Exploring Advanced Techniques in Wave Measurement
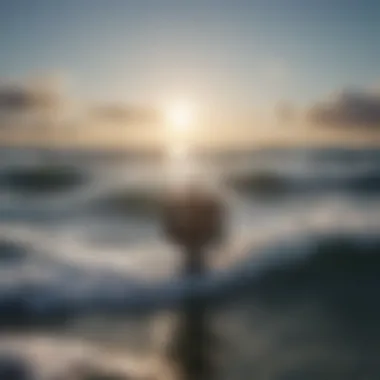
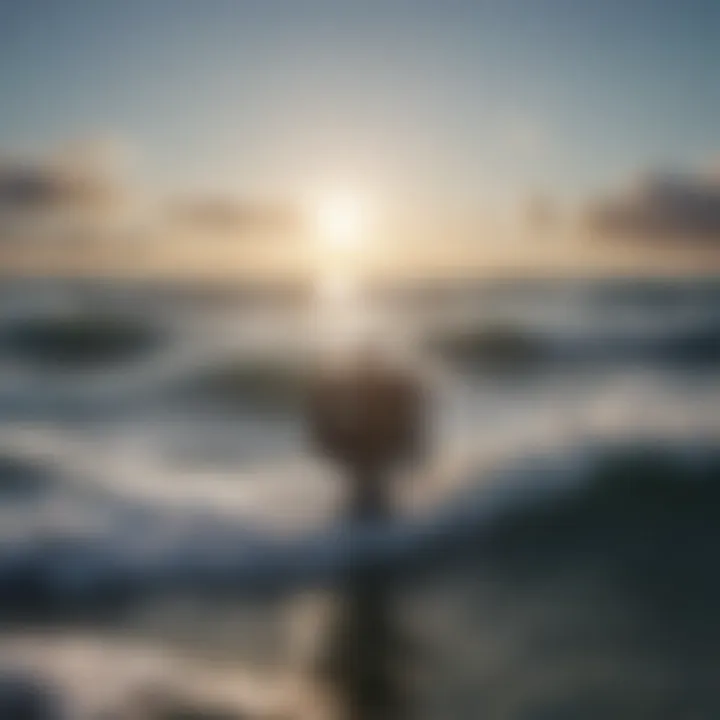
Article Overview
Purpose of the Article
In the world of scientific inquiry, understanding wave dynamics has become increasingly vital. This article concentrates on the various advanced techniques utilized for measuring these waves, as well as their significance in both theoretical and practical applications. From oceanography to engineering, the ability to accurately measure wave patterns and heights can profoundly influence decision-making and research validation. By diving into the methodologies and technologies that underpin wave measurement, this piece aims to equip students, researchers, educators, and professionals with a nuanced comprehension of the discipline.
Relevance to Multiple Disciplines
Wave measurement techniques hold significance across diverse fields, demonstrating their versatility and applicability. Some of these include:
- Environmental Science: Understanding wave behavior aids in predicting coastal erosion and sediment transport.
- Engineering: In marine construction, accurate wave data is crucial for designing resilient structures.
- Meteorology: Waves can influence weather patterns, making their measurement essential for weather forecasting.
By bringing together insights from these various domains, the article synthesizes how advanced methodologies of wave measurement impact numerous scientific and practical applications.
Research Background
Historical Context
The history of wave measurement can be traced back to ancient civilizations that relied on simple tools for navigation and understanding sea conditions. However, the modern era saw pivotal advancements in the 20th century with the invention of sophisticated equipment like radar and acoustic sensors. These innovations allowed for real-time data gathering and significantly improved the accuracy of wave measures. As a result, contemporary research has opened new avenues for understanding fluid dynamics in various environments.
Key Concepts and Definitions
Understanding the basic concepts relevant to wave measurement ensures a solid foundation for delving deeper into specific techniques. Here are some key terms to grasp:
- Wave Height: The distance from the trough to the crest of a wave, an important parameter for assessing wave energy.
- Wave Period: The time it takes for two successive wave crests to pass a fixed point, influencing the behavior of waves in different environments.
- Spectral Analysis: A method used to break down wave data into frequency components, helping in the detailed understanding of wave dynamics.
Each of these terms encapsulates fundamental aspects of wave behavior, laying the groundwork for advanced discussions on measurement methodologies.
"Accurate wave measurement plays a key role in predicting environmental changes and improving engineering designs."
"Accurate wave measurement plays a key role in predicting environmental changes and improving engineering designs."
As we advance through this article, we will dissect various innovative instruments and data processing techniques employed in wave dynamics, aiding in progressive understanding.
Foreword to Wave Measurement
Understanding wave measurement is not merely a scientific pursuit; it's essential for a plethora of applications, from coastal management to engineering designs. Waves shape our natural world and man-made structures—getting a grip on how to measure them accurately can mean the difference between success and failure in numerous domains. The marriage of technology with wave dynamics has given birth to advanced methodologies that push the boundaries of traditional measurement, providing enhanced precision and reliability. Here, we will delve into the layers of wave measurement techniques, focusing on both foundational elements and contemporary innovations.
Definition and Importance of Wave Measurement
Wave measurement, in essence, refers to the techniques employed to assess the characteristics of waveforms in various mediums, whether in water, air, or solid materials. It's a field that doesn’t just cater to academics; it has brass-tacks implications across several industries, including environmental science, engineering, telecommunications, and energy production.
One might ask, why is this so vital? The answer lies in the multifaceted nature of waves—they can convey energy, information, and serve as indicators of environmental conditions. For instance, coastal engineers rely on accurate wave measurements to design resilient structures that can withstand the onslaught of storms. Similarly, marine biologists depend on such data to monitor habitat conditions critical for the survival of marine species.
"Data collection might seem monotonous, but it lays the very foundation upon which scientific progress is built."
"Data collection might seem monotonous, but it lays the very foundation upon which scientific progress is built."
When we measure waves, we gauge not just their amplitude, frequency, and speed, but also how they interact with structures and ecosystems. This multifaceted understanding allows for predictive modeling, ultimately driving better management strategies and innovations.
Historical Context and Evolution
To appreciate where we stand today in wave measurement, it pays to look back at how far we've come. From ancient civilizations utilizing rudimentary tools to gauge water levels and tide movements, the quest for better measurement has been ongoing.
In the 20th century, advancements in technology spurred significant innovations. The introduction of electronic sensors in the mid-1900s marked a turning point. These devices allowed for real-time data collection, paving the way for more detailed and frequent observations. With the integration of digital communication, the accuracy of wave measurements leaped ahead, transforming how researchers analyze and interpret data.
Fast forward to now, wave measuring devices have evolved into sophisticated instruments that employ a range of methodologies—from buoy systems that autonomously gather oceanographic data, to satellite-based systems that monitor large-scale wave patterns.
In summary, as the landscape of wave measurement has expanded, so too has the necessity for precision, leading researchers and engineers to innovate continually. This historical journey illustrates a trajectory of increasing complexity and advancement that underscores the importance of this field in contemporary studies and applications.
Types of Waves in Measurement Studies
Understanding the types of waves relevant to measurement studies is pivotal for accurately interpreting data within various scientific realms. Each type of wave embodies unique characteristics and behaviors that must be comprehended to choose appropriate measurement techniques and instruments. The significance of this topic cannot be understated, as it forms the foundation on which accurate data assessment stands. This section explores three core classifications of waves—mechanical, electromagnetic, and surface waves—each with its distinctive properties and applications.
Mechanical Waves
Mechanical waves require a medium to propagate, be it solids, liquids, or gases. They are fundamentally categorized into two types: transverse and longitudinal waves. Transverse waves displace the medium in a direction perpendicular to the wave's travel direction. An example of this is a wave created when a stone is thrown into a still pond, producing ripples. Longitudinal waves, on the other hand, cause the medium to displace in the same direction as the wave travels, as observed in sound waves. This distinction is essential when measuring wave phenomena, as each type necessitates different sensing capabilities.
The measurement of mechanical waves finds applications in numerous fields, like engineering and environmental studies. By accurately gauging wave behavior, researchers can improve the design of structures such as bridges and dams to withstand the forces exerted by waves. Utilizing sophisticated instruments like strain gauges further enhances the reliability of data collection regarding mechanical waves.
Electromagnetic Waves
Electromagnetic waves, unlike mechanical waves, do not require a medium and can propagate through the vacuum of space. These waves encompass a broad spectrum, ranging from radio waves to gamma rays, with visible light nestled comfortably in between. The capacity of electromagnetic waves to travel in a vacuum makes them particularly valuable in telecommunications and remote sensing applications.
To measure electromagnetic waves, instruments like antennas and photodetectors are commonly used. The ability to analyze these waves helps researchers study cosmic phenomena, monitor climate change, and explore other key scientific inquiries. The versatility of electromagnetic waves allows for various methodologies in measurement, presenting both opportunities and challenges in data collection and interpretation.
Surface Waves

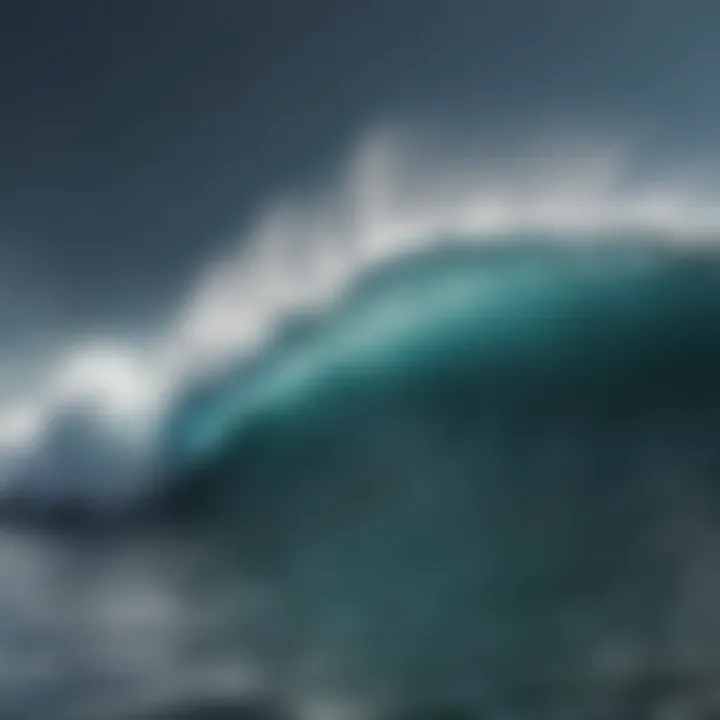
Surface waves travel along the interface between two different mediums, most notably at the boundary between air and water. These waves are critically important in oceanography and seismic studies, as they exhibit significant interactions with the medium they traverse. Surface waves, such as ocean waves or seismic waves, can reflect and refract depending on various environmental conditions, thus complicating their measurement.
In dealing with surface waves, precision in measurement techniques becomes even more crucial. Instruments such as wave buoys and radar systems play a significant role in capturing accurate data. These waves are vital for understanding coastal dynamics and their implications for engineering and ecological preservation. As such, ongoing advancements in measurement techniques cater to the nuances presented by surface waves, promising richer data for analysis.
"A thorough grasp of the types of waves in measurement studies is paramount. It not only aids in determining the appropriate instruments but also enhances the overall understanding of wave dynamics in various applications."
"A thorough grasp of the types of waves in measurement studies is paramount. It not only aids in determining the appropriate instruments but also enhances the overall understanding of wave dynamics in various applications."
The overview presented here illustrates how each type of wave serves its purpose within measurement studies. By systematically recognizing and categorizing waves, researchers can all the more effectively employ various advanced measurement methods, leading to more accurate data collection and improved outcomes across numerous scientific audiences.
Fundamental Instruments in Wave Measurement
In the realm of wave measurement, selecting the right instruments can mean the difference between precise data collection and erroneous readings. These instruments serve as the backbone of research in oceanography, meteorology, and environmental monitoring. Understanding their distinct roles not only aids in gathering accurate data but also highlights how each device contributes to a broader understanding of wave dynamics. With modern innovations continuously shaping these tools, the emphasis on effective wave measurement instruments cannot be overstated.
Wave Buoys
Wave buoys are like the watchful eyes on the ocean, bobbing up and down while capturing crucial information about wave height, period, and direction. These floating devices are often equipped with a variety of sensors and can transmit real-time data back to shore, offering invaluable insights for forecasters and researchers alike.
- Real-Time Data: Buoys ensure a constant stream of information that can be used for immediate weather forecasts and maritime safety.
- Versatility: Many wave buoys can operate in different marine environments, making them suitable for various studies from coastal regions to open ocean.
- Durability: Designed to withstand harsh oceanic environments, they maintain functionality despite adverse weather conditions.
However, the placement of these buoys is critical. They must be positioned in areas where they can adequately measure wave activity without interference from land or other obstacles.
Acoustic Doppler Devices
Acoustic Doppler devices represent an advanced approach to wave measurement, employing sound waves to gauge water current and wave heights. Unlike traditional methods, these instruments use sonar technology which allows them not just to measure surface waves but also to reach deeper waters.
It works as follows:
- Emission of Sound Waves: The device sends out sound pulses into the water.
- Reflection and Detection: Those waves bounce off particles in the water, and the device picks up the reflected signals.
- Data Analysis: By analyzing the frequency and timing of the returned signals, researchers can determine water velocity and wave characteristics.
- Depth Probing: This device helps in understanding interactions between waves and underwater currents, shedding light on complex ocean dynamics that affect coastal erosion and sediment transport.
- Precision: Acoustic measurements tend to be more accurate in turbulent conditions compared to traditional buoy readings.
The downside, however, is the potential for environmental noise to create measurement errors, requiring careful calibration and data filtering.
Pressure Sensors
Pressure sensors, often submerged at various depths, measure hydrostatic pressure changes due to wave activity. These devices can reveal a lot about wave patterns beneath the surface, allowing for deeper insights into wave dynamics that are not as readily apparent through surface measurements.
- Depth-Dependent Measurements: By analyzing pressure at different depths, researchers can infer wave energy and propagation characteristics.
- Cost-Effectiveness: Compared to other wave measurement technologies, pressure sensors can be relatively inexpensive and easy to deploy in various aquatic settings.
Nonetheless, they require meticulous calibration and sometimes extensive data processing to interpret properly. Factors such as water temperature, salinity, and atmospheric pressure can all influence readings.
Data Collection Techniques
Data collection plays a pivotal role in understanding wave dynamics, making it an essential focus of this article. Synthesizing high-quality data enables researchers, engineers, and environmentalists to make informed decisions based on actual wave behavior. With the waves being unpredictable to a degree, leveraging various data collection methods allows for a comprehensive picture of their patterns and impacts.
In wave measurement, employing the right techniques can greatly enhance the reliability of data. With each approach, certain nuances come to light, offering unique benefits and potential challenges. For instance, field measurements often yield direct, real-time observations, while remote sensing captures broader trends over extensive areas. It’s not a one-size-fits-all approach; the choice of method influences the effectiveness of data collection, and thus the outcome of research.
Field Measurements
Field measurements involve collecting data directly from the environment where the waves occur. This technique is invaluable because it allows for real-time data capture, which can be crucial in rapidly changing conditions like storms or high tides. Instruments such as wave buoys, pressure sensors, and shoreline monitors are often employed to gather precise and localized information.
Opting for field measurements provides certain advantages:
- Realism: Captures true wave behavior under natural conditions, unobstructed by artificial factors.
- Quick Feedback: Enables immediate analysis, which is beneficial in scenarios requiring urgent response to wave conditions, such as those impacting coastal communities.
- Customization: Field setups can be tailored to target specific conditions or outcomes unique to certain locations.
However, these techniques do come with their share of challenges. Environmental conditions can affect the accuracy of certain readings, and logistical factors such as the accessibility of locations can pose hurdles.
Remote Sensing Applications
Remote sensing refers to the use of satellite or aerial technologies to collect data over large areas from a distance. This method allows scientists to monitor wave behavior across vast expanses without the need for on-site instruments. Utilizing tools like lidar technology or synthetic aperture radar (SAR) can provide insights into wave height, speed, and direction.
Noteworthy benefits of remote sensing include:
- Extensive Coverage: Facilitates the monitoring of wave conditions over vast areas that might be economically or logistically impractical to measure through traditional field methods.
- Repeatability: Satellite data can be collected at regular intervals, allowing for the analysis of trends over time.
- Cost-Effective: While initial setup may be expensive, the operational costs of maintaining a satellite system can be lower compared to constantly deploying field instruments.
On the downside, remote sensing may lack the fine detail provided by localized instruments. Cloud cover or other atmospheric conditions can hinder the accuracy of data, creating gaps in information that can complicate analysis.
Modeling and Simulation Methods
Modeling and simulation are powerful data collection techniques that use mathematical formulas and computational systems to predict wave behavior. These methods can integrate data from both field measurements and remote sensing, offering a holistic view of wave dynamics in various environments.
Key advantages of modeling and simulation include:
- Predictive Power: By simulating different scenarios, researchers can forecast how waves will interact with various parameters over time.
- Scenario Testing: Enables the evaluation of 'what-if' situations, like assessing the impacts of a potential coastal development on wave patterns without having to conduct extensive physical alterations.
- Accessibility of Complex Data: Makes it easier to analyze multi-dimensional data sets that would be challenging to interpret directly from field or remote measurements.
However, it’s crucial to note that the quality of models is heavily reliant on the data used for their creation. Without inputting accurate and reliable data, the effectiveness of modeling diminishes, potentially leading to misleading conclusions.
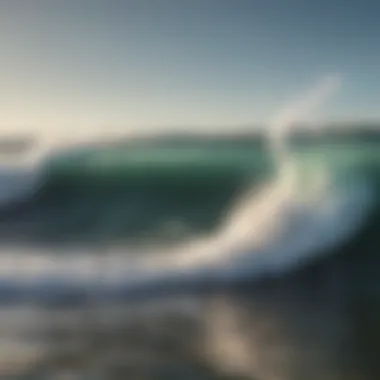
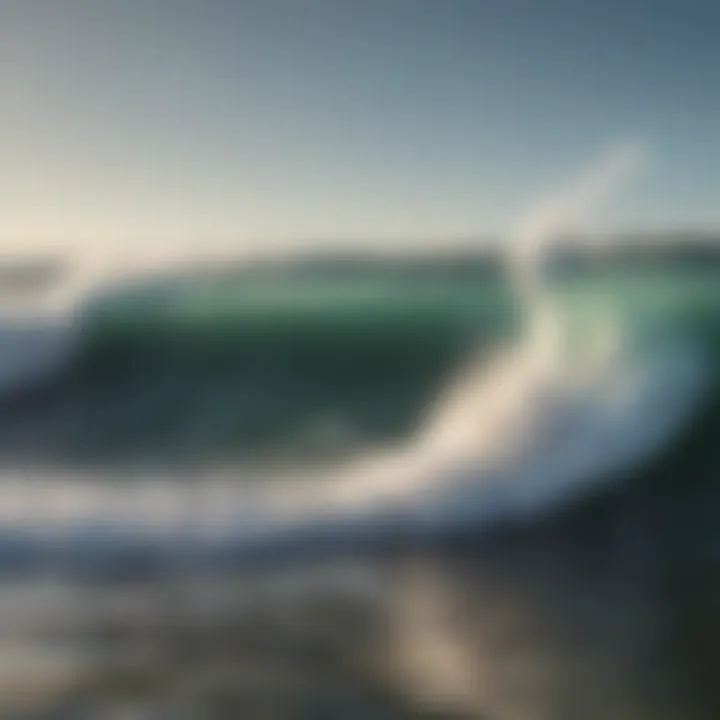
In the world of wave measurement, the blend of experiential field data, technological advancement through remote sensing, and innovative modeling strategies serves to create a robust framework for understanding the complexities of wave dynamics.
In the world of wave measurement, the blend of experiential field data, technological advancement through remote sensing, and innovative modeling strategies serves to create a robust framework for understanding the complexities of wave dynamics.
Data Processing and Analysis
In the realm of wave measurement, processing and analyzing the data collected is as vital as the measurements themselves. The effectiveness of wave measurement hinges not just on capturing data accurately, but also on interpreting and presenting it in meaningful ways. This section delves into the various facets of this critical process, underscoring its significance in drawing informed conclusions from the data gathered.
Signal Processing Techniques
Signal processing serves as the backbone of data analysis in wave measurement. Without robust processing, raw data may appear as nothing more than noise, making it challenging to extract useful insights. Techniques such as filtering, Fourier transforms, and wavelet analysis help to transform this raw data into coherent information.
Filtering methods are particularly essential. They remove unwanted frequencies, allowing researchers to isolate the relevant signals associated with wave activity. For instance, a low-pass filter can be employed to eliminate high-frequency noise from oceanographic data, focusing solely on the meaningful wave signals. This step is crucial for ensuring data integrity and clarity.
Another technique is Fourier analysis, which breaks down complex waveforms into simpler sine or cosine functions. This method assists in identifying dominant frequencies and wave patterns. Particularly in studies focusing on ocean waves, understanding frequency components can reveal critical insights, such as peak wave periods or energy distribution across different spectral bands.
"The quality of wave measurement data is largely determined by the signal processing techniques applied during analysis".
"The quality of wave measurement data is largely determined by the signal processing techniques applied during analysis".
These techniques, when applied correctly, enable researchers to distill large volumes of data into actionable intelligence, thus enhancing the quality of research outputs.
Statistical Methods in Wave Data
Statistical analysis plays a significant role in validating and interpreting wave measurement data. It involves the application of various methodologies to summarize, describe, and infer conclusions from the datasets collected. The use of descriptive statistics offers a first glance at the data, providing basic insights such as mean wave height, standard deviation, and range. Understanding these numbers lays the groundwork for deeper analysis.
Moreover, regression analysis and hypothesis testing are pivotal for exploring relationships between different wave characteristics. For instance, can be used to assess the impact of wind speed on wave height. Such analyses illuminate the dependencies within wave dynamics and can guide engineers in developing solutions to mitigate erosion or optimize coastal defenses.
In the context of wave data, researchers often employ time-series analyses. This involves examining datasets collected over time, enabling the identification of trends or patterns in wave behavior. Understanding how wave patterns change seasonally or in response to climatic events is crucial for long-term environmental and engineering assessments.
Machine Learning Applications
As technology evolves, so too do the methodologies employed in wave measurement. Machine Learning illustrates this evolution perfectly. By leveraging algorithms that can learn from data, researchers can enhance their analyses significantly. Machine learning models can predict wave behavior based on historical data, optimizing responses to emergencies like coastal flooding.
Techniques such as supervised learning, where the model is trained on labeled datasets, can forecast wave conditions under varying meteorological scenarios. For example, researchers could develop models to predict the likelihood of rogue waves by using historical wave data coupled with atmospheric conditions.
Unsupervised learning, on the other hand, finds hidden structures in datasets. This can help identify unusual wave patterns that may not be immediately evident. Using clustering algorithms, data can be grouped based on similarities, revealing insights into wave behavior that could be overlooked by traditional methods.
Furthermore, machine learning can also be utilized for classification tasks. This involves sorting wave data into categories, such as different types of wave patterns or events, based on learned features. These categorizations can aid in better understanding the behavior of waves under various conditions, contributing to more effective management strategies in coastal and oceanographic contexts.
Applications of Wave Measurement
The realm of wave measurement is not merely a laboratory undertaking; its applications stretch far and wide, touching fields like oceanography, engineering, and the environment. The importance of wave measurement lies in its role as a keystone for understanding various ecological and man-made systems. An accurate grasp of wave dynamics can influence critical decisions and innovations that range from predicting coastal conditions to the design of offshore structures. In this article, we will explore how these applications underpin significant advancements across multiple domains, addressing specific elements, benefits, and considerations.
Coastal and Oceanographic Research
Wave measurement exerts a profound impact on coastal and oceanographic research. Understanding wave behavior enables scientists to predict changes in coastal erosion, sediment transport, and marine ecosystem dynamics. For instance, when underwater topography transforms due to wave action, it affects local marine life; knowing how waves interact with submerged landscapes can assist in habitat preservation efforts.
Key Elements of Research:
- Hydrodynamics: Wave height, period, and direction contribute to hydrodynamic studies essential for modeling coastal processes.
- Ecosystem Studies: Tracking wave patterns informs researchers about nutrient flows, which can enhance productivity in marine regions.
- Climate Change Impacts: Measurements enable assessments of how global changes affect wave dynamics, creating models that predict future scenarios.
These insights help to anticipate coastal vulnerabilities, guiding efforts to fortify infrastructures against extreme weather events. The accumulation of reliable data also fosters interdisciplinary collaboration, amplifying research's societal relevance.
Engineering and Design Considerations
In the engineering domain, wave measurement is indispensable for the design and construction of marine structures such as breakwaters, piers, and wind turbines. Here, precise wave data translates into safer and more efficient engineering practices. Offshore engineering projects cover a vast array of activities, where understanding local wave climates can inform choices around materials, designs, and robust construction practices.
Critical Considerations in Engineering:
- Structural Integrity: Knowledge of wave forces aids in ensuring structural resilience against the elements.
- Cost Efficiency: Improved data collection techniques can lead to optimized designs that reduce material waste and construction costs.
- Regulatory Compliance: Adhering to safety standards is facilitated by comprehensive wave studies tailored to specific locations.
Collaboration between engineers and wave researchers creates a feedback loop; as new data emerges, engineering designs can be refined, leading to safer, smarter structures in maritime environments.
Environmental Monitoring and Management
Benefits of Wave Monitoring:
- Pollution Tracking: Waves play an essential role in the distribution of contaminants. Continuous monitoring reveals patterns that aid in pollution management.
- Habitat Restoration: Information gathered supports restoration initiatives by identifying areas with critical habitats adversely affected by wave action.
- Public Awareness: Data can also be shared with communities to raise awareness of local marine conditions and encourage responsible behavior toward ocean resources.
"Accurate wave measurement is not just a technical requirement; it serves as a bridge between sustainable practices and the health of marine ecosystems."
"Accurate wave measurement is not just a technical requirement; it serves as a bridge between sustainable practices and the health of marine ecosystems."
Challenges in Wave Measurement
Accurate wave measurement plays a crucial role in a wide array of fields, from environmental science to engineering. However, it is not without its hurdles. Understanding these challenges is essential because it helps refine measurement techniques and improve data reliability.
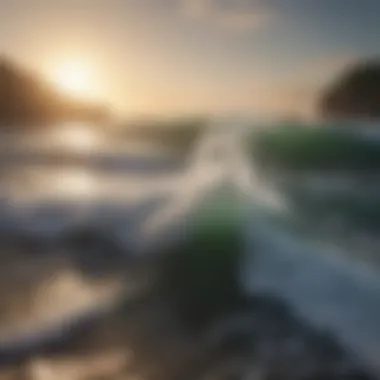
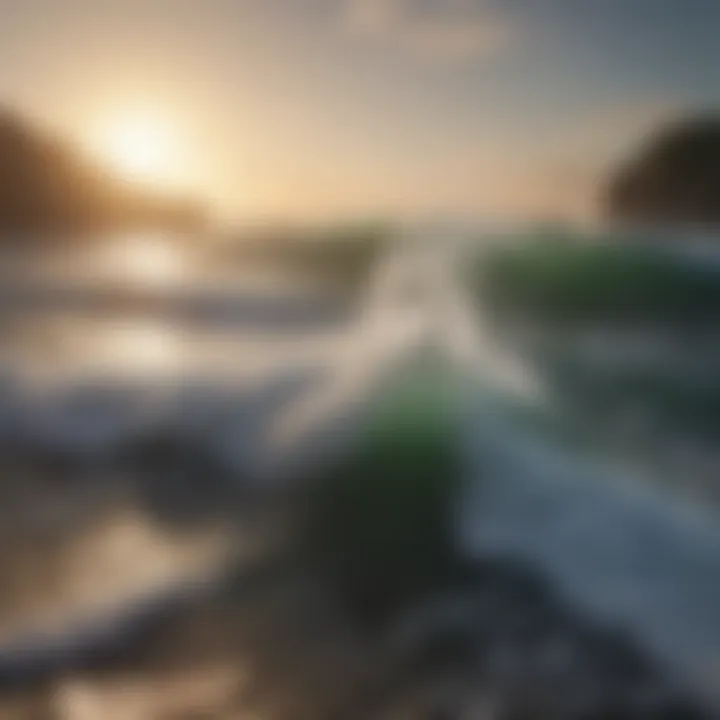
Wave measurement isn’t just about collecting data; it involves navigating numerous complexities that can affect results. Addressing these challenges is what makes achieving precision so valuable. Every step taken to mitigate issues can lead to enhanced understanding and innovative solutions.
Environmental Factors
When measuring waves, environmental factors are a significant concern. The ever-changing nature of the ocean, including waves, currents, and weather conditions, introduces variations that can skew measurements.
For instance, wind speed can drastically alter wave heights on a given day. A calm day may show waves at a certain height while a storm could double or triple those measurements. This natural variability demands constant attention from researchers to account for ephemeral influences.
Also, seasonal changes affect wave patterns, making it crucial to gather long-term data to identify trends rather than singular events. Imagine trying to gauge the average size of waves in a region without considering a hurricane season—it wouldn’t paint an accurate picture. Therefore, understanding local environmental conditions is vital for any effective wave measurement project.
Technological Limitations
Despite rapid advancements in technology, limitations still hamper the effectiveness of wave measurement tools. Not all devices can accurately gauge different types or conditions of waves. For example, while pressure sensors can measure wave heights underwater effectively, they struggle with accurately capturing surface wave dynamics during turbulence.
Another example lies within remote sensing technologies. While satellites can provide valuable data, their resolution may not always meet the demands of high-accuracy applications, particularly in coastal regions where land interference can distort readings. These technological limitations often necessitate the use of multiple instruments, increasing costs and complexity.
Therefore, researchers must not only select the appropriate tools but also remain aware of their restrictions. Understanding what each device can and cannot do is crucial for drawing valid conclusions from collected data.
Data Interpretation Difficulties
Even once accurate data is collected, interpreting that information can be fraught with challenges. The dynamics of wave behavior can create complications when trying to analyze data. Waves do not just exist in a vacuum; they interact with various factors, such as coastal features and human activities.
Further complicating analysis, there’s often a vast amount of data generated, leading to potential overload. Sorting through and making sense of extensive datasets requires sophisticated statistical methods and sometimes even machine learning techniques, both of which come with their own set of challenges. Additionally, misinterpretation can arise due to biases embedded within the models or analytical methods used.
This interpretation difficulty means that collaboration between various disciplines—like oceanographers, meteorologists, and engineers—is increasingly important. Each field brings its perspective, making it easier to develop a holistic understanding of wave dynamics.
In summary, navigating these challenges is essential for any effective wave measurement undertaking. By recognizing influences from the environment, understanding the limitations of technology, and addressing data interpretation difficulties, researchers can refine their methods and improve the reliability of their findings.
In summary, navigating these challenges is essential for any effective wave measurement undertaking. By recognizing influences from the environment, understanding the limitations of technology, and addressing data interpretation difficulties, researchers can refine their methods and improve the reliability of their findings.
Future Directions in Wave Measurement Research
The frontier of wave measurement is evolving at a rapid pace, and understanding these future directions is invaluable for advancing both scholarly pursuits and practical applications. As scientists and engineers strive for more accurate data and richer insights into wave dynamics, it’s vital to focus on how emerging technologies, interdisciplinary approaches, and sustainability considerations will shape the landscape of wave measurement research. This evolution not only enhances existing techniques but also opens doorways to novel applications that can positively impact environmental science and engineering design.
Emerging Technologies
The advent of new technologies is often the catalyst for revolutionary changes in wave measurement. Innovations such as drone-based sensors, high-resolution satellite imaging, and Internet of Things (IoT) devices are transforming how waves are analyzed. These platforms enable researchers to gather data from remote or difficult-to-access locations, providing unprecedented detail regarding wave patterns and behaviors.
- Drone Technology: Equipped with advanced sensors, drones can perform real-time monitoring of wave dynamics in coastal environments without the constraints of traditional vessels.
- Satellite Remote Sensing: The capabilities of satellites have advanced, and the resolution of data they collect can now capture minute details of wave heights and currents over vast areas.
- IoT Devices: These smart sensors can continuously communicate real-time wave data, allowing for immediate adjustments in engineering practices and environmental monitoring.
Exploring these technologies emphasizes the potential for more comprehensive and immediate data acquisition, which can significantly enhance the accuracy of models used in predictive analyses.
Interdisciplinary Approaches
Addressing the complexities inherent in wave measurement necessitates a collaborative effort across various fields. Physics, oceanography, environmental science, and engineering must come together to leverage their collective expertise. This interdisciplinary approach fosters a more holistic understanding of wave dynamics, connecting theoretical frameworks with practical applications.
- Physics and Fluid Dynamics: A solid understanding of fluid dynamics aids in refining mathematical models that predict wave behavior under various conditions.
- Environmental Science: Integrating ecological considerations into these models ensures that waves’ impacts on marine life and coastal communities are accounted for in research.
- Engineering Design: Insights from various scientific disciplines can influence the design of structures such as piers or offshore wind turbines, optimizing them against wave forces.
Through these collaborative efforts, wave measurement can evolve into a more nuanced practice, producing more applicable results for real-world challenges in both research and industry.
Sustainability Considerations
In light of increasing concerns regarding climate change and environmental disruptions, sustainability must be a key pillar in future wave measurement practices. This includes assessing the long-term impacts of measurement technologies on ecosystems and ensuring that data collection methods are environmentally friendly.
- Minimizing Ecological Footprint: Developing non-invasive measurement methods is essential to prevent disruption to marine habitats. Techniques that reduce noise pollution and limit physical intrusion will foster a more sustainable practice.
- Adaptive Management Practices: Utilizing wave measurement data for sustainable resource management can help coastal communities adapt better to changing environmental conditions, ultimately preserving their livelihoods.
- Long-term Monitoring Strategies: Establishing networks for ongoing measurement ensures that data remains relevant and can inform sustainable practices over time.
To conclude, the future of wave measurement research is fraught with the promise of innovation and the responsibility of addressing pressing societal concerns. Focusing on emerging technologies, interdisciplinary collaborations, and sustainability can enhance the significance of wave data in both scientific contexts and practical applications. As we stride into this new era, it is essential to articulate and implement practices that not only advance our understanding of waves but also protect the environments in which they manifest.
"The waves of technology keep rolling in, reshaping the shoreline of scientific inquiry.”
"The waves of technology keep rolling in, reshaping the shoreline of scientific inquiry.”
By fostering these future directions, researchers and industry professionals alike can contribute to a richer, more insightful dialogue about wave dynamics and their implications.
End
Drawing our conversation to a close, it’s essential to appreciate the multifaceted nature of wave measurement and its pivotal role in various fields. As we sift through the layers of information discussed, several key threads emerge, weaving together the narratives of technological innovation, interdisciplinary collaboration, and environmental stewardship.
Summary of Key Points
In our exploration of wave measurement techniques, we have looked closely at the fundamental components and methods that underlie this complex field. Here are some standout elements:
- Types of Waves: We’ve delineated the various categories of waves, such as mechanical, electromagnetic, and surface waves, each bringing its unique challenges and requirements.
- Instruments: The instruments ranging from wave buoys to advanced acoustic devices have been examined in detail, showcasing their significance in gathering vital data.
- Data Processing: Techniques for processing and analyzing data, including signal processing and machine learning, underline how raw measurements transform into actionable insights.
- Applications and Challenges: We’ve also touched on real-world applications, spanning coastal research and environmental studies, while acknowledging significant challenges like environmental factors and data interpretation difficulties.
The Importance of Continuous Research
Continuity in research is not simply a pursuit of knowledge; it forms the backbone for sustaining advancements in wave measurement techniques. The sea is ever-changing, influenced by climate variations, human activity, and natural events. Without ongoing studies, our understanding could quickly become outdated or even detrimental.
Investing in continuous research opens up numerous pathways. Emerging Technologies: Innovations like improved sensors can provide higher accuracy and reliability. Interdisciplinary Approaches: Collaborations among engineers, scientists, and environmentalists could lead to comprehensive solutions for water-related challenges. Sustainability Considerations: Lastly, examining how our practices in wave measurement affect the environment is crucial for long-term ecological balance.
"Continuous research is not merely an option; it's an onus we carry for future generations to ensure the planet is navigable and sustainable."
"Continuous research is not merely an option; it's an onus we carry for future generations to ensure the planet is navigable and sustainable."