Understanding the Production of Polypeptides in Biology
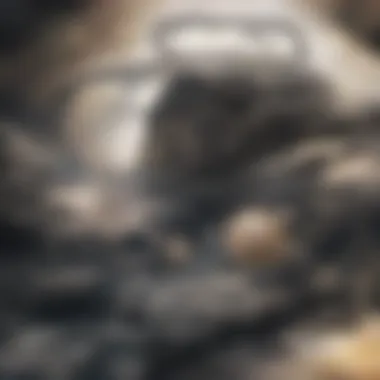
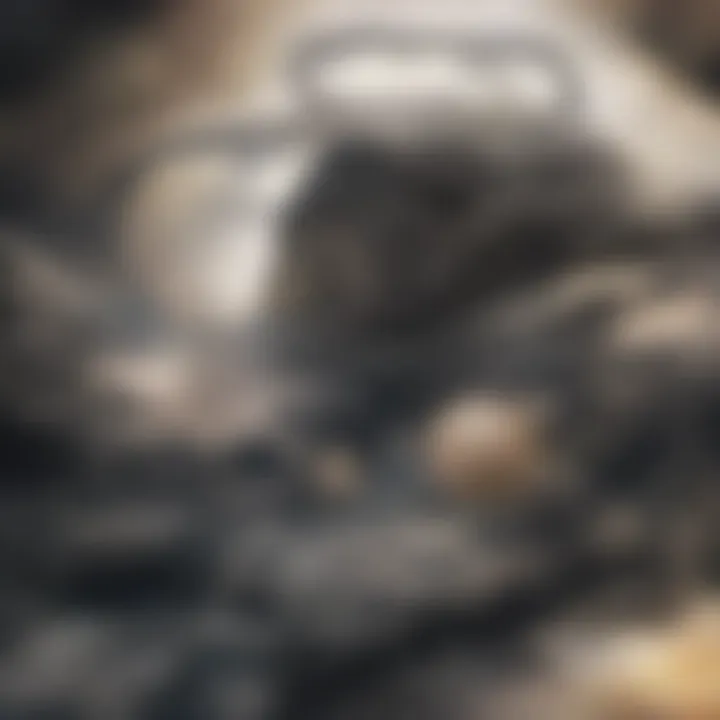
Article Overview
Purpose of the Article
The primary objective of this article is to dissect the multifaceted process of polypeptide production. This intricate process is fundamental to biological systems, and understanding it is pivotal for researchers and professionals alike. By focusing on mechanisms of synthesis, the roles of various molecular entities in translation, and the biochemical pathways of polypeptide formation, the article aims to create a comprehensive resource for students, educators, and industry specialists. Readers can expect a detailed examination that goes beyond mere surface-level descriptions, diving into the nuances that define polypeptide synthesis.
Relevance to Multiple Disciplines
Polypeptides are crucial in several scientific disciplines, including biology, biochemistry, and molecular genetics. Their role extends into medicine and biotechnology, influencing drug development and therapeutic interventions. As a result, understanding polypeptide production is relevant not only for basic science but also for applied research and clinical applications. By bridging these disciplines, this article provides a valuable perspective that benefits a variety of scholarly and practical pursuits.
Research Background
Historical Context
The exploration of polypeptides dates back to the early 20th century when scientists first began to understand the genetic code and its implications for protein synthesis. Notable figures like Frederick Sanger, who pioneered protein sequencing, and James Watson and Francis Crick, who unraveled the structure of DNA, laid the groundwork for modern molecular biology. This historical context sets the stage for comprehending how proteomics has evolved into a critical field within biological sciences today.
Key Concepts and Definitions
To foster a clearer understanding, it is essential to define some key concepts:
- Polypeptides: Chains of amino acids linked by peptide bonds, forming the building blocks of proteins.
- Synthesis Mechanisms: The processes through which polypeptides are produced, including transcription and translation.
- Translation: The phase in protein synthesis where the decoded information from messenger RNA leads to the formation of polypeptides.
- Biochemical Pathways: Networks of reactions through which cellular processes involving polypeptide synthesis and modifications occur.
Understanding these terms will facilitate a more nuanced appreciation of the upcoming sections which delve deeper into each aspect of polypeptide production.
Prelims to Polypeptides
Understanding polypeptides is essential for several scientific fields. These compounds play critical roles in how biological functions occur. A polypeptide consists of a linear chain of amino acids linked by peptide bonds. The sequence and the structure of these chains dictate the polypeptide's function within an organism. This article explores the synthesis and significance of polypeptides, detailing their role in cellular processes and how they underpin life's complexity.
Definition and Importance
Polypeptides are polymers made up of amino acids, which are the fundamental units of proteins. Each polypeptide can consist of varying numbers of amino acids, often ranging from just a few to several thousand. Their importance cannot be overstated; they influence cellular structure and enzymatic functions, and they often act as hormones or signaling molecules.
A few key points about polypeptides include:
- Diversity: Different arrangements of amino acids result in polypeptides with distinct functions.
- Cellular Functions: They participate in virtually every cellular process.
- Translation by Ribosomes: The synthesis of polypeptides occurs through intricate mechanisms that involve ribosomes and messenger RNA.
- Medical Applications: Understanding polypeptides can help in developing therapies for diseases, highlighting the intersection of biology and medical science.
In recent years, the exploration of polypeptides has provided insights into biochemical pathways. It has also led to advancements in drug development, vaccine design, and biotechnology.
Historical Context of Polypeptide Research
The study of polypeptides has a rich history in science. Early investigations into proteins laid the groundwork for understanding polypeptides. In the early 19th century, scientists like Frederick Hofmann began characterizing proteins and, eventually, polypeptides. The development of techniques such as chromatography allowed for the isolation and study of individual amino acids.
Later in the 20th century, significant discoveries like the elucidation of the DNA structure by James Watson and Francis Crick paved the way for more sophisticated studies. These advancements helped frame polypeptide synthesis in the context of genetic coding. As techniques improved, researchers began to appreciate not just how polypeptides were organized but also how they functioned within cellular environments.
The evolution of molecular biology has fostered an increasing understanding of gene expression and the translation processes. This historical context emphasizes the ongoing relevance of polypeptide research, shaping many areas, including agriculture and pharmaceuticals.
"Polypeptides constitute not merely the basic building blocks of life but also the key to understanding biological complexity."
"Polypeptides constitute not merely the basic building blocks of life but also the key to understanding biological complexity."
In summary, polypeptides form a critical component of biological sciences. Their basic structure and complex functional roles provide valuable insights for researchers and professionals alike. Understanding their history and significance is crucial for anyone interested in the intricacies of life at the molecular level.
Basic Biology of Polypeptides
The basic biology of polypeptides forms a fundamental part of our understanding of life on a molecular level. Polypeptides are polymers composed of amino acids, linked together through peptide bonds. The arrangement and sequence of these amino acids define the polypeptide's properties and functionality within biological systems. This section explores their structure and the vital role of amino acids, offering insight into the processes that govern their synthesis and function.
Structure of Polypeptides
Polypeptides are characterized by their unique structure, which is primarily determined by the sequence of amino acids. Each polypeptide can adopt different conformation based on environmental factors. There are four key levels of structure:
- Primary Structure: The linear sequence of amino acids that compose the polypeptide.
- Secondary Structure: Localized folding due to hydrogen bonding, resulting in structures like alpha-helices and beta-sheets.
- Tertiary Structure: The overall three-dimensional shape formed by the entire polypeptide's configuration, influenced by interactions among R-groups.
- Quaternary Structure: This structure is relevant for proteins composed of multiple polypeptides, dictating how these subunits interact.
Understanding the structure of polypeptides is crucial, as it affects not only their stability but also their functionality in biological processes. Changes in even a single amino acid can lead to significant functional repercussions, highlighting their importance in areas such as biochemistry and medicine.
Amino Acids: Building Blocks
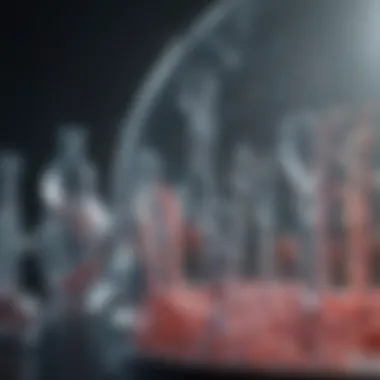
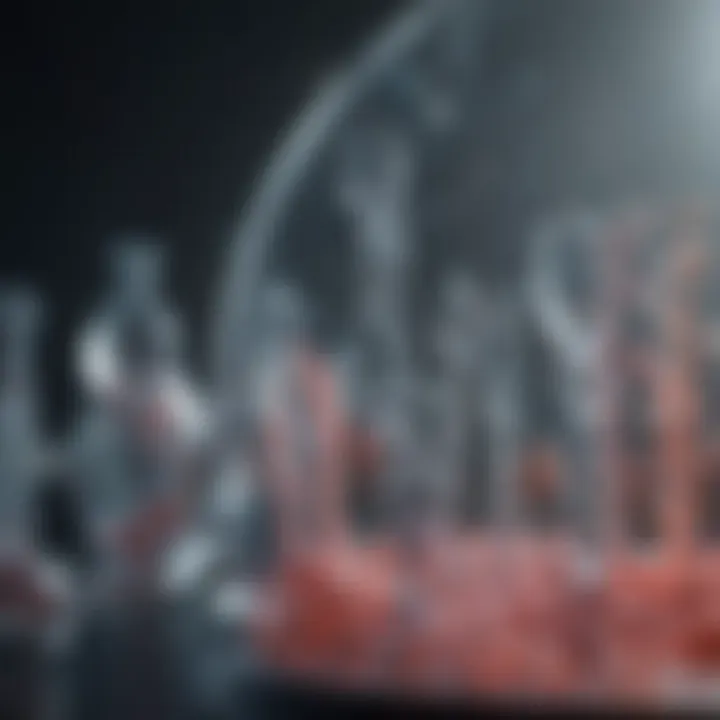
Amino acids serve as the essential building blocks of polypeptides. There are twenty standard amino acids, each varying in its side chain (or R-group), which contributes to the diversity of polypeptide forms.
Amino acids can be categorized into:
- Essential Amino Acids: These are not synthesized by the body and must be obtained through the diet. Examples include leucine, lysine, and valine.
- Non-Essential Amino Acids: The body can synthesize these amino acids. Examples include alanine, asparagine, and glutamic acid.
The sequence in which amino acids are linked together is critical. It influences how a polypeptide will fold and, consequently, its biological activity. The arrangement of polar, non-polar, acidic, or basic amino acids affects the molecule's interactions with its environment, playing a decisive role in its overall function in metabolic pathways and cellular events.
Understanding these concepts lays the groundwork for grasping more complex mechanisms, such as transcription and translation. As we explore the various processes of polypeptide synthesis further in this article, it becomes apparent how fundamental these biological principles are in the broader context of molecular biology.
Mechanisms of Polypeptide Synthesis
The production of polypeptides is a fundamental biological process with far-reaching implications in various fields, including medicine and biotechnology. Understanding the mechanisms involved in polypeptide synthesis is crucial. It grants insight into how proteins, which play key roles in cellular functions, are formed. The process involves multiple steps, with transcription and translation being the two primary phases. This section emphasizes the intricate nature of these mechanisms and the essential players involved, providing a clear framework for comprehending protein synthesis.
Transcription Process
Transcription is the initial step in the production of polypeptides. It occurs in the nucleus of eukaryotic cells. During transcription, a specific segment of DNA is transcribed into messenger RNA (mRNA). The enzyme RNA polymerase binds to the promoter region of a gene. This binding initiates the unwinding of the DNA strands, allowing the RNA polymerase to synthesize a single strand of mRNA by adding complementary ribonucleotides.
This process ensures that the genetic code is accurately copied from DNA to mRNA. The newly formed mRNA strand undergoes several modifications, including the addition of a 5′ cap and a poly-A tail, which help protect the mRNA from degradation and facilitate its export from the nucleus to the cytoplasm. The importance of transcription cannot be understated, as it serves as the first regulatory checkpoint in the overall process of gene expression.
Translation Mechanism
Translation is the subsequent step where the mRNA is decoded to synthesize polypeptides. It occurs in the cytoplasm and involves ribosomes, transfer RNA (tRNA), and various enzymatic factors. The process begins when a ribosome attaches to the mRNA molecule. The sequence of codons on the mRNA dictates the order of amino acids that will be assembled into a polypeptide chain.
tRNA plays a crucial role in this stage. Each tRNA molecule carries a specific amino acid and has an anticodon that is complementary to the corresponding codon on the mRNA. As the ribosome moves along the mRNA, tRNA molecules sequentially bring the appropriate amino acids to the ribosome. The ribosome catalyzes the formation of peptide bonds between adjacent amino acids, leading to the elongation of the polypeptide chain. This translation process is vital for cellular function, as it determines the protein produced based on the mRNA sequence.
Role of Ribosomes
Ribosomes are often described as the machinery of protein synthesis. They exist as large and small subunits, which come together during translation. Located in the cytoplasm, ribosomes can either be free-floating or associated with the rough endoplasmic reticulum. Their structure allows them to provide a site for mRNA and tRNA interaction, facilitating efficient translation.
Ribosomes have three important sites: the A (aminoacyl) site, the P (peptidyl) site, and the E (exit) site. The incoming tRNA carrying an amino acid binds at the A site, while the growing polypeptide chain is held at the P site. The E site acts as a exit point for discharged tRNA molecules. The ribosome's movement along the mRNA transcript ensures the synthesis of polypeptides based on the codon sequence, highlighting the ribosome's integral function in translation.
"Ribosomes are the heart of protein synthesis, meticulously orchestrating the translation of genetic information into functional proteins."
"Ribosomes are the heart of protein synthesis, meticulously orchestrating the translation of genetic information into functional proteins."
In summary, understanding the mechanisms of polypeptide synthesis is critical for a comprehensive knowledge of biological processes. Transcription and translation are essential for transforming genetic information into functional proteins, each step contributing to the overall efficiency and accuracy of protein production.
Key Players in Polypeptide Production
In the intricate landscape of polypeptide production, several key elements interact seamlessly to ensure successful synthesis. This section delves into the vital roles of each of these elements in the biosynthesis of polypeptides, fostering a deeper understanding of their importance in both physiological and experimental contexts.
mRNA: The Blueprint
Messenger RNA, or mRNA, serves as the fundamental blueprint in the synthesis of polypeptides. It is synthesized during the transcription process, where DNA is transcribed into a complementary mRNA strand. This mRNA contains codons, which are sequences of three nucleotides that correspond to specific amino acids.
The significance of mRNA lies in its specificity and accuracy. It carries the genetic instructions from the DNA in the nucleus to the ribosomes, where translation occurs. In essence, when the mRNA reaches the ribosome, it dictates the sequence of amino acids that need to be assembled to form a polypeptide.
Each mRNA strand is unique, allowing for the expression of different proteins suited for various functions in the cell.
Each mRNA strand is unique, allowing for the expression of different proteins suited for various functions in the cell.
tRNA: The Adapter Molecule
Transfer RNA, or tRNA, is essential in translating the genetic code from mRNA into a polypeptide. Each tRNA molecule carries a specific amino acid and has an anticodon that is complementary to the mRNA codon. This relationship ensures that the correct amino acid is added to the growing polypeptide chain during synthesis.
The role of tRNA highlights precision.
- Recognition: tRNA recognizes the corresponding codon on the mRNA.
- Transfer: It transfers its attached amino acid to the polypeptide chain.
- Regulation: The availability of specific tRNA can influence the efficiency of polypeptide synthesis, thus affecting gene expression.
Enzymatic Factors
In polypeptide production, enzymatic factors are indispensable. They catalyze various reactions necessary for the synthesis and modification of polypeptides. Key enzymatic factors include:
- Aminoacyl-tRNA Synthetases: These enzymes attach amino acids to their corresponding tRNA, forming aminoacyl-tRNA, which is crucial for translating the genetic code.
- Peptidyl Transferase: A component of the ribosome that forms peptide bonds between adjacent amino acids, facilitating the elongation of the polypeptide chain.
- Chaperones: Proteins that assist in proper folding and assembly of newly synthesized polypeptides, preventing misfolding or aggregation.
Each of these enzymatic factors plays a distinct role in controlling the efficiency and fidelity of polypeptide synthesis. Understanding their functions not only sheds light on the basic biological processes but also opens avenues for biotechnological innovations.
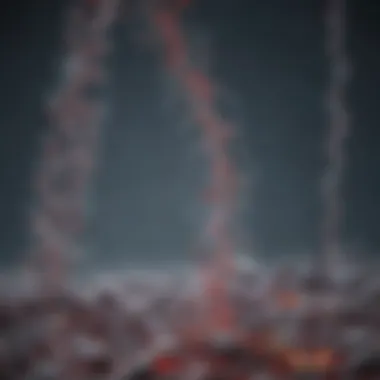
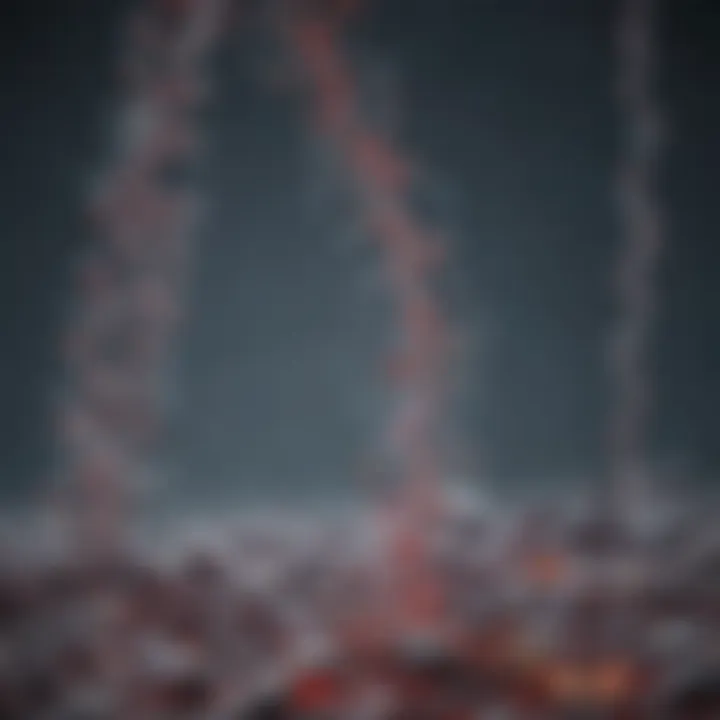
The Role of the Endoplasmic Reticulum
The endoplasmic reticulum (ER) serves a crucial role in the production of polypeptides, acting as a primary site for protein synthesis, folding, and modification. Understanding its functions is vital for grasping how cells build and mature proteins. There are two distinct forms of the ER: rough and smooth, each with specific responsibilities that interlink with the overall process of polypeptide production.
Rough vs Smooth ER
The rough endoplasmic reticulum (RER) is characterized by the presence of ribosomes on its cytoplasmic surface. This gives it a bumpy appearance under a microscope. Ribosomes synthesize proteins directly into the RER lumen. Once a polypeptide enters the RER, it may begin to undergo folding and post-translational modifications. These modifications are vital for ensuring the polypeptide is functional and stable. The RER thus plays an essential role in producing proteins that are either secreted from the cell or embedded in cellular membranes.
On the other hand, the smooth endoplasmic reticulum (SER) lacks ribosomes and is involved primarily in lipid synthesis and detoxification processes. Although it does not directly contribute to polypeptide synthesis, the SER supports overall cell function by providing essential lipids needed for membrane formation and other cellular processes. Furthermore, it is responsible for the metabolism of carbohydrates and the detoxification of drugs and poisons, which can influence the cell's capacity to produce and maintain proteins.
The rough endoplasmic reticulum is integral to the initial stages of polypeptide processing, while the smooth ER complements these functions by maintaining cellular homeostasis.
The rough endoplasmic reticulum is integral to the initial stages of polypeptide processing, while the smooth ER complements these functions by maintaining cellular homeostasis.
Protein Folding and Modification
Once polypeptides are synthesized and enter the lumen of the rough ER, they undergo a series of folding and modification processes. Proper folding is critical; misfolded proteins can lead to cellular dysfunction or diseases. Within the ER, molecular chaperones assist in the correct folding of the polypeptides. These chaperones guide the polypeptides, helping them attain their final three-dimensional structures.
Moreover, post-translational modifications are key steps that happen in the ER. These can include the addition of carbohydrate groups, a process known as glycosylation. This modification aids in protein stability, sorting, and recognition by other molecules within the cell. Additionally, disulfide bonds are often formed in the ER, helping to stabilize the protein structure in preparation for its final functions.
Quality Control in Polypeptide Production
Quality control in polypeptide production is crucial for ensuring the proper function and effectiveness of proteins within biological systems. Given that polypeptides can influence numerous cellular processes, the steps taken to maintain quality during their synthesis directly affect their functionality. When polypeptides fold incorrectly, they may become non-functional, leading to cellular malfunction and various diseases. Therefore, rigorous quality control mechanisms are essential in the production phase.
The quality control processes involve monitoring and adjusting factors that ensure polypeptides maintain proper structures. This encompasses everything from the synthesis stage through to post-translational modifications. Efficient quality control not only improves the reliability of polypeptides but also enhances the overall yield of proteins that are required in various applications, including therapeutic settings.
Chaperones and Their Functions
Chaperones are a class of proteins that assist in the proper folding of newly synthesized polypeptides. They are essential in preventing misfolding and aggregation of proteins that can occur during the synthesis process. Heat shock proteins are one well-studied group of chaperones, which become especially active under stress conditions.
These molecular chaperones work by binding to exposed hydrophobic regions of nascent polypeptides, stabilizing them in an unfolded state until they are ready to fold correctly. By preventing premature folding, chaperones help minimize the risk of errors. Their role is vital in environments where rapid protein production is necessary, such as in recombinant DNA technology.
Some common types of chaperones include Hsp70, Hsp60, and Thioredoxin. Each serves specific functions, thereby contributing to the overall fidelity of protein production. The action of chaperones exemplifies one of the many layers of quality control in polypeptide production.
Proteasomes: Degradation Mechanisms
The proteasome is a crucial complex involved in regulating the concentration of specific proteins by degrading misfolded or unnecessary polypeptides. This mechanism is fundamental to maintaining cellular homeostasis. By selectively removing these unwanted proteins, proteasomes play a significant role in ensuring that cellular machinery remains efficient.
Proteasomal degradation is not random; it typically follows the tagging of target proteins with ubiquitin molecules, a process known as ubiquitination. Once marked, the polypeptides are directed to the proteasome for degradation. This process allows cells to recycle amino acids and regulate protein levels, which is essential for normal cellular functions.
In summary, the interplay between chaperones and proteasomes exemplifies the multifaceted quality control mechanisms in polypeptide production. These systems work synergistically to ensure that only properly folded and functional proteins are available for cellular processes. The importance of rigorous quality control cannot be overstated as it directly influences both cellular health and the development of therapeutic interventions.
Applications of Polypeptide Research
The examination of polypeptide research has substantial implications across various fields. Understanding how polypeptides function and their structures can lead to major advancements in medical and biotechnological applications. These applications not only highlight the importance of polypeptides in human health and diseases, but also showcase the potential for innovation in different industries.
Medical and Therapeutic Uses
Polypeptides play a critical role in medicine, particularly in the development of therapeutics. One significant aspect is the creation of peptide-based drugs. These drugs can target specific receptors and enzymes, providing better efficacy and reduced side effects compared to traditional small molecule drugs.
For instance, insulin is a polypeptide hormone that regulates blood sugar levels. Synthetic versions of insulin have improved diabetes management, illustrating the transformative power of polypeptide research in treating chronic conditions. Moreover, peptide vaccines are emerging as a promising strategy in immunotherapy. By using specific antigenic peptides, these vaccines can induce targeted immune responses, offering advantages over conventional vaccines.
Some examples of peptide therapeutics in the market include:
- Exenatide: Used for diabetes management.
- Liraglutide: Another medication for diabetes, also approved for weight management.
- Somatropin: A growth hormone used in growth disorders.
In addition to these examples, polypeptides also serve as biomarkers which can help diagnose diseases earlier and monitor the effectiveness of treatments. Their research remains central to advancing precision medicine strategies.
Biotechnological Innovations
Beyond their medical uses, polypeptides are at the forefront of biotechnological innovation. The use of recombinant DNA technology has revolutionized the way we produce polypeptides. This approach allows for the manipulation of DNA to create genetically engineered organisms that can express desired polypeptides in large quantities, such as industrial enzymes or bioactive compounds.
In industrial applications, polypeptides serve vital functions in:
- Biocatalysis: Enzymes derived from polypeptides are used to enhance reaction efficiency in various chemical processes.
- Food Industry: Polypeptides are used as flavor enhancers or preservatives.
- Agriculture: Peptide-based products can act as biopesticides, promoting sustainable practices.
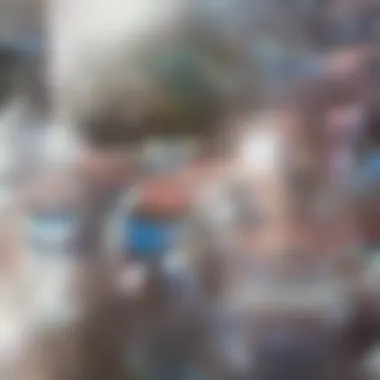
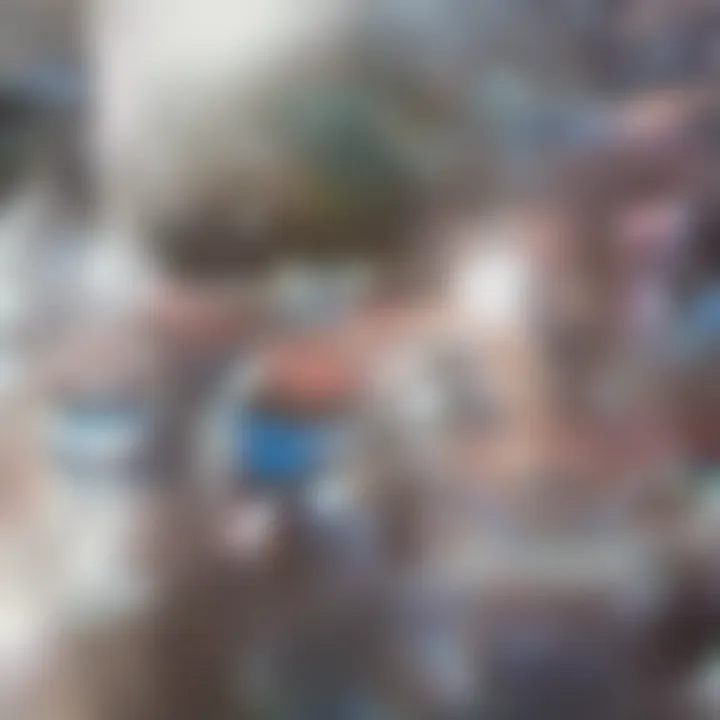
Additionally, advancements in synthetic biology have opened new doors for designing custom polypeptides with specific functions. These innovations allow for better drug delivery systems and materials science applications.
The exploration of polypeptides in biotechnology not only enhances existing products but also paves the way for novel applications, reshaping various industries.
The exploration of polypeptides in biotechnology not only enhances existing products but also paves the way for novel applications, reshaping various industries.
Challenges in Polypeptide Production
The production of polypeptides, while fundamental to biology, presents multiple challenges that must be addressed for successful synthesis and application in various fields. These challenges range from inherent inefficiencies in the synthesis processes to complications stemming from post-translational modifications. Recognizing and overcoming these obstacles is critical for advancing research and practical uses in medicine and biotechnology.
Inefficiencies in Synthesis
Polypeptide synthesis is often riddled with inefficiencies. One significant factor is the complexity involved in accurately producing the desired sequence of amino acids. Even slight errors during transcription or translation can result in dysfunctional polypeptides, which may not achieve the intended biological activity. The processes of transcription and translation rely on precise mechanisms, yet mistakes can occur due to various reasons such as mutations in DNA or inaccuracies in the ribosomal machinery.
Some common inefficiencies include:
- Yield Variability: The amount of polypeptide produced can differ widely among batches, causing inconsistencies in research and applications.
- Time Consumption: The lengthy duration required for synthesis can delay experiments and product development.
- Resource Allocation: Significant amounts of energy and materials may be wasted during large-scale production, which is costly and unsustainable.
Addressing these inefficiencies requires ongoing research into optimization techniques and the development of more reliable synthesis protocols. This could potentially involve methods such as optimizing enzyme conditions or enhancing the fidelity of the translation process.
Post-Translational Modifications
Post-translational modifications (PTMs) add another layer of complication in polypeptide production. After a polypeptide is synthesized, it often undergoes various modifications that are vital for its functionality. These modifications can include glycosylation, phosphorylation, and methylation.
The significance of post-translational modifications cannot be overstated. For example, glycosylation is crucial for cell signaling and protein stability. A polypeptide without the correct modifications may fail to perform its designated role in a biological context.
The challenges posed by PTMs include:
- Complexity of Modifications: The pathways for these modifications are multifaceted and vary between cell types. This variability can introduce challenges when trying to replicate specific modifications in vitro.
- Quality Control: Ensuring that polypeptides have undergone the correct modifications requires sophisticated quality control methods, which can be resource-intensive and time-consuming.
- Therapeutic Implications: In therapeutic applications, the presence or absence of PTMs can significantly impact a drug's efficacy and safety. Variations in production methods can lead to products that are not therapeutically viable.
Future Directions in Polypeptide Studies
The study of polypeptides is evolving rapidly, and current advancements are opening new pathways for research and application. As the world of molecular biology unfolds, it is crucial to identify and understand the future directions that promise to reshape our approaches to polypeptide production. This section elaborates on emerging technologies and biotechnological innovations that may vastly increase our capabilities in manipulating and utilizing polypeptides. These directions not only hold potential benefits for various scientific fields but also bring along considerations that must be addressed.
Emerging Technologies in Gene Editing
Gene editing technologies are revolutionizing biological research, particularly in the synthesis and manipulation of polypeptides. Techniques such as CRISPR-Cas9 are allowing for precise edits in genetic sequences, which can improve the efficiency of polypeptide production. These advancements enable researchers to directly modify the DNA responsible for peptide synthesis, resulting in tailored polypeptides with specific properties.
- Precision Editing: With tools like CRISPR, scientists can alter amino acid sequences, which directly impact polypeptide structures and functions.
- Efficient Production: Gene editing can reduce the time and resources needed for generating proteins, addressing some of the inefficiencies currently faced in polypeptide synthesis.
- Customization: The ability to create specific modifications can lead to polypeptides that perform better in therapeutic or industrial applications.
Despite these advantages, ethical considerations around gene editing must be taken seriously. Responsible research and guidelines will be essential to avoid misuse. Also, the long-term effects of genetic alterations are still not fully understood, and this uncertainty calls for cautious exploration.
Synthetic Biology Applications
Synthetic biology represents another frontier where the manipulation of polypeptides plays a critical role. By combining biology and engineering, synthetic biology aims to design and construct new biological parts or systems. Polypeptides can be synthesized to create novel proteins with enhanced functionality.
Several applications of synthetic biology concerning polypeptides include:
- Biopharmaceuticals: Customized polypeptides can lead to new drug therapies that are more effective and specific for diseases.
- Environmentally Friendly Processes: Engineered polypeptides can be used in sustainable manufacturing processes, reducing the need for harsh chemicals.
- Biosensors: Innovative designs can lead to the development of biosensors that detect environmental pollutants or pathogens based on specific polypeptide interactions.
Through these applications, synthetic biology holds the promise of revolutionizing how we use polypeptides for benefit of human health and the environment. However, the field must continuously navigate the ethical implications of synthetic organisms, ensuring that developments do not pose unforeseen risks.
These advancements represent a significant promise for the future of polypeptide research, yet ongoing ethical scrutiny is essential.
These advancements represent a significant promise for the future of polypeptide research, yet ongoing ethical scrutiny is essential.
Closure
The conclusion of this article serves as a critical synthesis of the intricate processes involved in polypeptide production. This topic is crucial in understanding various biological mechanisms that underpin cellular functions. The production of polypeptides, driven by a series of complex reactions and interactions, supports essential activities in all living organisms. A comprehensive grasp of this subject can lead to advances in medicine, agriculture, and biotechnology.
Summary of Findings
The exploration regarding polypeptide production provides insight into multiple areas:
- Synthesis mechanisms: The article traces the pathways leading from DNA transcription to mRNA translation, emphasizing the role of ribosomes in assembling polypeptides.
- Key players: The functions of mRNA, tRNA, and various enzymes were highlighted, detailing how they cooperate to produce functional proteins.
- Quality control: The mechanisms ensuring the correct folding and modification of proteins were discussed, showcasing the role of chaperones and proteasomes.
- Applications and challenges: Attention was brought to the significance of polypeptides in medicine and biotechnology, along with the challenges related to synthesis efficiency and post-translational modifications.
This summary encapsulates key components that contribute to the understanding of polypeptide production and highlights the integration of scientific knowledge across disciplines.
Implications for Future Research
Future research in polypeptide production can have important implications in several fields:
- Emerging technologies: Innovations in gene editing, such as CRISPR, can refine the production processes, making them more efficient. This is likely to pave the way for customized proteins that humans can benefit from, especially in therapeutic contexts.
- Synthetic biology: The development of synthetic biology techniques enables the design of novel polypeptides with specific functions. This offers vast possibilities for medical therapies and industrial applications.
- Understanding diseases: Research could focus on the implications of improperly folded or misallocated polypeptides in diseases, particularly neurodegenerative disorders. By addressing these issues, scientists may find new treatment avenues.