Exploring the Complex Functions of Nerve Cells
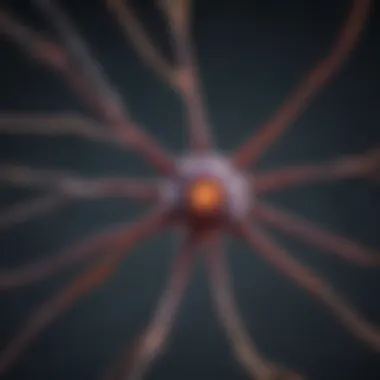

Intro
The nervous system is a vast and intricate network that plays a vital role in regulating nearly every aspect of human function. At the center of this complex system lie nerve cells, or neurons, that operate as the fundamental units for communication. Understanding how these cells work offers insights, revealing much about how we perceive, respond to, and interact with our surroundings.
Neurons are not solitary entities; rather, they form a web of connections which facilitate communication. Their ability to transmit signals rapidly is critical to processes ranging from voluntary muscle movement to involuntary reflexes. But to fully grasp their role, we must delve into their structural features, as well as their interaction with supporting cells called glial cells.
Article Overview
Purpose of the Article
This article aims to dissect the intricate functions of neurons, shedding light on their architecture and functionality. By exploring the mechanisms of neurotransmission and examining the roles of glial cells, we aim to enrich the reader's understanding of not just the neurons themselves, but also the entire nervous system. The significance of this exploration extends beyond mere academic inquiry; understanding neurons helps in the broader fight against neurological disorders.
Relevance to Multiple Disciplines
The study of nerve cells intersects with various fields, such as biology, psychology, medicine, and even computer science. From researching cognitive processes to understanding the biological underpinnings of diseases like Alzheimer’s, the implications are vast. Thus, the insights gained from this article can serve students, researchers, educators, and professionals aiming to expand their knowledge in these areas.
Research Background
Historical Context
The journey into understanding nerve cells is rich with historical breakthroughs. Pioneers like Santiago Ramón y Cajal were instrumental in establishing the neuron doctrine, positing that neurons are the basic functional units of the nervous system. This perspective was revolutionary and set the stage for modern neuroscience. As research progressed, various roles of different types of neurons were identified, emphasizing their specialized structures and functions.
Key Concepts and Definitions
To navigate this topic effectively, it helps to clarify some key concepts:
- Neuron: A cell that transmits nerve impulses; the fundamental building block of the nervous system.
- Glial Cells: Supporting cells that provide nourishment and protection for neurons. They play critical roles in maintaining homeostasis and aiding recovery after injuries.
- Neurotransmission: The process through which neurons communicate, often involving chemical signals called neurotransmitters.
- Synapse: The junction between two neurons that allows them to communicate.
Understanding these terms sets a concrete foundation for exploring the multifaceted functions of nerve cells in subsequent sections.
Overview of Nerve Cells
Nerve cells, or neurons, are the fundamental units of the nervous system, playing a pivotal role in how organisms interact with their environment and process information. Understanding nerve cells is crucial because they are the building blocks of both sensory perception and cognitive function. Without the intricate networking of neurons, life as we know it would not function. Think of neurons as the wires in an electronic circuit, allowing signals to flow and create meaningful outputs.
Definition and Importance
Nerve cells, or neurons, are specialized cells responsible for transmitting information throughout the body via electrical and chemical signals. Each neuron consists of a cell body, dendrites, and an axon. The importance of neurons cannot be overstated; they facilitate communication between different parts of the body, making it possible for organisms to respond to stimuli and coordinate complex behaviors.
A noteworthy aspect is their role in reflex arcs, where sensory neurons respond to immediate threats. For instance, if one accidentally touches a hot surface, sensory neurons quickly send signals to motor neurons, resulting in a rapid withdrawal of the hand. The intricate nature of neuronal function underlies everything from basic reflexes to advanced cognitive tasks, reinforcing the necessity of their study in neuroscience.
Historical Context
The understanding of nerve cells has evolved significantly over centuries. Early theories posited a more rudimentary view of neuronal function, often conflating nerve cells with mere conductors of electricity. The work of pioneers like Santiago Ramón y Cajal in the late 19th century was groundbreaking, as he used histological techniques to reveal neurons as individual entities rather than an interconnected network.
Moreover, the discovery of neurotransmitters by researchers such as Otto Loewi opened new avenues in understanding chemical signaling between neurons. Today, historical insights form a foundation for ongoing studies, helping link neuronal function with contemporary medical practices. The strides made in nerve cell research highlight the continuous journey toward decoding the complexities of the human brain.
"Neurons are the wiring that makes the human experience possible; without them, our thoughts, actions, and feelings simply could not exist."
"Neurons are the wiring that makes the human experience possible; without them, our thoughts, actions, and feelings simply could not exist."
In summary, nerve cells are not just a biological curiosity but a vital component that enables life functions. Their structure, function, and historical evolution underscore their significance in health, medicine, and understanding the brain. A comprehensive grasp of nerve cells fosters greater insights into neural disorders, paving the way for innovative therapeutic strategies.
Structure of Neurons
The structure of neurons is foundational to their role in the nervous system. Just like a well-built house needs a solid foundation, neurons rely on their unique architectures to effectively transmit signals throughout the body. Understanding this structure allows us to appreciate how neurons function, connect, and communicate.
Cell Body: The Soma
At the heart of the neuron lies the cell body, commonly referred to as the soma. This is where the nucleus resides, the control center that manages cellular activities and genetic information. The soma is filled with cytoplasm, which contains various organelles crucial for the neuron's maintenance and health. Without a properly functioning soma, the neuron would be unable to produce the proteins that facilitate signaling or repair its damaged components. Furthermore, the size and shape of the soma can differ significantly across various types of neurons, reflecting their specific functions within the nervous system. For instance, motor neurons often possess larger somas compared to sensory neurons, enabling them to support longer axons that reach out to muscles.
Dendrites: Receiving Signals
Dendrites function like the antennas of neurons, receiving signals from other neurons. They are tree-like extensions that branch out from the soma, increasing the surface area for incoming messages. The more branches a neuron has, the more signals it can receive, which is particularly important for integrating information from multiple sources. When neurotransmitters from neighboring neurons bind to receptors on the dendrites, they generate small electrical impulses that travel toward the soma. It’s fascinating to note that these dendritic branches can even change in response to experience, a process known as dendritic plasticity. This adaptability underlies learning and memory, contributing to the dynamic nature of the nervous system.
Axon: The Transmission Line
Once the soma processes incoming signals, the axon takes over to transmit these signals across long distances. Think of the axon as a highway, designed for high-speed travel of electrical impulses. The length of the axon can vary greatly; some axons can stretch over a meter, facilitating communication between distant parts of the body. The action potential, an electrical charge moving along the axon, is generated when the neuron reaches a certain threshold of excitation, allowing it to relay messages efficiently. Moreover, some neurons can even regenerate their axons, a trait that is crucial when considering injuries in the nervous system.
Myelin Sheath: Insulating the Signal
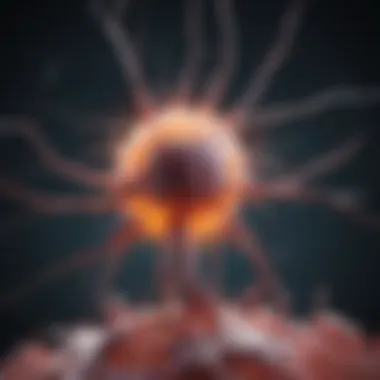
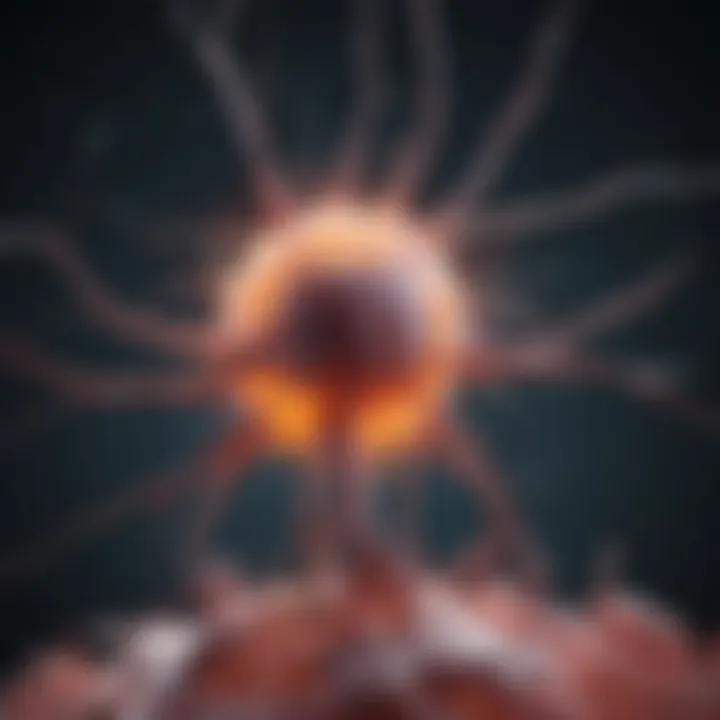
The axon’s speed and efficiency are significantly enhanced by the myelin sheath, a fatty layer that insulates the axon akin to rubber around an electrical wire. This specialized coating enables the efficient transmission of signals via saltatory conduction, where the action potential jumps from one node of Ranvier to another, decreasing the time it takes for the impulse to travel. It’s worth noting that disorders like multiple sclerosis occur when this myelin sheath is damaged, leading to a slowdown in signal transmission. Protecting this sheath is crucial for maintaining proper neuronal function and, by extension, overall nervous system health.
The structure of neurons, including the soma, dendrites, axons, and myelin sheath, not only defines their function but also determines how well the nervous system operates in maintaining bodily functions.
The structure of neurons, including the soma, dendrites, axons, and myelin sheath, not only defines their function but also determines how well the nervous system operates in maintaining bodily functions.
Through understanding these components, researchers and educators can better grasp how intricate neuronal communication and connectivity are. This knowledge not only sheds light on normal neuronal function but also provides insights into neurodegenerative diseases and other disorders, underscoring the importance of the neuronal structure in health and disease.
Types of Neurons
When delving into the complex world of nerve cells, the importance of understanding the different types of neurons cannot be overstated. Each type of neuron plays a critical role in how our nervous system operates, handling everything from basic reflexes to intricate cognitive functions. By dissecting the various forms of neurons, we gain insight into their specific roles and contributions to the greater whole of neurological function.
Sensory Neurons: Processing External Stimuli
Sensory neurons serve as the body's initial contact with the world. They are responsible for converting external stimuli into electrical signals which can be interpreted by the brain. These neurons are equipped with specialized receptors that respond to specific types of stimulus, whether it’s light, sound, touch, taste, or smell. For instance, photoreceptors in the retina respond to light, while mechanoreceptors in the skin detect touch and pressure.
This sensory input is crucial as it informs the brain about the environment. Without sensory neurons, our interactions with the world would be non-existent, effectively leaving us in the dark, so to speak. Imagine trying to navigate a crowded room without the ability to see, hear, or feel—this clearly illustrates their importance in everyday life.
Motor Neurons: Executing Movements
Motor neurons are the masterminds behind movement, transmitting signals from the brain and spinal cord to muscles and glands. When you decide to pick an object or walk, it's these neurons that spring into action. They translate the electrical impulses arising from the brain into physical action, making them essential for voluntary movements.
There are two main types of motor neurons: upper motor neurons, which originate in the brain, and lower motor neurons, which extend into the peripheral nervous system to connect with muscle fibers. Notably, the loss or dysfunction of motor neurons often leads to varied degrees of paralysis, showcasing their vital role in mobility.
Interneurons: Connecting the Dots
Interneurons are the communicators of the nervous system, serving as the bridge between sensory and motor neurons. They reside within the central nervous system and facilitate the transmission of signals, allowing for rapid reflexive responses and complex processing of information. A classic example can be seen in the reflex arc, where sensory input leads to an immediate motor response.
Interneurons come in diverse types and play varying roles—from those that enhance the processing of signals to others that inhibit them. This universality means they adapt exquisitely to the specific needs of neural circuits, making them crucial players in both simple reflexes and higher-level brain functions.
Specialized Neurons: Unique Functions
In addition to the primary categories, there exists a subgroup of specialized neurons, each tailored for unique functions. Some of these neurons exhibit characteristics that allow for specialized processing, such as the dual function of certain neurons in the retina that manage both rod and cone properties, thus influencing how we perceive light and color.
On another note, there are also neurons like the unipolar neurons, which primarily function within the sensory systems, delivering tactile information from the skin back to the central nervous system. These nuances highlight how diversity among neuron types enhances the overall functionality of the nervous system.
“The complexity of neuron types is matched only by the intricacy of their interactions, shaping everything we do from the simplest movements to the most profound thoughts.”
“The complexity of neuron types is matched only by the intricacy of their interactions, shaping everything we do from the simplest movements to the most profound thoughts.”
By comprehensively studying the different types of neurons, researchers can better understand how these cells contribute to the sophisticated actions and reactions that define human experience. In essence, grasping the roles of these neurons enriches our understanding of neuroscience and the intricacies of the nervous system.
Neurotransmission Mechanisms
Neurotransmission mechanisms form the backbone of how nerve cells communicate and interact with each other. This topic is significant as it touches upon the very essence of neural function, revealing how information travels through the nervous system. Understanding these mechanisms allows researchers, educators, and students to grasp the complexities of neural processes that underpin everything from reflexes to advanced cognitive functions.
Chemical Signaling: Neurotransmitters
Chemical signaling in the nervous system operates through neurotransmitters, which are small molecules that transmit signals across the synapse. When a neuron sends a signal, it releases neurotransmitters from its terminals. These molecules then traverse the synaptic cleft and bind to specific receptors on the post-synaptic neuron's membrane, which can initiate a response.
Neurotransmitters can be broadly categorized into excitatory and inhibitory types. Some prominent examples include dopamine, which plays a critical role in pleasure and reward pathways; serotonin, often linked with mood regulation; and gamma-aminobutyric acid (GABA), a chief inhibitory neurotransmitter that helps to calm nervous activity. Their balance is crucial; an overabundance of excitatory neurotransmitters could lead to hyperactivity or even neurotoxicity, while excessive inhibitors might result in lethargy or depression.
"Neurotransmitters not only convey messages but also modulate the nature of those messages, influencing mood, perception, and behavior."
"Neurotransmitters not only convey messages but also modulate the nature of those messages, influencing mood, perception, and behavior."
Electrical Signaling: Action Potentials
Action potentials are a crucial part of neuronal signaling involving electrical impulses that travel along the neuron's axon. These impulses begin when a neuron reaches a certain threshold potential due to an influx of positively charged sodium ions, depolarizing the membrane. The swift shift in voltage is what we call an action potential, a rapid wave of electrical activity that propagates down the axon.
Once the action potential reaches the axon terminals, it triggers the release of neurotransmitters into the synapse. This electrical aspect of neurotransmission ensures rapid communication, allowing the nervous system to function in real-time. Essentially, without action potentials, much of our neural processing would come to a grinding halt.
Synapses: The Communication Junctions
Synapses are specialized structures where the transmission of signals occurs between neurons. They are essentially the meeting points where neurotransmitters do their important work. A synapse can be compared to a bridge where one neuron sends signals across to another. The efficiency of this communication has significant implications for overall neural function.
There are two primary types of synapses: chemical and electrical. Chemical synapses are the most common and rely on neurotransmitters for signal transmission, whereas electrical synapses allow direct cytoplasmic connections through gap junctions, permitting faster communication. The structure of synapses facilitates a remarkable degree of modulation; the same neurotransmitter can have different effects based on the receptors present.
Plasticity: Adapting Neural Connections
Neural plasticity refers to the brain's remarkable ability to reorganize itself by forming new neural connections throughout life. This adaptability is critical for learning, memory, and recovery from injury. For instance, when you learn a new skill, synaptic connections can strengthen or weaken based on experience.
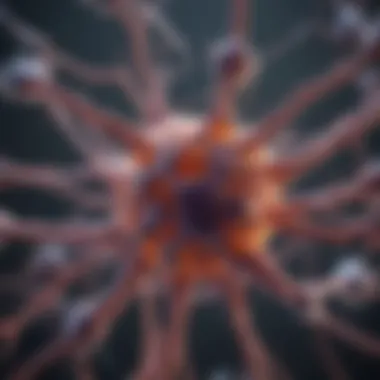
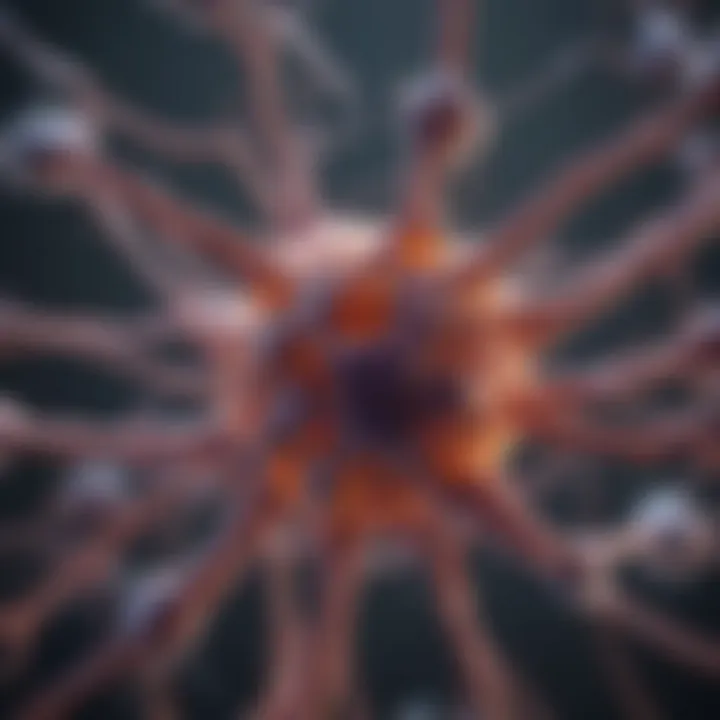
One well-known example of plasticity is synaptic potentiation, where repeated stimulation of a particular synapse can enhance its efficiency, making it easier for future signals to traverse the connection. Conversely, synaptic depression can occur when neural pathways are used less frequently, leading to weakened connections.
Broader implications of plasticity have been observed in rehabilitation settings, demonstrating that with the right stimuli, such as therapeutic exercises, individuals can retrain their brains to compensate for lost functions.
Understanding these mechanisms enhances our view of neuronal function and highlights the fine balance required for optimal neural communication. Through neurological research, we can unravel the complexities of how these processes not only facilitate basic cellular functions but also higher cognitive processes.
The Role of Glial Cells
Glial cells, often overshadowed by neurons in discussions about the nervous system, hold extraordinary importance. Without these silent supporters, neurons would struggle to perform their functions effectively. They serve as the unsung heroes of the nervous system, providing not just structural support, but also facilitating various critical processes that keep neurons in tip-top shape. This section uncovers the roles they play, showcasing how they contribute to neuronal health and function.
Definition and Types of Glial Cells
Glial cells, or neuroglia, are non-neuronal cells that maintain homeostasis, form myelin, and provide support and protection for neurons. Their definition extends beyond mere support; they actively participate in brain functions. Here are the main types of glial cells:
- Astrocytes: These star-shaped cells offer physical support to neurons. They help in the formation of the blood-brain barrier and regulate blood flow in the brain.
- Oligodendrocytes: Their main job is to create the myelin sheath that insulates axons in the central nervous system.
- Schwann Cells: Similar to oligodendrocytes but located in the peripheral nervous system, they also form myelin.
- Microglia: These are the immune cells of the brain. They help to eliminate waste and fight infections.
- Ependymal Cells: These cells line the ventricles of the brain and produce cerebrospinal fluid, which cushions and protects the brain.
Each type of glial cell plays a unique role in ensuring that neural networks function efficiently. Their diverse functions underscore their significance in maintaining a healthy nervous system.
Support Functions in Neuronal Health
Glial cells are akin to a nurturing gardener tending to a delicate garden of neurons. They not only provide the necessary nutrients but also serve a protective role. For instance, astrocytes regulate the extracellular environment by controlling ions and neurotransmitters around neurons, ensuring that they function optimally. This balancing act is crucial, as an uncontrolled environment can lead to neuronal dysfunction.
Moreover, these cells are pivotal in repairing and regenerating neurons after injury. When an area of the brain or spinal cord gets damaged, microglia rush in to clear out debris and pathogens, paving the way for healing. Without this swift action, the recovery process could be hampered.
Impact on Neurotransmission
Neurotransmission could be thought of as a relay race, where each runner must pass the baton smoothly to ensure a successful completion. Glial cells are essential for the success of this relay. They modulate neurotransmitter release and uptake, ensuring that communication between neurons is not just quick, but also precise. For instance, astrocytes can influence synaptic activity by controlling how quickly neurotransmitters are recycled, offering a fine-tuning capability that enhances synaptic efficiency.
In summary, glial cells are far more than passive support structures. They are deeply involved in neuronal health, playing a significant role in maintaining homeostasis, assisting in repair processes, and regulating the nuances of neurotransmission. Understanding their functions is imperative for a holistic view of how the nervous system operates, illustrating the delicate balance of collaboration between neurons and glial cells.
Neural Networks and Processing
The intricate world of neural networks plays a paramount role in understanding how nerve cells, or neurons, interact to facilitate communication within the human body. Neural networks are not merely clusters of neurons; they are dynamic systems that help interpret sensory data, coordinate motor functions, and support cognitive processes. By delving into this subject, we come to appreciate how interconnected neurons work in harmony to enhance our responses to everyday stimuli as well as complex decision-making processes.
Communicative Efficiency in Networks
Communicative efficiency within neural networks concerns how effectively and rapidly information is transmitted from one neuron to another. Each neuron operates as a node in a vast web of connections, with synapses serving as critical communication junctions. Here are some key points regarding the efficiency of these networks:
- Speed of Transmission: Action potentials, which are electrical impulses, convey information swiftly along axons. The presence of myelin sheaths significantly increases conduction speed, allowing for quick responses crucial in emergency situations.
- Signal Strength: Not all signals are created equal. Neurons can adjust their firing rates, affecting how strongly a signal is communicated. This ability ensures that important messages aren't drowned out by weaker signals, thus maintaining clarity in communication.
- Network Adaptability: Neural networks are remarkably adaptable, with the ability to strengthen or weaken connections based on experience and learning. This plasticity boosts communicative efficiency because it fine-tunes the pathways used for transmitting information over time.
In summary, the efficiency of neural communication is a cornerstone for overall brain function, impacting everything from reflexes to higher-order cognitive processes.
Distributed Processing: Beyond Individual Neurons
Distributed processing alludes to the concept that while neurons act as individual entities, their true power lies in collective operations across networks. This means that functions such as sensation, movement, and thought don't rely solely on one neuron but rather on intricate networks. Understanding this is essential for a comprehensive look at neuronal function. To elaborate:
- Parallel Processing: Neurons work in parallel to enhance efficiency. For example, when you touch a hot surface, many sensory neurons signal pain simultaneously, allowing for a quick reflexive withdrawal.
- Complex Function Integration: Different regions of the brain process varied aspects of a single experience. For instance, seeing a dog triggers visual processing in one area while also activating emotional centers; this integrated processing creates a nuanced understanding of the experience.
- Redundancy and Resilience: The distributed nature of neural processing affords resilience. If one pathway is damaged, alternate routes can often fill the gap, which is significant in injury recovery and rehabilitation.
Implications of Neuronal Function
The function of nerve cells, or neurons, extends beyond mere electrical signals and synaptic connections, influencing a wide array of processes that define our interactions with the environment and ourselves. Understanding these implications provides invaluable insights into how we perceive, respond, and think. This section will delve into how neuronal activity shapes our reality, regulate movements, and impact cognition.
Sensory Processing and Perception
Neurons play a pivotal role in sensory processing, acting as messengers that relay information from our senses to the brain. When light, sound, or physical stimuli engage our sensory receptors, it is the neurons that convert these external signals into electrochemical impulses.
Consider the following processes involved in sensory processing:
- Transduction: Sensory neurons convert stimuli into neural signals, a process crucial to perception. For example, photoreceptors in our eyes capture light and transduce it into signals sent to the brain.
- Integration: Once these signals reach the central nervous system, interneurons integrate and process inputs, ensuring we interpret what we are experiencing accurately. This might involve differentiating between the warmth of sunlight and the chill of shade.
The ability of the brain to construct an understanding of these signals highlights the intricate dance of neurons at play. They not only facilitate our interaction with the environment but also influence how we perceive emotions, color, sound, and taste, weaving a rich tapestry of human experience.
Control of Movement and Reflexes
Nerve cells are essential for controlling movements, whether voluntary or reflexive. Motor neurons deliver commands from the brain to muscles, initiating actions ranging from delicate finger movements to powerful leg thrusts. The connection between motor neurons and muscle fibers is a site of profound significance, facilitating seamless coordination of movement.
Reflex actions, such as pulling back a hand from a hot surface, demonstrate the rapid response enabled by neurons. Reflex arcs, which involve sensory neurons, interneurons, and motor neurons, allow for immediate reactions without the delay of routing signals through the brain. This is especially critical for survival, as it provides a protective mechanism against harm.
Cognition and Memory Formation
The implications of neuronal function extend deeply into cognition and memory. Neurons form complex networks that underpin our thoughts, decisions, and memories. The process of memory formation involves several stages:
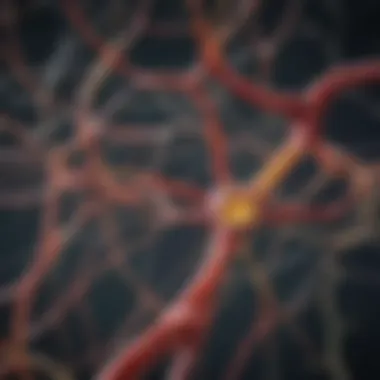
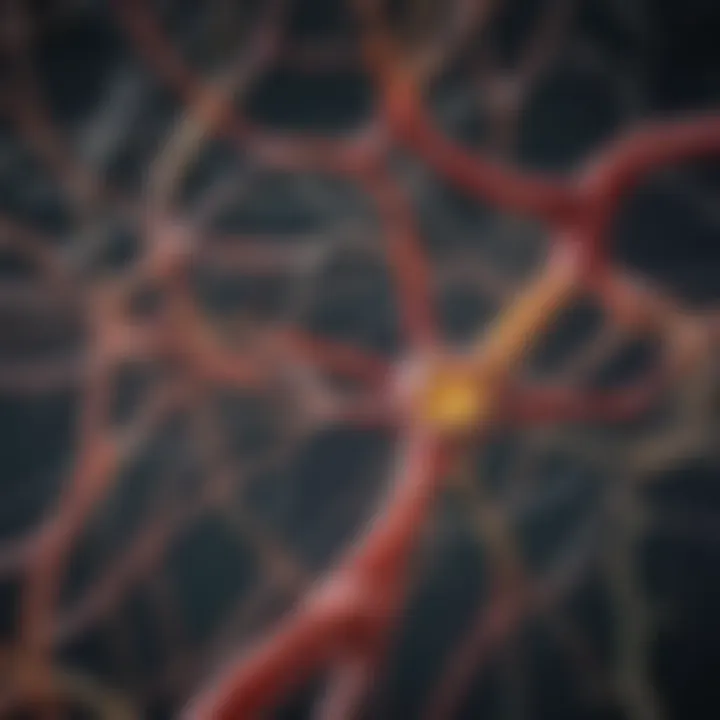
- Encoding: New information is transformed into a format suitable for storage. Different inputs activate distinct neural pathways.
- Storage: Long-term potentiation, a phenomenon where synaptic connections strengthen, allows memories to be solidified and retained.
- Retrieval: Upon recalling a memory, neurons activate in a pattern that mirrors the original experience, allowing us to relive moments in time.
Critically, impairments in neuronal function can lead to challenges in these cognitive processes. Disorders such as Alzheimer's disease or other neurodegenerative conditions underline the fragile nature of neuronal health and its impact on memory and cognition. Understanding these implications sets a foundation for ongoing research into therapeutic interventions aimed at restoring or enhancing neuronal function.
"Neurons are the storytellers of our experiences, shaping how we navigate the world and retain our memories."
"Neurons are the storytellers of our experiences, shaping how we navigate the world and retain our memories."
As we dissect the implications of neuronal function, it becomes evident that these cells are not simply biological structures; they are the bedrock of our sensory experiences, movements, and capacity for thought. The dialogue between neurons shapes our identity and influences every facet of our existence.
Neuronal Disorders
Neuronal disorders stand as a significant focus in neuroscience, as they encompass a variety of conditions impacting the brain and nervous system. These disorders range from mild cognitive impairment to severe diseases that can greatly affect daily functioning. By exploring neuronal disorders, we gain insights into how disturbances in neural functionality can influence behavior, perception, and physical abilities.
Understanding these disorders is essential not just for medical professionals, but also for educators, researchers, and those in caregiving roles. It could lead to improved treatment options, foster empathy for those affected, and fuel further research into novel therapies. As we delve further, we will uncover the complexities behind specific disorders that highlight the delicate balance of neural health.
Neurodegenerative Diseases
Neurodegenerative diseases, such as Alzheimer's and Parkinson's, involve the gradual degeneration of neurons, leading to significant cognitive and motor challenges. These conditions typically present a progressive decline in cognitive skills, memory, and physical abilities, disrupting the lives of those affected and their families.
In neurodegenerative diseases, the role of protein aggregation and inflammation is often at the forefront of research. For example, in Alzheimer’s, abnormal proteins accumulate in the brain, leading to neuron death. Symptoms can vary widely, but they often include difficulty remembering recent events, language problems, and impaired judgment. A critical point of understanding these diseases is the relationship between early diagnosis and intervention strategies.
- Key Characteristics of Neurodegenerative Diseases:
- Gradual progression of symptoms
- Cognitive decline and memory issues
- Potential genetic predisposition
"Understanding neurodegenerative diseases can open doors to better medical treatments and interventions that can mitigate symptoms and improve patients' quality of life."
"Understanding neurodegenerative diseases can open doors to better medical treatments and interventions that can mitigate symptoms and improve patients' quality of life."
Trauma and Injury Impacts
Trauma and neural injuries—be it from accidents, strokes, or other external factors—can lead to immediate and long-lasting alterations in neuronal function. Unlike neurodegenerative diseases, which develop over time, these impacts can lead to swift and, at times, irreversible damage. The aftermath often raises complications such as paralysis, seizures, or cognitive deficits.
The response of neurons to injury involves both physiological and psychological dimensions. For instance, while some neurons may regenerate or adapt, others may suffer permanent loss, leading to significant challenges in recovery. Rehabilitation plays a crucial role in recovery by helping patients develop new skills or relearning lost ones, highlighting the plasticity of the nervous system.
- Important Considerations in Trauma and Injury:
- Immediate medical intervention is crucial
- Rehabilitation is essential for recovery
- Long-term monitoring and support are often needed
Mental Health Disorders: Links to Neuronal Dysfunction
Mental health disorders, including depression, anxiety, and schizophrenia, often have deep roots in neuronal dysfunction. Emerging research establishes a connection between the health of neurons and the regulation of emotions and behaviors. Chemical imbalances, altered neurotransmission, and changes in neural circuitry have been identified as potential culprits.
For example, depression has been linked to lower levels of serotonin and norepinephrine, essential neurotransmitters responsible for mood regulation. Understanding these links not only contributes to better treatment plans but also encourages a compassionate approach toward those struggling with mental health issues. Education on how neuronal health influences mental well-being is vital, especially in destigmatizing these common disorders.
- Key Points About Mental Health and Neuronal Dysfunction:
- Neurotransmitter imbalances affect mood and thought patterns
- Early intervention can prevent worsening symptoms
- Collaborative care involving psychological and medical professionals is beneficial
In summary, neuronal disorders encompass a range of conditions that emphasize the fragility of the nervous system. As research continues to evolve, it shines a light on the necessity for holistic approaches to treatment and understanding, as well as further investigation into these intricate topics.
Future Directions in Neuronal Research
Researching the function of nerve cells is pressing, especially as our understanding of the human brain becomes ever more intricate. Future directions in neuronal research hold the promise of technological breakthroughs, innovative treatment methodologies, and ethical considerations that greatly impact the realm of neuroscience. The insights gained from ongoing studies not only illuminate our understanding of nerve cell functions but also pave the way for potential therapies that address both neurological and mental health issues.
Technological Advances in Neuroscience
As technology leaps ahead, its applications in neuroscience are compelling. Tools such as functional magnetic resonance imaging (fMRI) and optogenetics drastically transform how researchers observe neuronal activity in real-time.
- fMRI allows scientists to visualize brain activity while patients perform tasks, honing in on specific neural circuits involved in various cognitive functions.
- Optogenetics takes it a step further by enabling researchers to control neurons with light, providing a clearer picture of how neural pathways interact.
The fusion of these technologies promises more precise diagnostic tools as well as tailored therapeutic strategies that can be adjusted to fit the unique neural architecture of each patient. Together, they bear the potential for discoveries that could one day lead to reversing damage caused by neurodegenerative diseases, as well as more nuanced treatments for conditions such as depression and anxiety disorders.
Innovative Treatment Approaches
With the mapping of neuronal connections, the foundation for innovative treatments becomes clear. For instance, the advent of stem cell therapy holds immense promise. By employing stem cells to regenerate damaged neuronal tissue, there's a possibility of restoring functionality in spinal cord injuries or Alzheimer's disease patients. Additionally, developments in gene therapy may allow for corrective interventions at the genetic level, altering neuronal processes to mitigate conditions like Huntington's disease.
Psychopharmacology is also on the move with a focus on developing medications that target specific neurotransmitter pathways or receptors, enhancing specificity and reducing side effects. Scientists have been exploring personalized medicine—tailoring treatments based on individual genetic markers, thus improving efficacy and patient outcomes.
Ethical Considerations in Neuroresearch
While the future glows bright with possibilities, ethical considerations are a must in neuroscientific exploration. As we advance towards manipulating neuronal connections and possibly even consciousness, we face questions of autonomy, informed consent, and the essence of identity. The boundaries between therapy and enhancement blur—when is it acceptable to modify cognitive functions or memory? This issue requires careful deliberation.
There are also concerns regarding the potential misuse of technology. For example, techniques that gather data on brain structure and function could be susceptible to privacy violations. Formulating ethical guidelines is crucial to ensure that advancements in neuronal research do not outpace our moral compass.
In summary, the future directions in neuronal research underscore the importance of integrating technological advances, exploring innovative treatment approaches, and safeguarding ethical standards. It truly is a dynamic field, holding potential that could reshape our understanding and treatment of the human brain, thus bringing us one step closer to enhanced well-being and prosperity.