Exploring the Dynamics of Electricity Generating Turbines
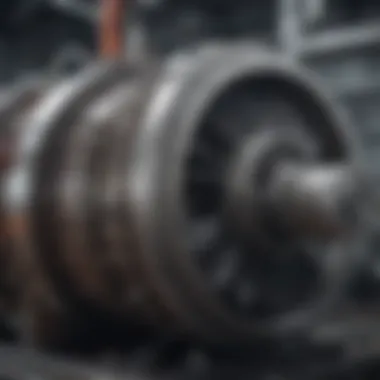
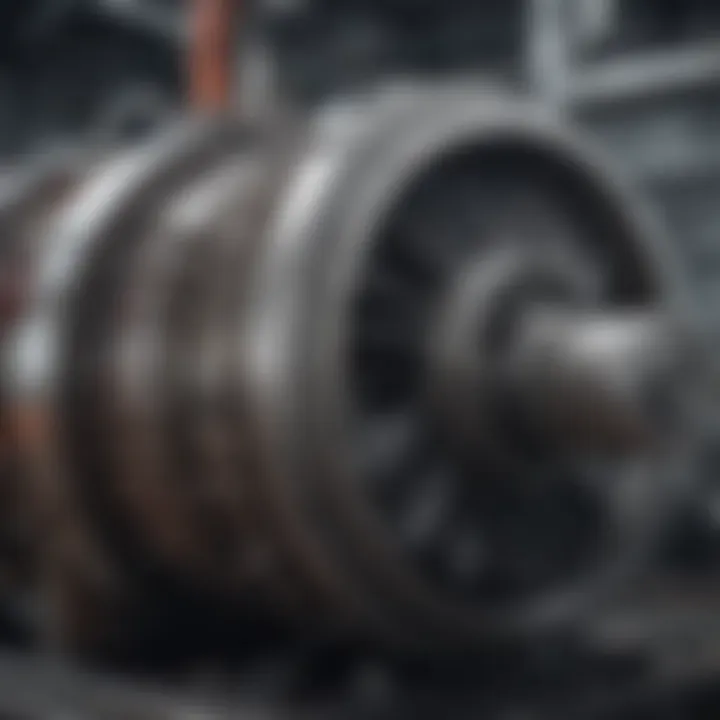
Intro
Electricity generating turbines are essential components in the field of energy production. They transform kinetic energy from various sources into electrical energy. Understanding their functions and types is crucial for those involved in engineering, energy policy, and environmental science. This article delves deeply into the mechanisms, advantages, and challenges associated with turbines, providing a clear guide for students, researchers, educators, and professionals.
Article Overview
Purpose of the Article
The aim of this article is to provide a thorough exploration of electricity generating turbines. It will cover the diverse types of turbines, such as steam, gas, and wind turbines, discussing their operational principles. The article intends to clarify the technological advancements made in turbine design to enhance efficiency and reduce environmental impact.
Relevance to Multiple Disciplines
Electricity generating turbines are relevant not only to engineers but also to environmentalists, economists, and policy-makers. Their design influences energy efficiency and sustainability, which are key considerations in today’s discussions on future energy solutions. Knowledge of turbine technology is crucial for developing energy strategies that align with global sustainability goals.
Research Background
Historical Context
The history of electricity generating turbines traces back to the Industrial Revolution when steam turbines first emerged. These machines harnessed steam produced by heating water, a novel method for generating mechanical work. As technology evolved, gas turbines and hydro turbines became more common, illustrating the diverse applications of turbines across various energy domains.
Key Concepts and Definitions
To comprehend electricity generating turbines efficiently, it is necessary to understand several key concepts:
- Turbine: A device that converts the energy in a fluid into mechanical energy. Fluids can include steam, water, or air.
- Kinetic Energy: The energy possessed by a body due to its motion, which turbines exploit to generate power.
- Efficiency: A measure of how well a turbine converts input energy into output energy, typically represented in percentage.
Each of these elements plays a critical role in the overall performance and impact of electricity generating turbines.
Preamble to Electricity Generating Turbines
Electricity generating turbines are at the heart of modern energy production systems. Their ability to convert various forms of energy into electrical power makes them crucial to meeting the world's increasing energy demands. Understanding the different types, mechanisms, and efficiencies of these turbines provides insights not only into engineering but also into the global transition towards sustainable energy sources.
Definition and Purpose
Electricity generating turbines are mechanical devices that transform kinetic and thermal energy into electrical energy. They function primarily on the principle of rotational motion, where the movement of fluid—be it steam, water, or gas—interacts with the blades of the turbine, causing it to spin. The rotational movement then drives a generator that produces electricity.
The purpose of these turbines extends beyond energy generation. They contribute to energy efficiency by enabling the conversion of raw energy resources into usable forms. They also play a pivotal role in advocates of renewable energy, as many modern turbines are designed to minimize environmental impacts while supporting energy needs.
Historical Development
The development of electricity generating turbines has evolved significantly over the decades. The first steam turbine was invented by Sir Charles Parson in the late 19th century. This innovation represented a breakthrough in energy efficiency and set the stage for the widespread use of steam power in industrial applications.
In the early 20th century, advances in materials science and engineering techniques enhanced turbine design, leading to increased efficiency and output. Gas turbines emerged as an efficient means to generate electricity from natural gas, gaining popularity in the mid-1900s.
As awareness of environmental impacts grew, hydraulic and wind turbines started gaining traction in the latter part of the 20th century. With continuous improvements in technology and design, modern turbines are now equipped to utilize various energy sources, emphasizing the importance of sustainable energy production.
"Turbines have not just shaped the industrial landscape; they are vital in our quest for cleaner energy solutions."
"Turbines have not just shaped the industrial landscape; they are vital in our quest for cleaner energy solutions."
This historical perspective highlights the evolution of electricity generating turbines from simple mechanical devices to sophisticated systems that integrate with contemporary energy challenges.
Types of Turbines
Understanding the types of turbines is crucial in the realm of electricity generation. Each type serves distinct purposes while contributing to the overall efficiency and reliability of power systems. By exploring these variants, we can appreciate their unique roles and implications for energy production. Knowing the specific advantages and disadvantages of each turbine type is essential for making informed decisions in energy planning and management.
Gas Turbines
Operational Principle
Gas turbines function based on the principles of thermodynamics. They convert chemical energy from fuel into mechanical energy. This process involves air compression, fuel injection, and combustion. Hot gases drive the turbine blades, resulting in electricity generation. One key characteristic of gas turbines is their capacity for rapid start-up and operation at varying loads. This makes them a valuable choice for peak load management, accommodating fluctuating power demands.
The efficiency of gas turbines is also notable. Their operational flexibility allows them to perform well even when power demand changes quickly. However, one unique feature is the dependency on high-quality fuel, which can increase operational costs. Additionally, emissions from gas combustion pose environmental challenges that need addressing.
Applications
Gas turbines find extensive applications in power plants, particularly where quick energy supply transitions are essential. They are utilized in peaking power plants, aviation engines, and even in naval ships. The key characteristic here is their adaptability, making them effective in both stationary and mobile environments.
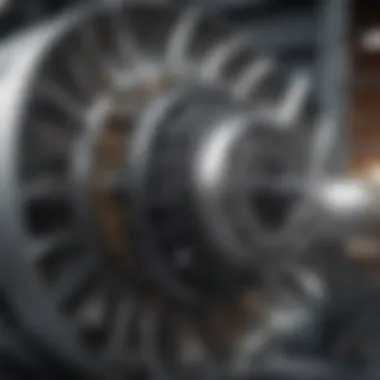
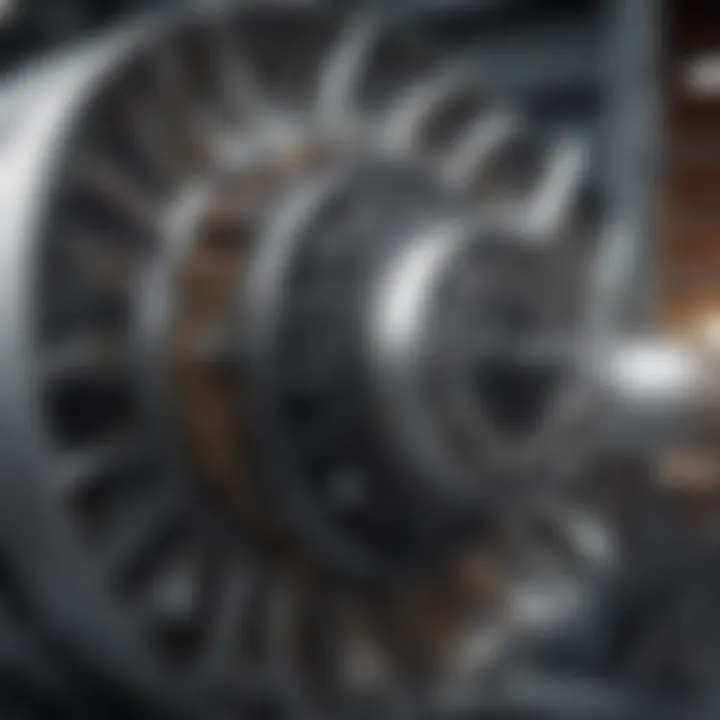
One notable advantage of gas turbines is their ability to combine with steam turbines in combined cycle power plants. This hybrid approach significantly enhances efficiency. However, their dependency on fossil fuels raises concerns regarding sustainability, especially in regions with strict emissions regulations.
Advantages and Disadvantages
The advantages of gas turbines include high power-to-weight ratio and operational flexibility. They can efficiently supply electricity in varying demand situations. However, disadvantages involve relatively high initial costs and significant greenhouse gas emissions.
Overall, gas turbines are a critical component in modern energy solutions, despite their environmental concerns that must be mitigated through technology advancements.
Steam Turbines
Thermodynamic Cycle
Steam turbines operate on the Rankine cycle, which involves converting water into steam through heat, followed by utilizing the steam to drive turbine blades. This process efficiently converts thermal energy into mechanical energy. A key characteristic is their ability to harness waste heat from other processes, improving overall energy efficiency.
Steam turbines are especially beneficial for large-scale power generation. They typically have high efficiency ratings but require significant infrastructure investment. A unique feature is their capability to produce large volumes of energy continuously, which is advantageous in base load power generation.
Applications
Steam turbines are primarily applied in large thermal power plants, including coal, nuclear, and geothermal facilities. Their prevalent use stems from a well-established technology that allows for significant power outputs, especially in continuous operations.
The adaptability of steam turbines is one of their defining characteristics; they can be combined with various heat sources for effective energy conversion. However, they necessitate substantial water resources and infrastructure, which can be limiting in some regions.
Advantages and Disadvantages
Steam turbines offer several advantages, including high efficiency and reliability in steady-state operations. However, their disadvantages include long start-up times and the requirement for extensive water supplies. The engineering complexities also increase costs and maintenance requirements.
In summary, steam turbines remain a staple in power generation due to their high capacity and efficiency, but challenges exist in water management and infrastructure.
Hydraulic Turbines
Types of Hydraulic Turbines
Hydraulic turbines are categorized mainly into two types: impulse and reaction turbines. Impulse turbines, like the Pelton wheel, convert kinetic energy of water into mechanical energy primarily through velocity. Reaction turbines, such as Francis and Kaplan types, utilize the pressure difference in water to generate power.
Choosing the right type of hydraulic turbine depends on water flow characteristics and site-specific conditions. Impulse turbines are advantageous in high-head environments, while reaction turbines excel in lower head scenarios.
Operational Mechanism
The operational mechanism of hydraulic turbines hinges on water flow directing through blades to create motion. This transformation from kinetic and potential energy to mechanical energy is efficient and stable. A vital characteristic is the ability to adjust blade settings in reaction turbines, optimizing performance based on flow conditions.
The unique feature of hydraulic turbines is their minimal environmental footprint compared to fossil fuel turbines. They often employ renewable water sources, which leads to reduced greenhouse gas emissions. However, their construction can disrupt local ecosystems, requiring careful environmental consideration.
Advantages and Disadvantages
The advantages of hydraulic turbines include their efficiency and low operational costs once installed. They have significant longevity and fewer emissions. In contrast, disadvantages consist of high initial investment, potential ecological impacts, and reliance on water availability.
Hydraulic turbines thus represent a key solution in renewable energy, balancing energy needs with environmental responsibility.
Wind Turbines
Design Variations
Wind turbines exhibit varied designs, primarily categorized into horizontal and vertical axis orientations. Horizontal-axis wind turbines are the most common, known for their efficiency in harnessing wind energy through rotating blades aligned with wind flow. Vertical-axis wind turbines, while less conventional, offer advantages in turbulent wind conditions.
The design variations often dictate performance under different environmental conditions. For instance, horizontal turbines typically achieve higher efficiency in open areas, whereas vertical designs can be beneficial in urban settings due to their compact nature.
Operational Mechanics
Wind turbines convert kinetic energy from wind into mechanical energy via rotor movement. The operational mechanics involve pitch control and yaw mechanisms, ensuring optimal blade angles and alignment with wind direction. A significant advantage of wind turbines is their ability to generate power without emissions during operation.
However, there are drawbacks, such as dependence on wind availability and variable electricity production, requiring backup systems or storage solutions.
Advantages and Disadvantages
Wind turbines provide notable benefits, including renewable energy generation and low operational costs. They have minimal environmental impacts once operational compared to fossil fuel counterparts. Yet, challenges include noise concerns, visual impact on landscapes, and the reliance on weather conditions for functionality.
Operational Mechanisms of Turbines
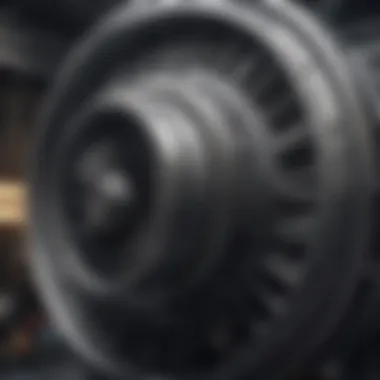
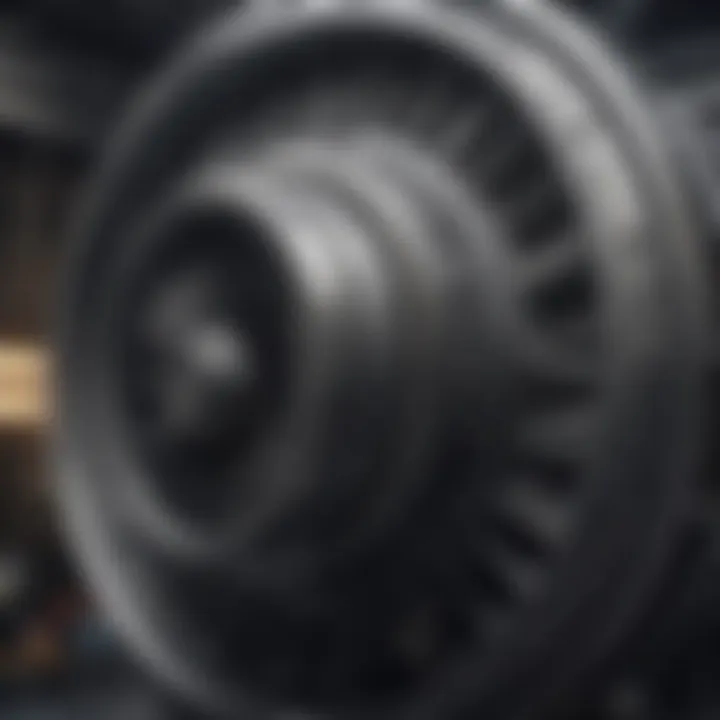
Understanding the operational mechanisms of turbines is crucial in comprehending how they convert various forms of energy into electricity. Turbines are at the heart of power generation, transforming kinetic and thermal energy into electrical energy efficiently. This section explores two primary aspects: the conversion of energy and the control systems that govern turbine operation.
Conversion of Energy
Energy conversion is an essential process that occurs within turbines. It involves taking a specific type of energy, like steam, gas, or water flow, and converting it into mechanical energy that can drive electricity generators.
The basic principle behind energy conversion in turbines is to harness the motion of fluids. For instance, in a steam turbine, high-pressure steam spins blades attached to a rotor; this spinning motion is then transformed into electrical energy through a generator. Similarly, gas turbines utilize hot gases produced from fuel combustion to achieve the same effect.
This conversion is not merely about rotating blades; it involves complex thermodynamic cycles and various engineering efficiencies. For a turbine system to be considered effective, it must minimize energy losses during this process. Factors such as fluid dynamics, blade design, and material properties significantly impact the efficiency of energy conversion.
Control Systems
Control systems in turbines play a significant role in maximizing efficiency and ensuring safety. They regulate turbine operation by adjusting inputs and maintaining optimal performance under varying conditions.
Types of Control Systems
Various types of control systems are utilized in turbine operation.
- PID Controllers: Proportional-Integral-Derivative controllers are common in industrial settings. They provide precise adjustments to maintain the desired operating conditions.
- Feedforward Control: This anticipates changes based on system events, allowing quicker responses to disturbances.
- Adaptive Control: This system adjusts its parameters in real-time depending on the operational context.
Each type of control system serves a specific purpose. The PID controllers, for instance, are popular due to their straightforward implementation and reliability. However, adaptive control can be more complex but allows for higher efficiency in dynamic environments.
Role in Efficiency
Control systems significantly influence turbine efficiency. They ensure that turbines operate within optimal parameters to maximize energy output and minimize waste. The primary function of these systems is to adapt to changes in energy input, weather conditions, and mechanical stresses.
One key characteristic is their responsive nature. A well-designed control system can pick up on variations quickly, maintaining stable operations. However, this responsiveness can introduce complexities in the system's design, requiring more advanced technology and expertise.
In summary, the operational mechanisms of turbines encompass a range of processes that convert energy, along with sophisticated control systems that enhance efficiency. Understanding these dynamics is key to enhancing turbine design and improving energy production.
Efficiency Considerations
Efficiency is a crucial aspect when discussing electricity generating turbines. The performance of these machines directly impacts both the cost-effectiveness of energy production and the environmental footprint. Understanding the factors that influence efficiency can assist in optimizing the design and operation of turbines. Increased efficiency in energy generation not only lowers operational costs but also facilitates the reduction of harmful emissions. This section will delve into the aspects that govern turbine efficiency and how these factors interrelate with overall performance.
Factors Influencing Efficiency
Several key elements affect the efficiency of electricity generating turbines. These include:
- Design features: The geometrical and material choices in turbine construction can greatly influence operational efficiency.
- Operating conditions: Variation in temperature, pressure, and load can affect performance outcomes.
- Maintenance practices: Regular upkeep ensures that turbines run smoothly, minimizing efficiency losses.
- Fuel quality: The type and purity of fuel used in gas turbines can directly affect combustion efficiency.
Each of these factors must be closely monitored and optimized to achieve peak operational efficiency in electricity generating machines.
Performance Metrics
Evaluating the efficiency of electricity generating turbines involves the use of performance metrics. Understanding these metrics is vital for assessing operational capabilities and identifying areas for improvement. Two major performance metrics are:
Overall Efficiency
Overall efficiency is a measure of how effectively a turbine converts the energy input into useful electrical output. This metric is crucial because it determines the amount of fuel required to generate a given amount of electricity.
The key characteristic of overall efficiency lies in its relation to fuel consumption and output power. A higher overall efficiency indicates that less fuel is necessary to produce the same amount of energy, leading to lower operational costs.
Its unique feature is that it integrates various operational aspects, from combustion to mechanical output. Higher overall efficiency can significantly reduce the carbon footprint of energy generation, making it a priority in design and operation.
Specific Fuel Consumption
Specific fuel consumption measures the amount of fuel needed per unit of electricity generated. This metric is particularly significant as it gives a clear indication of turbine performance. Lower specific fuel consumption signifies better performance and efficiency.
The central characteristic of specific fuel consumption is its direct correlation with overall operating costs. Turbines that exhibit low specific fuel consumption can be more economical to run over time.
A unique feature of this metric is its ability to quantify performance concerning environmental impact. Reducing specific fuel consumption is essential for minimizing the carbon emissions associated with power generation. Thus, it often motivates advancements in turbine technology and operational strategies.
In summary, the efficiency of electricity generating turbines is shaped by a combination of design, operational conditions, and maintenance practices. Key metrics like overall efficiency and specific fuel consumption provide insights into performance. As this field continues to evolve, the focus on enhancing these efficiency metrics becomes critical for sustainable energy production.
Environmental Impact
Understanding the environmental impact of electricity generating turbines is essential as they play a significant role in energy production. These turbines contribute to the wider conversation about sustainability and ecological balance. Their efficiency and operational mechanisms have direct consequences for both local ecosystems and global climate patterns. The choices made today in turbine technology can influence future environmental health and energy sustainability. It is necessary to consider how these machines interact with the natural world, as their emissions, resource use, and waste output matter greatly in the fight against climate change.
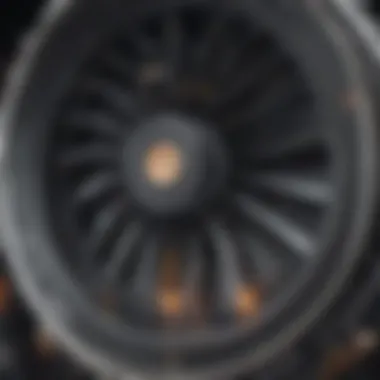
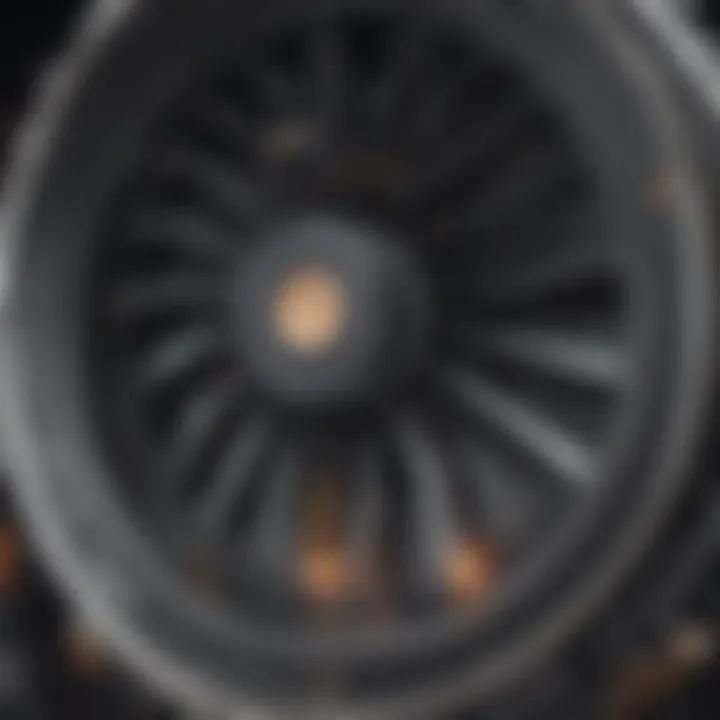
Carbon Footprint
The carbon footprint of electricity generating turbines varies significantly depending on the type of turbine and the fuel source used. Gas turbines, for example, emit less CO2 compared to coal-fed steam turbines. However, regardless of type, any fossil fuel combustion contributes to greenhouse gases that trap heat in the atmosphere. Therefore, understanding the carbon footprint provides a clearer picture of how clean or damaging a particular turbine design or operation might be. This understanding is vital for policy-makers and engineers as they work towards adopting cleaner technologies in the future.
Mitigation Strategies
To address the challenges posed by turbine-related emissions, several mitigation strategies have emerged. These strategies can be categorized into technological innovations and regulatory frameworks.
Technological Innovations
Technological advancements play a significant role in reducing the environmental impact of turbines. Innovations such as carbon capture and storage (CCS) are critical. CCS technology can effectively capture up to 90% of the CO2 emissions produced from fossil fuel use in electricity generation. This significant reduction is possible because of sophisticated methods that separate carbon dioxide from other gases.
The key characteristic of these innovations is their ability to integrate with existing turbine systems, thus requiring less infrastructure overhaul. While this technology offers numerous advantages, such as reduced environmental pollution, it also faces criticism. High costs and the requirement for substantial energy input for operation limit its current applicability. Despite these challenges, the long-term potential of technological innovations in making turbines more sustainable is promising.
Regulatory Frameworks
Regulatory frameworks are another fundamental aspect of environmental impact mitigation. Government regulations often dictate strict emission standards for turbines, encouraging manufacturers to adopt cleaner technologies. Such frameworks not only promote innovation but also safeguard public health and the environment from harmful pollutants.
A significant characteristic of these regulations is their ability to enforce compliance among energy producers. While these frameworks are beneficial in ensuring lower emissions, they can sometimes lead to resistance from industry stakeholders due to perceived limitations on production capabilities. Nevertheless, the unique feature of regulatory frameworks lies in their potential to drive long-term environmental improvements and compliance with international agreements on air quality and emissions.
"Mitigation strategies, combining technological innovations with robust regulatory frameworks, create a more sustainable future for electricity generation."
"Mitigation strategies, combining technological innovations with robust regulatory frameworks, create a more sustainable future for electricity generation."
Future Trends in Turbine Technology
The topic of Future Trends in Turbine Technology holds considerable significance among professionals and academics alike. This section provides insights into the ways in which turbine technology is evolving to meet the increasing demand for efficient and sustainable energy generation. It explores innovative materials utilized in turbine construction, as well as the integration of turbines with renewable energy sources. Understanding these trends is crucial, as they offer not only operational advantages but also promote environmental sustainability.
Advancements in Materials
Recent advancements in materials technology are profoundly influencing the efficiency and longevity of electricity generating turbines. Engineers are increasingly turning to advanced composites and alloys that can withstand extreme conditions. For instance, high-temperature superalloys are now used in gas turbines to enhance thermal efficiency and durability. Moreover, lighter yet strong materials contribute to designs that minimize weight without sacrificing performance.
Some notable material advancements include:
- Carbon Fiber Composites: These materials improve weight savings while maintaining high strength, which is invaluable in rotor and blade manufacture.
- Ceramic Matrix Composites: These offer significant advantages in high-temperature environments, extending the operational life of turbine components.
- Nanostructured Metals: They demonstrate superior mechanical properties and improve fatigue resistance.
These innovations not only enhance performance but also provide manufacturers with tools to optimize operational costs. By decreasing wear and tear, these materials lead to less frequent replacements and downtime, ultimately fostering more sustainable energy production.
Integration with Renewable Energy Sources
The integration of turbine technology with renewable energy sources represents a critical trend in modern energy landscapes. As the world shifts towards sustainable practices, turbines can no longer focus solely on traditional energy production methods. Instead, they must adapt to work alongside wind, hydro, and solar power systems effectively.
Wind turbines, for instance, are being designed to efficiently operate even at low wind speeds. Enhanced control systems allow these turbines to maximize energy capture and enhance stability during fluctuating conditions.
Some important aspects of this integration include:
- Hybrid Systems: The combination of wind turbines and solar panels can lead to a more stable power supply.
- Smart Grid Technologies: These systems facilitate the coordination of various energy sources, optimizing overall energy production and consumption.
- Energy Storage Solutions: Innovations in battery technologies and other energy storage methods are crucial for managing supply and demand fluctuations in renewable energy.
As turbine technology advances, the collaboration with renewable energy sources will likely accelerate, producing cleaner energy and reducing reliance on fossil fuels. This reflects a proactive approach toward combating climate change while ensuring that energy needs are met sustainably.
Closure
The conclusion of an article on electricity generating turbines plays a crucial role in summarizing the key themes discussed throughout the text. It serves as a consolidation of the information, reiterating the importance of understanding how turbines function in modern energy production. By distilling the main points, this section provides clarity and facilitates retention of the material for the reader.
Some specific elements to consider in the conclusion are:
- Recap of Major Types: Emphasizing the distinctions among gas, steam, hydraulic, and wind turbines highlights their unique applications and contributions to energy generation.
- Efficiency and Environmental Impact: Addressing how advancements in turbine technology correlate with efficiency improvements helps underscore the role of innovation in reducing carbon footprints and enhancing sustainability.
- Future Trends: Finally, a brief overview of future developments in turbine technology drives home the dynamic nature of the field, inviting further exploration into how these innovations will affect energy sources moving forward.
The benefits of revisiting these points in the conclusion cannot be understated. This reinforcement not only aids comprehension but also encourages readers to engage with the ongoing discourse surrounding energy generation and its implications for our planet.
Summary of Key Points
This article has traversed the foundational aspects of electricity generating turbines, detailing their types, operational mechanisms, and efficiency metrics. Each turbine type was examined in terms of its operational principles and respective applications, offering insights into:
- Gas Turbines: Notable for their high efficiency and versatility, used in various applications from simple power generation to complex combined cycles.
- Steam Turbines: Integral to many large power plants, they utilize steam produced by boiling water to drive the turbine blades.
- Hydraulic Turbines: Essential in utilizing water flow energy, showcasing diverse designs based on the nature of water sources.
- Wind Turbines: Representing a growing focus on renewable energy, their design and mechanics cater to harnessing kinetic energy from wind.
Additionally, the article highlighted the significance of efficiency considerations and the environmental impacts of turbine operations. It tackled both positive advancements and ongoing challenges associated with turbines in the context of global energy needs.
Future Outlook
Looking ahead, the evolution of turbine technology is set to unfold through several promising developments. Key trends include:
- Advancements in Materials: The introduction of lightweight and high-strength materials promises enhanced performance, leading to larger and more efficient turbines.
- Integration with Renewable Energy Sources: There is increasing interest in developing hybrid systems, combining traditional turbine technology with renewable energy systems to improve overall efficiency and sustainability.
- Automation and Smart Technology: The adoption of advanced control systems equipped with AI will optimize turbine performance and maintenance schedules, ensuring stability and longevity.
As we progress economically and environmentally, understanding these trends provides insight into how turbines will adapt to meet future energy demands. The importance of resilience and innovation in this sector cannot be overstated, solidifying the critical role that electricity generating turbines will continue to play.