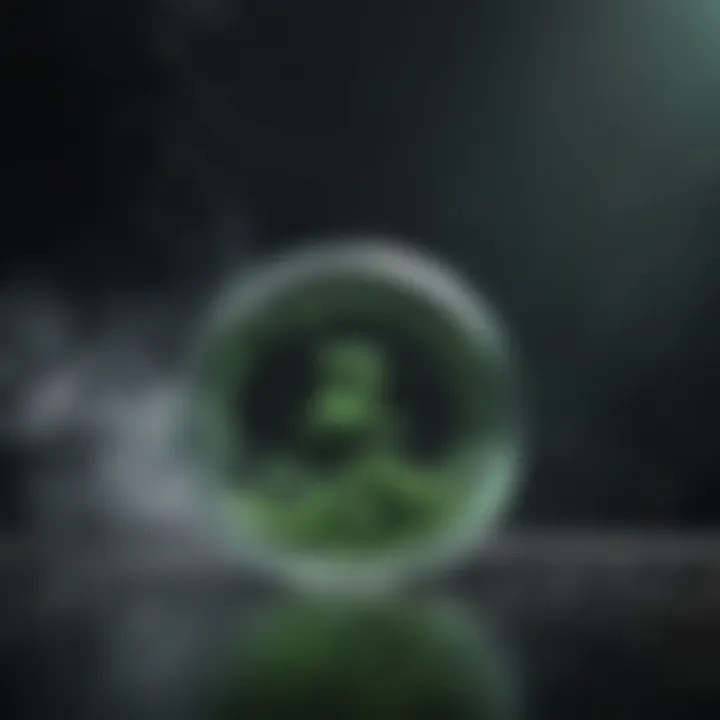
Integration with Renewable Energy Systems
The integration of green hydrogen with renewable energy systems is essential for harnessing the full potential of available resources. This process often involves utilizing surplus energy generated from renewable sources, like solar and wind, during peak production times. Rather than wasting this excess energy, it can be converted into hydrogen through electrolysis. This not only helps in managing energy demand but also enhances the utilization of renewable resources.
Key benefits of this integration include:
- Energy Storage: Green hydrogen acts as a means of storing energy that can be used later when renewable energy production might be low.
- Grid Stabilization: Hydrogen production can help balance supply and demand on the electricity grid.
- Decentralization: By deploying hydrogen technologies at various locations, it reduces the dependency on centralized power plants.
Furthermore, utilizing green hydrogen can encourage investments in renewable energy infrastructure, promoting a circular economy. The idea is to create a streamlined form of energy that transitions seamlessly from generation to consumption.
Applications in Transportation and Industry
The applications of green hydrogen extend across various sectors, particularly in transportation and industry. As a clean fuel, hydrogen offers a solution for reducing greenhouse gas emissions in areas where battery electric vehicles may not be practical.
In the transportation sector:
- Fuel Cell Electric Vehicles (FCEVs) are gaining traction. These vehicles utilize hydrogen and emit only water vapor, making them an environmentally friendly alternative to traditional combustion engines.
- Long-distance transport of heavy goods. Hydrogen-powered trucks can provide the necessary power without the weight penalties associated with batteries.
In industry:
- Steel Production: Traditional methods release significant carbon emissions. Hydrogen has the potential to replace fossil fuels in steelmaking, offering an emissions-free alternative.
- Chemical Production: Many industrial processes use hydrogen as a feedstock. Producing this hydrogen from renewable sources can significantly lower carbon footprints.
Future Outlook for Green Hydrogen
The development of green hydrogen is not only pivotal but also timely in the context of global energy transition objectives. As nations grapple with the pressing challenge of climate change, integrating green hydrogen into existing energy frameworks offers numerous opportunities. The significance of this topic lies in understanding its potential to reshape energy landscapes and contribute to sustainability.
As technology evolves, green hydrogen promises to become a cornerstone of future energy systems. Factors such as advancements in production technologies, economic competitiveness, and supportive policies will greatly influence its trajectory. Thus, investing in green hydrogen can lead to considerable economic and environmental benefits over the long term.
Technological Innovations on the Horizon
Emerging technologies are set to revolutionize the green hydrogen production process. Presently, electrolysis remains the leading method. However, new approaches are being explored which may enhance efficiency and bring down costs. For instance, proton exchange membrane (PEM) electrolysis, solid oxide electrolysis, and photo-electrochemical processes are making strides. These technologies promise improvements in both energy conversion efficiency and operational versatility.
Moreover, innovations in materials science are paving the way for more durable and efficient electrolyzers. Research into catalysts that require less energy or rare materials can significantly lower production costs, making green hydrogen more attractive to investors and stakeholders alike.
- Key Innovations to Monitor:
- Advanced Electrolytic Cells
- Catalysts and Membrane Innovations
- Integration with Solar and Wind Energy
With ongoing research and development, the sector may witness breakthroughs that could mitigate some of the current limitations associated with production scalability.
Policy and Regulatory Frameworks
The role of policy in fostering the growth of green hydrogen cannot be overstated. Governments across the globe are beginning to recognize the potential of green hydrogen in achieving energy security and environmental goals. Thus, suitable policies and regulatory frameworks are essential for facilitating investment and innovation.
Effective policies must address multiple facets:
- Financial Incentives: These can include grants, tax credits, and subsidies aimed at reducing the initial investment for green hydrogen projects.
- Standards and Regulations: Clear guidelines are necessary for the safety and efficiency of hydrogen production and usage.
- International Collaboration: Global partnerships can enhance technology transfer and create larger markets for hydrogen.
"Creating a coherent framework will help align public and private efforts in scaling up hydrogen technologies effectively."
"Creating a coherent framework will help align public and private efforts in scaling up hydrogen technologies effectively."
In summary, as green hydrogen gains momentum, sustainable policies and strategic frameworks will be essential in unlocking its full potential. A united commitment from various sectors can lead to a significant evolution in the energy landscape, positioning green hydrogen as a leader in the transition to a carbon-neutral future.
End
The topic of green hydrogen production holds significant importance in this article as it is central to understanding its role in sustainable energy solutions. Green hydrogen presents a vital opportunity to transition from fossil fuels to cleaner energy sources, and recognizing its production methods is key for stakeholders in energy, technology, and policy. An overview of the various techniques, such as electrolysis, thermochemical processes, and biological production, illustrates the diverse avenues for harvesting hydrogen sustainably.
Summarizing Key Insights
In summarizing the key insights from the discussions, it is clear that green hydrogen can vastly impact our energy landscape. The main points are:
- Diverse Production Methods: The article highlighted three primary production methods: electrolysis, thermochemical cycles, and biological processes. Each of these comes with its unique advantages and challenges.
- Challenges in Economic Viability: The costs associated with producing green hydrogen continue to be a barrier. Lowering production costs is essential to enable broader adoption.
- Environmental Considerations: The environmental benefits of hydrogen stem from its potential to reduce carbon emissions when replacing conventional fuels.
- Integration into Current Systems: For green hydrogen to be effective, its integration with existing infrastructures and renewable energy sources is critical.
These insights encapsulate the importance of continued innovation, investment, and policy support in advancing green hydrogen technology.
The Future of Green Hydrogen in a Sustainable Energy Landscape
Looking forward, the future of green hydrogen in a sustainable energy landscape seems optimistic yet challenging. As nations strive to meet climate goals, the emergence of robust policies and incentives will be crucial. Key considerations include:
- Technological Innovations: Continuous research and development will lead to more efficient production methods. Technologies improving electrolysis efficiency promise to enhance economic viability.
- Global Collaboration: International partnerships may facilitate sharing of knowledge, technology, and capital. This collaborative approach can drive advances in both production and use of green hydrogen.
- Policy Frameworks: Governments play a pivotal role in shaping the market for green hydrogen. Comprehensive regulations could support the infrastructure required for hydrogen production and distribution.
Green hydrogen can become a cornerstone in achieving a sustainable energy system. With known benefits and ongoing research, its role could significantly shape future economic and environmental scenarios.