Understanding Medium pH Across Scientific Disciplines
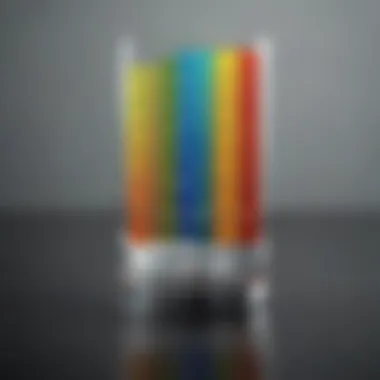
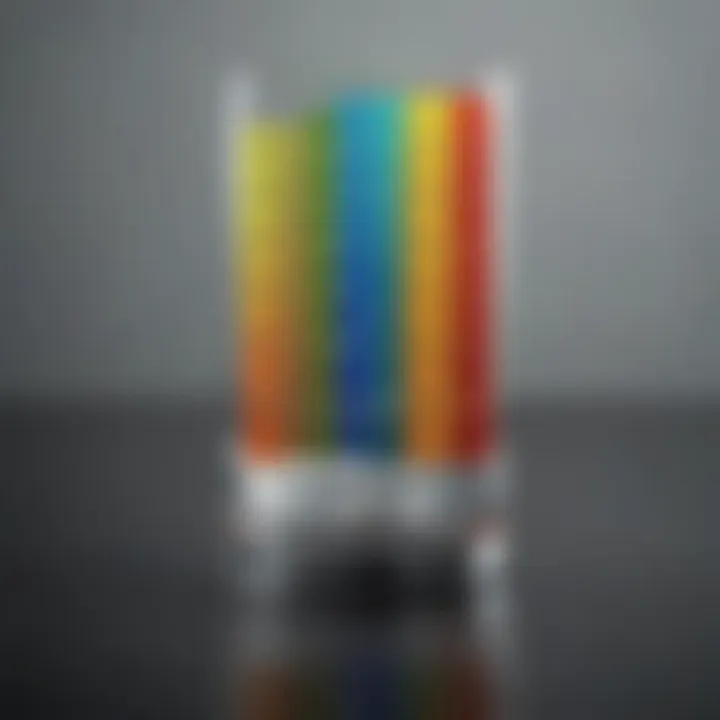
Intro
The concept of medium pH is critical across several scientific disciplines. Understanding its implications can enhance research, inform experiments, and drive innovations. This article explores different dimensions of pH within biology, chemistry, and earth sciences.
Article Overview
Purpose of the Article
The primary aim of this article is to delve into the significance of medium pH. Its importance in experimental and real-world applications cannot be overstated. By examining various facets of pH, we seek to provide a comprehensive resource for students, researchers, educators, and professionals.
Relevance to Multiple Disciplines
Medium pH has essential applications in diverse fields, including:
- Biology: pH levels greatly influence enzymatic activities, cellular functions, and metabolic processes.
- Chemistry: Understanding pH is fundamental for characterizing chemical reactions and solutions.
- Earth Sciences: pH impacts soil composition, water quality, and ecosystem health.
These areas reveal that an accurate understanding of pH can lead to significant advancements in research and practical applications.
Research Background
Historical Context
The concept of pH was first introduced in the early 20th century. Danish chemist Søren Sørensen developed the pH scale to measure acidity and alkalinity, providing a crucial tool for scientific inquiry. Over time, the understanding of pH has evolved significantly, leading to further discoveries in various scientific domains.
Key Concepts and Definitions
To fully grasp medium pH, one must be familiar with several key terms:
- pH Scale: Ranges from 0 to 14, with 7 being neutral. Values below 7 are acidic, while values above 7 are alkaline.
- Acidity and Alkalinity: Refers to the concentration of hydrogen ions (H+) in a solution. Higher concentrations indicate higher acidity.
- Buffer Solutions: Solutions that resist changes in pH upon the addition of small amounts of acid or base.
Understanding these fundamental concepts establishes a solid foundation for exploring the intricate role of pH in various scientific contexts.
"Precision in measuring pH is vital for obtaining reliable data across scientific experiments."
"Precision in measuring pH is vital for obtaining reliable data across scientific experiments."
If you would like to learn more, resources like Wikipedia, Britannica, and various scientific communities on Reddit provide deeper insights into pH and its relevance.
Prologue to pH and Its Measurement
Understanding the concept of pH is essential for numerous scientific fields. pH reflects the concentration of hydrogen ions present in a solution, influencing acidity or alkalinity. This chapter discusses why understanding pH measurement is crucial. It allows researchers and practitioners to monitor and control chemical reactions, biological processes, and environmental conditions.
Accurate pH measurement aids in providing insights that can guide experiments and industrial applications. Researchers can identify changes in chemical behavior, optimize nutrient availability in soils, or assess water quality. This makes pH a fundamental parameter in many areas of scientific inquiry.
Definition of pH
pH is a logarithmic scale used to specify the acidity or alkalinity of a solution. The pH value ranges from 0 to 14. A value of 7 denotes a neutral solution, while values below 7 indicate acidity and values above 7 indicate alkalinity.
The formula for calculating pH is given by:
[ pH = -\log[H^+] ]
Where ([H^+]) is the concentration of hydrogen ions in moles per liter. This formula provides a clear, quantitative measure of hydrogen ion activity in a solution.
Historical Context of pH Measurement
The term pH was first introduced by the Danish chemist Søren Sørensen in 1909. His work aimed to develop a standard method for assessing acidity. The idea quickly gained traction in scientific communities.
In the early 20th century, pH measurement primarily relied on simple acid-base indicators. However, advancements in technology have led to the development of more sophisticated methods, including electrode-based systems. These systems now dominate pH measurement in laboratories and industry.
Key Techniques for Measuring pH
Electrode-Based Methods
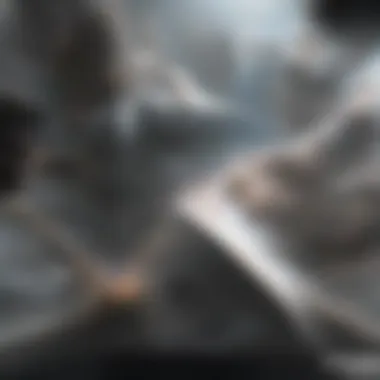
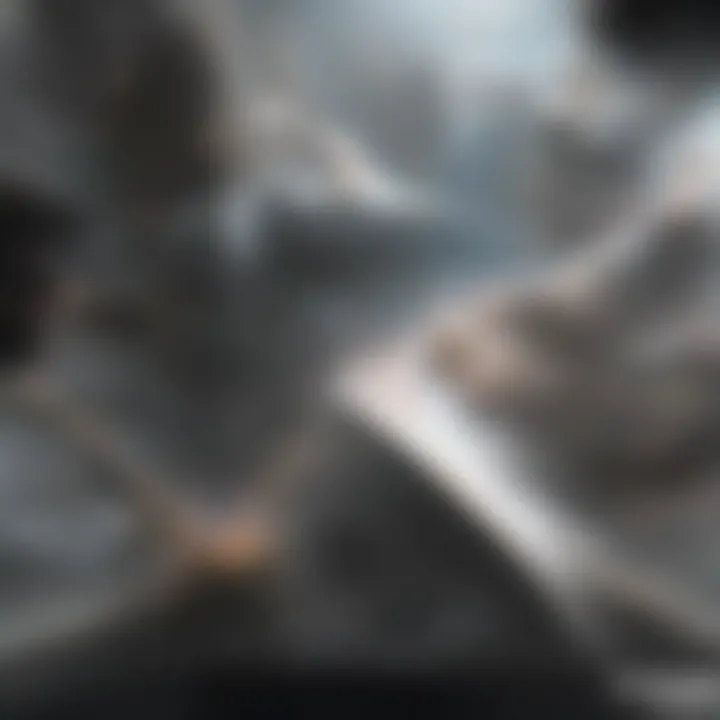
Electrode-based methods are the most widely used techniques for measuring pH. These methods employ glass electrodes, which are sensitive to hydrogen ion concentration. They provide rapid and accurate readings, making them a staple in laboratories worldwide.
The key characteristic of electrode-based methods is their ability to offer real-time data, which is essential during experiments. However, they require calibration and maintenance to ensure reliability. These electrodes can also be expensive.
Indicator Solutions
Indicator solutions involve using chemical dyes that change color according to the pH level of the solution. Common indicators include phenolphthalein and bromothymol blue. This method is straightforward and does not require complex equipment, making it accessible for educational purposes.
However, indicator solutions have limitations in precision. They typically provide only approximate pH values. Users must visually interpret color changes, which can introduce subjective bias in measurement.
Automated Systems
Automated systems for pH measurement utilize electronic sensors connected to computers or display devices. These systems offer high precision and can continuously monitor pH levels over time.
The key advantage of automated systems is their ability to operate in environments where frequent manual measurement is impractical. They also reduce human error associated with manual readings. However, these systems can be costly to set up and maintain.
Accurate measurement of pH is essential in ensuring safe and effective outcomes in scientific and industrial processes.
Accurate measurement of pH is essential in ensuring safe and effective outcomes in scientific and industrial processes.
The Science Behind pH: Understanding Acidity and Alkalinity
Understanding pH is crucial for a wide spectrum of scientific disciplines. It concerns not just chemistry but extends into biology, environmental science, and industrial applications. Comprehending the underlying principles associated with acidity and alkalinity can dramatically impact research outcomes and practical implementations. This section delves into the chemical mechanisms that underpin pH, detailing how they influence various systems and processes.
Chemical Foundations of pH
The term pH originates from the French "puissance d'hydrogène," which indicates the strength of hydrogen ions in a solution. pH measures how acidic or basic a solution is, on a log scale from 0 to 14. A pH value of 7 is considered neutral, while values below 7 indicate acidity, and those above signify alkalinity.
The hydrogen ion concentration is fundamental to understanding pH. For instance, a solution with a pH of 3 has a higher concentration of hydrogen ions compared to a solution with a pH of 5. This logarithmic nature means that a change of one unit in pH represents a tenfold change in hydrogen ion concentration. Hence, acidity and alkalinity are not merely descriptive but reflect significant chemical properties that determine reactivity, solubility, and biological functionality. Biological and chemical systems depend on maintaining specific pH ranges to function optimally, thus emphasizing the need for precise understanding and measurement.
Ionization and Proton Transfer
Ionization refers to the process where molecules gain or lose electrons, transforming neutral atoms or molecules into charged ones. In the context of pH, it frequently involves water molecules dissociating into hydrogen ions (H+) and hydroxide ions (OH-). This balance dictates the pH of a solution.
When acids dissolve in water, they release more hydrogen ions, thereby lowering the pH. For example, hydrochloric acid (HCl) dissociates completely in water to produce H+ ions, increasing acidity. Conversely, bases contribute hydroxide ions, which can capture hydrogen ions, raising the pH. For instance, sodium hydroxide (NaOH) behaves this way. The dynamic equilibrium of these ions fosters a continuous state of proton transfer, essential for many chemical reactions, including those in living organisms.
Proton transfer reactions are critical in many biological processes, such as enzyme function and metabolic pathways. They are often facilitated by surrounding molecules, which play a role in stabilizing or destabilizing these interactions.
Understanding the process of ionization and proton transfer is key in fields such as biochemistry, ecology, and pharmacology, influencing everything from enzyme efficiency to nutrient availability in ecosystems.
Understanding the process of ionization and proton transfer is key in fields such as biochemistry, ecology, and pharmacology, influencing everything from enzyme efficiency to nutrient availability in ecosystems.
Monitoring and manipulating pH is crucial in laboratory and industrial settings to optimize processes, such as fermentation in food production, or in drugs formulation within the pharmaceutical industry. Consequently, both pH management and understanding its science hold significant implications for a wide variety of disciplines.
pH in Biological Systems: Critical Roles
The significance of pH in biological systems is profound. It influences various aspects of cellular processes, metabolic pathways, and overall organism health. The intricate balance of pH can determine the efficiency of biochemical reactions, the structure of biological molecules, and even dictate the habitats organisms can thrive in. Understanding the role of pH is crucial for fields like microbiology, medicine, and environmental biology.
Cellular Functions and pH Levels
Cellular functions are meticulously controlled by pH levels. Most human cells operate optimally at around pH 7.4. Deviations from this range can cause cellular distress or dysfunction. For instance, acidic environments can lead to damage in cell membranes and macromolecules like DNA and proteins.
This balance of pH is essential for physiological processes.
- Cellular respiration: Enzyme activity in glycolysis relies on maintaining a stable pH.
- Signal transduction: Changes in pH can affect intracellular signaling, impacting cellular responses to external stimuli.
The proper regulation of pH within cells ensures metabolic efficiency and health, demonstrating the integrative role of pH in biological processes.
Impact on Enzymatic Reactions
Enzymatic reactions are highly sensitive to pH variations. Each enzyme has an optimal pH at which its activity is maximized. Deviations can lead to reduced activity or complete inactivation of enzymes. This is critical in pathways involving digestion and energy production.
For example, pepsin, an enzyme in the stomach, functions best at a low pH, around 1.5 to 2. On the other hand, alkaline phosphatase operates optimally at pH 9. Such differences highlight the need for precise pH maintenance in various cellular environments.
pH Regulation in Organisms

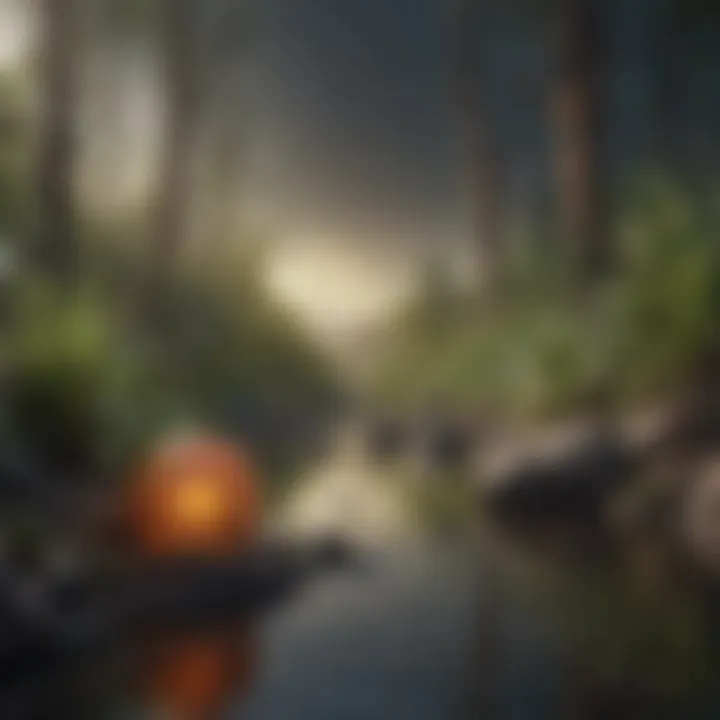
All organisms have developed mechanisms to regulate pH. This regulation occurs through various systems, from buffers in blood to specialized glands in organisms like humans.
- Buffer systems: Carbonic acid-bicarbonate buffer in blood maintains pH around 7.4.
- Glands: The pancreas secretes bicarbonate to neutralize stomach acid as it enters the small intestine.
The ability to regulate pH is not just a matter of homeostasis; it is vital for survival. For instance, a significant drop in blood pH can result in acidosis, leading to severe physiological complications.
Understanding how organisms maintain pH balance is crucial in medical and biological research. It also has implications for environmental science, as shifts in ecological pH can impact species resilience and biodiversity.
In summary, pH serves as a fundamental metric in biological systems. It dictates cellular functions, influences enzymatic activities, and is essential for the regulation of life processes. The implications of these relationships extend far beyond single organisms, affecting entire ecosystems and their sustainability.
Environmental Perspectives: Medium pH and Ecosystems
Understanding the significance of medium pH in ecosystems is essential. The pH level of the environment deeply influences the health of both soil and water systems. These aspects are crucial for biodiversity, agricultural productivity, and overall ecological balance. In exploring this topic, it becomes clear that the implications of pH extend far beyond simple acidity or alkalinity. Environmental variables, driven by pH levels, create a web of interconnections that can either promote or hinder life.
Soil pH and Plant Growth
Soil pH plays a fundamental role in plant growth. The acidity or alkalinity of the soil affects nutrient availability and root development. Most plants thrive in soils with a pH between 6.0 and 7.5. When pH levels fall below this range, essential nutrients like phosphorus become less available. In contrast, high pH can lead to deficiencies in micronutrients such as iron and manganese.
"Soil's pH balance determines nutrient uptake and influences plant health significantly."
"Soil's pH balance determines nutrient uptake and influences plant health significantly."
When assessing the impact of soil pH, it is crucial to consider specific plants. Acid-loving plants, such as blueberries, prefer lower pH values. On the other hand, plants accustomed to alkaline conditions might struggle in acidic soils. Gardeners and farmers must meticulously manage soil pH to foster optimal growth conditions. Strategies include applying lime to raise pH or sulfur to lower it.
Aquatic Systems and pH Dynamics
In aquatic environments, pH dynamics are equally important. Water bodies naturally exhibit a range of pH levels, influenced by atmospheric carbon dioxide, organic materials, and local geology. A stable pH is vital for aquatic life. For example, fish and invertebrates have specific pH ranges for health and reproduction. Deviations from these ranges can lead to stressed ecosystems, resulting in decreased species diversity.
The process of eutrophication, often driven by nutrient runoff, can lead to changes in pH through increased organic decay. When algae blooms die and decompose, they can lower the oxygen levels and alter the pH balance. Monitoring pH in lakes and rivers can inform conservation strategies to protect aquatic habitats.
Acid Rain and Its Effects
Acid rain presents a significant challenge to both terrestrial and aquatic systems. Formed through the emission of sulfur dioxide and nitrogen oxides into the atmosphere, acid rain can lower the pH of both soil and water sources. This change adversely affects biodiversity. For instance, certain fish species can only reproduce in specific pH conditions. Likewise, acid rain can strip the soil of essential nutrients required for plant health, leading to forest decline.
Key consequences of acid rain include:
- Decreased biodiversity: Species unable to adapt to rapid pH changes can be lost.
- Soil degradation: Nutrient leaching leads to diminished soil fertility.
- Habitat alteration: Aquatic ecosystems may become increasingly inhospitable.
Mitigating the effects of acid rain requires collaborative effort between industries, governments, and communities. Strategies include reducing emissions through cleaner technologies and improving regulations on pollutants.
Industrial Application of pH Management
pH management plays a crucial role across various industries. Understanding and controlling pH levels can significantly influence product quality, efficiency, and safety. This necessity arises from the fact that every industrial process has an optimal pH range, and deviations from this range can lead to detrimental outcomes. Therefore, effective pH management not only ensures compliance with regulatory standards but also enhances the overall operational efficiency of industrial practices.
Pharmaceutical Industry
In the pharmaceutical sector, pH plays a vital role in drug formulation and stability. Medications must be formulated at precise pH levels to ensure optimal drug absorption and efficacy. Furthermore, the solubility and bioavailability of active pharmaceutical ingredients are often pH-dependent.
Reduced pH can lead to degradation of certain compounds. For instance, ascorbic acid and penicillin are sensitive to changes in pH, primarily affecting their therapeutic properties. Consequently, manufacturers use specific pH testing equipment to monitor the conditions during production.
Quality control in pharmaceutical manufacturing demands rigorous pH measurement throughout the production phases. To ensure compliance with standards set by regulatory bodies like the FDA, facilities must implement robust pH monitoring systems.
Food and Beverage Processing
The food and beverage industry also relies heavily on pH management. pH is a critical factor influencing taste, preservation, and product safety. The acidity of various products can affect microbial growth and enzyme activity. For example, fruits and vegetables have different pH levels, which not only determine their taste but also their shelf life.
During fermentation processes, maintaining the correct pH is essential. Beverages like beer and vinegar require specific pH levels to ensure proper fermentation and flavor development. Furthermore, many preservation techniques, such as pickling, depend on acidity levels to inhibit bacterial growth. In this context, pH control ensures not only product quality but consumer safety as well. Regular testing and adjustments are standard practices in this industry.
Wastewater Treatment
Wastewater treatment is another key area where pH management is essential. The treatment process often involves chemical reactions that are sensitive to pH levels. For instance, the effectiveness of lime treatment to neutralize acidic wastewater relies heavily on maintaining the appropriate pH range.
If the pH is too high or too low, it can impede the effectiveness of the treatment, leading to inefficient removal of harmful contaminants. Moreover, pH impacts the solubility of heavy metals, thereby influencing the overall treatment process. Regulatory compliance demands that treated wastewater meets specific pH standards before release back into the environment. Therefore, continuous monitoring and management of pH are crucial to achieving successful wastewater treatment outcomes.

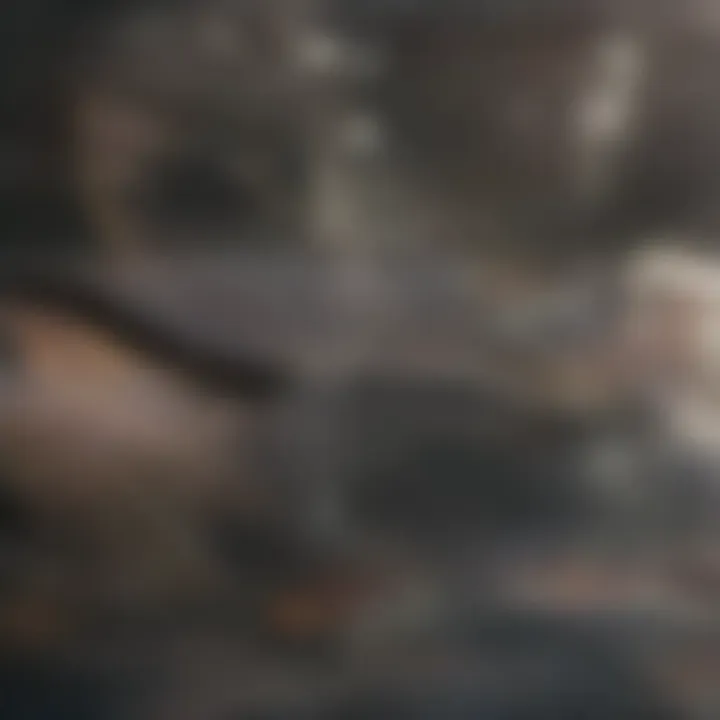
Effective pH management is not simply a routine practice; it is an essential component of quality assurance and product safety across various industrial sectors.
Effective pH management is not simply a routine practice; it is an essential component of quality assurance and product safety across various industrial sectors.
Current Research Trends in pH Studies
Research on pH is expanding, reflecting its vital role across various fields. Understanding the current trends in pH studies helps professionals grasp emerging methodologies and their implications on science and industry. The topic is relevant not just for academics but also for practitioners aiming to apply these findings in practical scenarios.
Innovations in pH Measurement Techniques
Recent advancements in pH measurement have transformed how researchers assess acidity and alkalinity in different mediums.
- Miniaturization of Sensors: Researchers have developed smaller, more portable pH sensors. These innovations allow for real-time monitoring in various environments, from laboratories to field settings.
- Smart pH Meters: Technologies have integrated smart sensors with connectivity features, enabling data collection and analysis using mobile devices. This change enhances precision and accessibility.
- Nanotechnology: Applications of nanomaterials in pH meters have improved sensitivity and response times, which is particularly essential in detecting minute pH changes that can significantly affect biochemical reactions.
These innovations not only streamline measurement processes but also enhance accuracy, facilitating more sophisticated research.
pH in Climate Change Studies
The understanding of pH's role in climate change is a growing area of study. The continuous rise of carbon dioxide levels in the atmosphere results in ocean acidification, impacting marine ecosystems.
- Impact on Marine Life: Studies indicate that declines in ocean pH disrupt calcium carbonate formation, essential for the survival of coral reefs and shellfish. This trend poses severe risks to biodiversity and fisheries.
- Feedback Mechanisms: Research also explores how changing pH levels can affect biogeochemical cycles, creating feedback loops that might exacerbate climate change.
- Mitigation Strategies: Current investigations aim at identifying ways to mitigate pH changes. These strategies are crucial for conservation efforts and developing sustainable practices in coastal zones.
By focusing on pH in climate change studies, scientists provide insights necessary for developing adaptive measures in mitigating environmental impacts.
"Understanding pH variations is crucial for predicting ecological outcomes as climate conditions evolve."
"Understanding pH variations is crucial for predicting ecological outcomes as climate conditions evolve."
The interplay of these research trends highlights the expanding scope of pH studies, emphasizing the need for continuous exploration in various contexts.
Future Directions: Challenges and Opportunities
The exploration of medium pH presents significant challenges and opportunities, critically influencing diverse scientific fields. Understanding pH is vital for both research and practical applications. As our world faces pressing issues, like climate change and environmental degradation, pH management becomes more essential. New developments in technology and sustainable practices aim to address these challenges, creating a systematic approach to monitoring and improving pH levels in various settings.
Advancements in technology have profoundly impacted pH monitoring. Professionals engaged in chemical analysis, environmental science, and biological research benefit from these innovations. Tools such as portable pH meters and smartphone applications facilitate accurate measurements in real-time. Moreover, wireless sensors can provide continuous data, enhancing our ability to track changes over time. This advancement is crucial for quick and informed decision-making, especially in industries like agriculture and water resource management.
Technological Advancements in pH Monitoring
Recent innovations in pH measurement techniques have revolutionized how scientists and industry professionals monitor pH levels. For instance, the development of optical pH sensors is gaining traction. These sensors offer an alternative to traditional electrode-based methods, often providing greater stability and accuracy over prolonged periods. They utilize light instead of electrical signals to measure pH, reducing interference from other ions in the solution.
Key Benefits of Optical pH Sensors:
- Durability: Long-lasting and less prone to damage than glass electrodes.
- Quick Response Time: Immediate feedback on pH changes, beneficial for dynamic environments.
- Less Calibration Required: Decreasing the frequency of recalibration saves time and resources.
Furthermore, the integration of artificial intelligence in monitoring systems holds promise. AI algorithms can analyze data patterns, predict pH fluctuations, and even suggest corrective measures, optimizing resource use.
The Role of pH in Sustainable Practices
Incorporating pH knowledge into sustainable practices is essential for environmental conservation. The role of pH extends beyond mere measurement; it influences choices in agricultural practices, waste management, and even energy production. By understanding the pH of soil, farmers can significantly enhance crop yields through proper amendment techniques. Likewise, industries engaged in wastewater treatment must manage pH to promote microbial activity that breaks down harmful substances efficiently.
Strategies for Sustainable pH Management:
- Regular Monitoring: Timely measurement of pH helps detect issues before they escalate.
- Use of Organic Amendments: Adjusting soil pH using natural products reduces chemical dependency.
- Community Education: Teaching local communities about pH's effects can foster grassroots involvement in sustainability efforts.
Ending: The Ongoing Importance of Medium pH
The examination of medium pH is critical not only in academic research but also in practical applications across various fields. Understanding pH levels can lead to improved outcomes in biological processes, environmental conservation, and industrial efficiencies. This article provides a comprehensive overview of the significance of medium pH, focusing on its essential roles and implications.
Summary of Key Findings
The exploration of medium pH revealed several vital points:
- Fundamental Role in Biological Systems: pH directly influences enzymatic activities, cellular functions, and metabolic pathways. Fluctuations in pH can disrupt these processes and have significant consequences on organism health.
- Environmental Impact: The pH level of soil and aquatic systems determines the biodiversity and health of ecosystems. For instance, acidic soils can hinder plant growth, while aquatic pH dynamics affect fish populations.
- Industrial Relevance: Accurate pH monitoring is crucial in industries such as pharmaceuticals, food and beverage, and wastewater treatment. It ensures product quality and regulatory compliance while enhancing operational efficiency.
Implications for Future Research
Future research on medium pH can expand in several meaningful directions:
- Innovative Measurement Techniques: There is a growing need for advanced pH measurement technologies that are both accurate and user-friendly. Research into nanotechnology and biosensors may provide significant advancements in this area.
- pH in Climate Change Studies: As global temperatures and CO2 levels change, understanding how these factors affect aquatic and soil pH is essential. Links between pH shifts and biodiversity loss warrant further investigation.
- Longitudinal Studies on Organism Adaptability: Long-term studies on how organisms adapt to varying pH levels can give insight into the resilience of species under changing environmental conditions.
Understanding medium pH is an ongoing journey with profound implications. As research continues to evolve, so will the strategies for managing pH in both natural and synthetic environments, underlining its centrality in scientific discourse.