Innovative Energy Transfer Solutions Explained
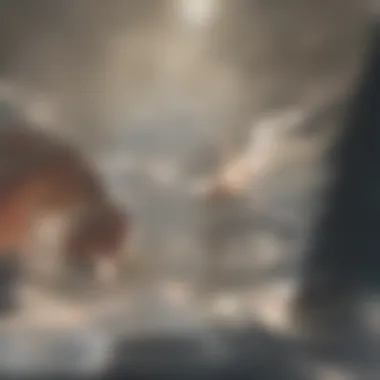
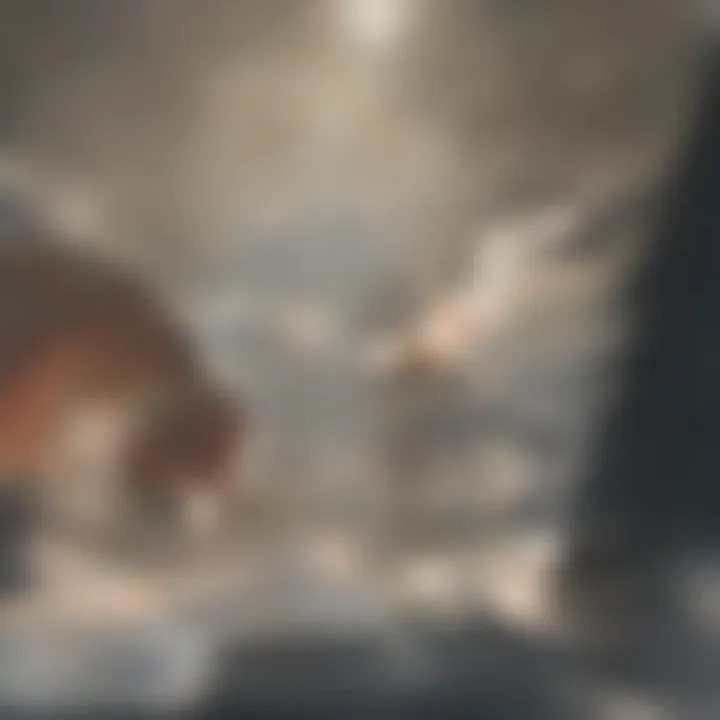
Intro
Energy transfer is a critical aspect of our modern world. It involves the movement and conversion of energy from one form to another, enabling a wide range of technological applications. The growing demand for sustainable energy solutions and efficient transfers makes this a timely topic.
As we explore this article, we will cover various methodologies and innovations within the realm of energy transfer solutions. Our aim is to provide a comprehensive understanding for students, researchers, educators, and professionals alike.
Article Overview
Purpose of the Article
The purpose of this article is to elucidate the principles guiding energy transfer solutions and highlight the latest advancements. Innovations in this field can bridge gaps in energy efficiency and create avenues for sustainability. By dissecting various methodologies employed across sectors, we aim to demonstrate the relevance and application of these solutions.
Relevance to Multiple Disciplines
Energy transfer solutions touch upon numerous disciplines. Here are some relevant fields:
- Engineering: Development of efficient systems for energy transfer.
- Environmental Science: Studying the impact of energy solutions on ecosystem sustainability.
- Economics: Assessing the financial implications of energy transfer innovations.
The interconnectivity among these fields reinforces the importance of understanding energy transfer in a multidisciplinary context.
Research Background
Historical Context
Historically, energy transfer has been essential since the dawn of civilization. From basic methods like burning wood to advanced systems utilizing renewable resources, methods have evolved tremendously. The rise of industrialization in the 19th century marked a pivotal shift, showcasing the need for more effective energy transfer methods to power machinery.
Key Concepts and Definitions
Understanding key concepts in energy transfer is fundamental. Some essential terms include:
- Conduction: The process of energy transfer through direct contact.
- Convection: The transfer of energy through fluids due to density differences.
- Radiation: Transfer of energy through electromagnetic waves.
These concepts will be addressed in detail throughout the article, providing a solid foundation for the discussions that follow.
"Energy transfer methods have shaped human progress and will continue to play an integral role in future innovations."
"Energy transfer methods have shaped human progress and will continue to play an integral role in future innovations."
In summary, this exploration will offer an insightful examination of energy transfer solutions. It will cover principles, innovations, and implications for various sectors. Read on to uncover case studies and potential advancements that highlight the importance of this field.
Prologue to Energy Transfer Solutions
Energy transfer is a critical component of our modern world. It encompasses the various ways energy moves from one system to another. Understanding energy transfer solutions is essential for numerous disciplines, including engineering, environmental science, and economics. This section provides a foundational overview of energy transfer and its significance.
Defining Energy Transfer
Energy transfer refers to the processes through which energy changes form and moves between objects or systems. This can occur through various mechanisms, including conduction, convection, and radiation. Each mechanism has unique characteristics and applications. For instance, conduction involves direct contact between substances, leading to the transfer of thermal energy through molecular collisions. On the other hand, convection relies on fluid movement, which transports heat through the bulk movement of fluids. Lastly, radiation allows energy transfer through electromagnetic waves, a process that can occur in a vacuum.
Understanding these definitions allows for better comprehension of the energy systems in our daily lives and planetary systems.
Importance of Energy Transfer Solutions
The study of energy transfer solutions is significant for several reasons:
- Sustainability: With pressing concerns about global warming and resource depletion, optimizing energy transfer can improve energy efficiency, thus supporting sustainable practices.
- Innovation: Advancements in technology, such as smart grids and energy harvesting, highlight the need to adapt and improve energy transfer solutions.
- Economic Impact: Energy systems affect various sectors of the economy. Improved methods lead to cost savings and increased productivity.
Effective management of energy transfer can lead to a more sustainable and efficient future economy.
Effective management of energy transfer can lead to a more sustainable and efficient future economy.
Fundamental Principles of Energy Transfer
Understanding the fundamental principles of energy transfer is crucial, as they underpin all applications of energy systems. Knowledge of these principles facilitates better design, implementation, and optimization of energy transfer solutions. This section explores two central themes: thermodynamics and conservation of energy. Both these concepts are vital for grasping how energy is managed and utilized efficiently across various contexts.
Thermodynamics and Energy Flow
Thermodynamics is the branch of physics that deals with heat and temperature, and their relation to energy and work. In the context of energy transfer, thermodynamics provides the frameworks that govern how energy flows from one system to another. There are four laws of thermodynamics, but the first and second laws are particularly pertinent.
The First Law of Thermodynamics, often dubbed the law of energy conservation, states that energy cannot be created or destroyed, only transformed. This principle explains why energy inputs must equal energy outputs in any closed system.
The Second Law of Thermodynamics introduces the concept of entropy, indicating that energy transformations are not always 100% efficient. As energy changes form, some energy is often lost as waste heat, increasing entropy in the system. This means that energy flow must be managed carefully to minimize losses and enhance efficiency.
In practical terms, understanding how thermodynamics impacts energy transfer can lead to improved designs in systems ranging from power plants to refrigeration units. Engineers can create systems that maximize energy efficiency, thereby reducing costs and environmental impact.
Conservation of Energy
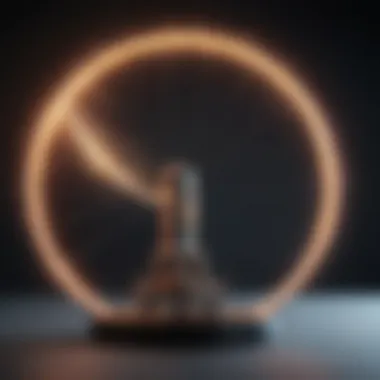
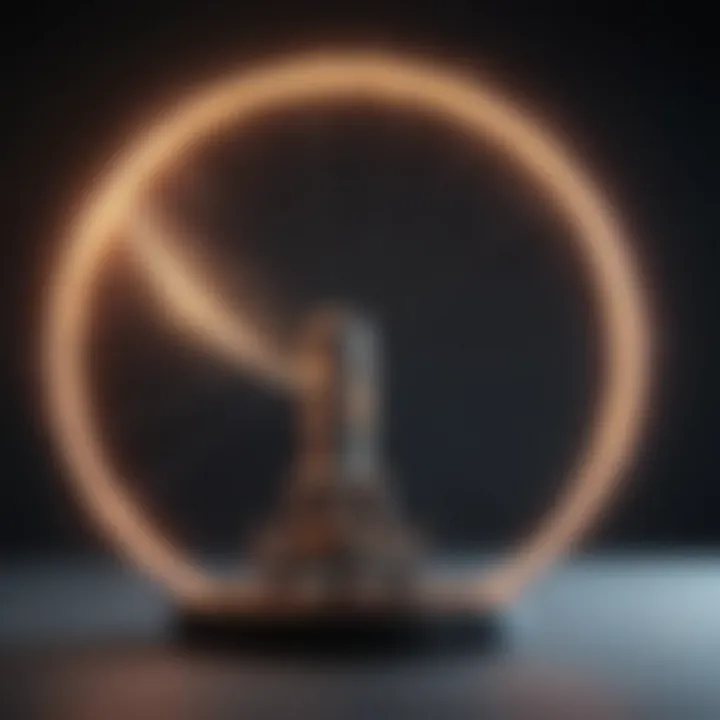
The conservation of energy principle is integral to all forms of energy transfer. It asserts that the total energy in a closed system remains constant. This means that energy can change forms—such as from kinetic to potential—but the total amount remains unchanged.
When applied to energy transfer solutions, conservation principles lead to several important considerations:
- Efficiency Analysis: It is essential to assess how much usable energy is produced compared to what is input. High efficiency reduces waste and maximizes output.
- Sustainability: By recognizing that energy must be conserved and not wasted, systems can be designed with a focus on renewable sources, promoting long-term sustainability in energy use.
- Innovation: Insights from conservation principles drive innovation in energy technologies. Researchers explore ways to harness wasted energy to create more robust and efficient systems.
In summary, the foundational principles of thermodynamics and energy conservation are indispensable in the study of energy transfer. These concepts not only frame the scientific understanding of energy systems but also underscore the importance of efficiency and sustainability in modern energy practices.
"The first law of thermodynamics asserts that energy cannot be created or destroyed, providing a vital framework for understanding energy transfer across systems."
"The first law of thermodynamics asserts that energy cannot be created or destroyed, providing a vital framework for understanding energy transfer across systems."
Ultimately, recognizing these fundamental principles empowers students, researchers, and professionals alike to develop (and apply) more effective energy transfer solutions.
Types of Energy Transfer Methods
Energy transfer methods are fundamental to understanding how energy moves and interacts across various systems. Each method offers unique benefits and considerations that play a critical role in applications across multiple sectors. A comprehensive grasp of these methods is essential for advancing technologies and enhancing efficiency in how we utilize energy. The three primary methods of energy transfer include conduction, convection, and radiation. Each method serves specific needs and applications, which will be explored in detail.
Conduction
Conduction is the process of heat transfer through solid materials without the movement of the material itself. It occurs when two objects at different temperatures come into contact, allowing thermal energy to flow from the hotter object to the cooler one. The effectiveness of conduction depends on the properties of the materials involved. Materials like metals are excellent conductors because they have free electrons that can move easily, while insulators such as rubber or wood have tightly bound electrons that limit movement.
Key Characteristics of Conduction:
- Temperature Gradient: The rate of heat transfer is directly related to the temperature difference between two objects. A larger difference results in faster energy transfer.
- Material Properties: Conductivity varies among materials, influencing efficiency.
- Surface Area: Increased contact areas facilitate more effective heat transfer.
Conduction is crucial in many applications, from cooking utensils to heating systems, making it a key element in energy transfer solutions.
Convection
Convection involves the transfer of heat through fluids, which can be either liquids or gases. In this process, warmer parts of the fluid rise while cooler parts sink, creating a circulating current. There are two types of convection: natural and forced. Natural convection occurs due to density differences in the fluid, while forced convection is facilitated by external means, such as a fan or pump.
Advantages of Convection:
- Efficient Heat Distribution: It allows for the rapid distribution of heat throughout a volume, making it ideal for heating and cooling systems.
- Application Versatility: Convection is employed in various technologies, including HVAC systems and cooking methods.
Convection enables effective thermal management in many systems, highlighting its significance in energy transfer solutions.
Radiation
Radiation is distinct from conduction and convection as it does not require a medium for heat transfer. It involves the emission of electromagnetic waves, primarily in the infrared spectrum, which transfer energy through space. All objects emit radiation, and the amount depends on their temperature and surface characteristics.
Essential Aspects of Radiation:
- Line of Sight: Radiation travels in straight lines, meaning it requires a clear path between the source and the recipient.
- Distance Dependency: The intensity of radiant energy decreases with distance, necessitating proximity for effective heat transfer.
- Material Interaction: Certain materials can absorb, reflect, or transmit radiation differently, affecting overall energy transfer efficiency.
Radiation plays a vital role in applications ranging from solar heating to thermal insulation approaches, ensuring its importance in the vast landscape of energy transfer solutions.
As we explore these three methods, it becomes evident that each method has its context and applications, contributing to the comprehensive analysis of energy transfer solutions.
Emerging Technologies in Energy Transfer
Emerging technologies represent a transformative force in energy transfer. These innovations not only enhance efficiency but also offer sustainable alternatives to traditional methods. In a world where energy demands continue to rise, the exploration and implementation of these technologies are critical. They help reduce operational costs and mitigate environmental impacts. Understanding new methodologies can provide valuable insights into future energy scenarios.
Nanotechnology Applications
Nanotechnology applications in energy transfer involve manipulation of materials at the molecular or atomic level. This nanoscale engineering leads to significant improvements in energy efficiency. For example, nanomaterials enhance thermal conductivity, making heating and cooling systems more effective. They also enable the development of superior energy storage devices.
- Solar Energy: Nanotechnology can increase the efficiency of solar panels. By incorporating nanomaterials, researchers have developed photovoltaic cells that absorb more sunlight and convert it into electricity.
- Thermal Insulation: Nanocomposite materials provide better insulation properties. This results in reduced energy consumption for heating and cooling buildings.
- Batteries and Supercapacitors: Devices using nanostructured electrodes can store more energy and have longer life cycles.
Smart Grid Innovations
Smart grid technologies revolutionize how energy is distributed and consumed. They utilize digital communication and automation to improve the reliability and efficiency of the energy system. This enhances energy management and allows for real-time monitoring.
- Demand Response Programs: These help manage consumer energy usage during peak times, reducing the need for additional power plants.
- Advanced Metering Infrastructure (AMI): AMI allows for better monitoring of energy consumption and enables dynamic pricing, encouraging conservation.
- Integration of Renewable Energy Sources: Smart grids facilitate the integration of solar, wind, and other renewable sources into the energy mix, promoting cleaner energy.
The deployment of smart grid technology can lead to a significant reduction in energy loss, making systems more resource-efficient and sustainable.
The deployment of smart grid technology can lead to a significant reduction in energy loss, making systems more resource-efficient and sustainable.
Energy Harvesting Technologies
Energy harvesting technologies capture and store energy from various sources instead of relying on traditional generation methods. They contribute to decentralizing energy production and supporting sustainable practices.
- Piezoelectric Devices: These generate electricity from mechanical stress, such as footsteps on a surface. They can power small devices in urban areas without needing a power grid connection.
- Thermal Energy Harvesting: This technology captures waste heat from industrial processes or even human bodies. It can then convert it into usable electricity.
- Solar Harvesting: Beyond solar panels, advances in materials enable the creation of flexible solar cells that can be integrated into various surfaces, expanding their application.
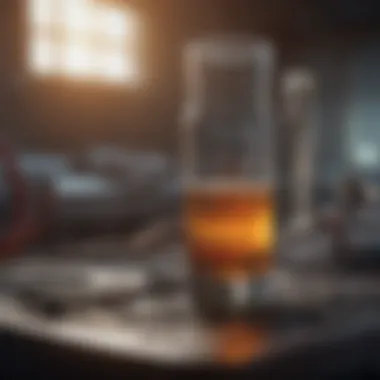
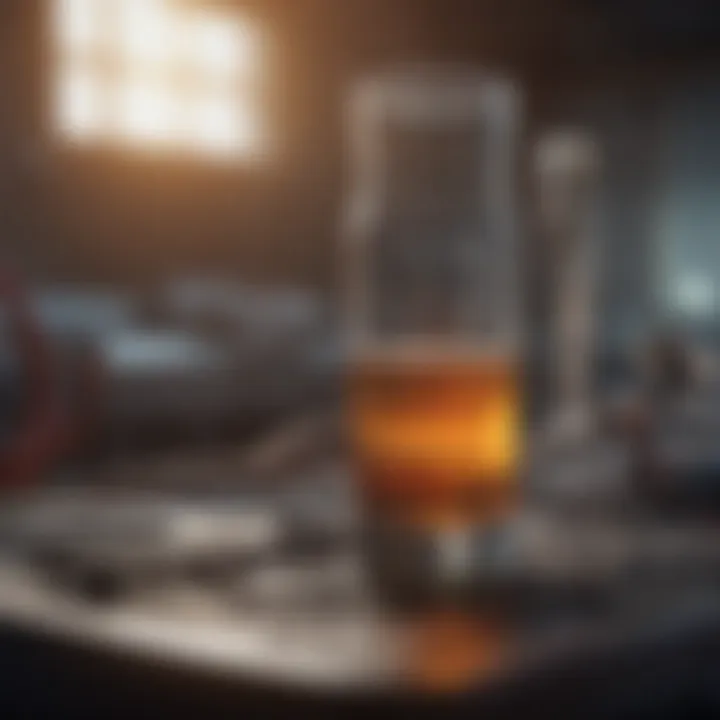
The importance of these technologies cannot be overstated. They present new opportunities for improving energy efficiency and reducing environmental footprints. As we continue to explore these innovations, their role in shaping a sustainable energy future will be invaluable.
Case Studies in Energy Transfer Solutions
Case studies provide valuable insights into the practical applications and effectiveness of energy transfer solutions. They illustrate how theoretical principles are implemented in real-world scenarios. By examining specific examples, we can identify both successful strategies and areas for improvement. This understanding aids in advancing future technologies and methodologies.
Industrial Applications
In industrial settings, energy transfer solutions play a crucial role in enhancing efficiency. One prominent case is the use of heat exchangers in chemical processing plants. These devices transfer thermal energy between two or more fluids. They are essential for maintaining the desired reaction temperatures in reactors, significantly impacting yields and energy consumption.
Another great example is in the manufacturing sector where combined heat and power (CHP) systems are utilized. These setups simultaneously generate electricity and useful heat from the same energy source, often natural gas. The integration of CHP can lead to substantial savings in energy costs while also reducing greenhouse gas emissions.
The benefits found in these applications have broad implications, including increased productivity, cost savings, and minimized environmental footprints. Industries adopting such solutions often achieve a competitive advantage in today’s economy.
Residential Solutions
Energy transfer solutions also find relevance in residential contexts. A notable case involves solar thermal systems, which capture and convert solar energy into heat for domestic hot water. These systems have become more prevalent as homeowners seek renewable energy sources to reduce utility bills and reliance on fossil fuels.
In a different vein, smart thermostats have transformed how heat is distributed in homes. By leveraging data and machine learning, these devices optimize heating patterns based on resident behavior. This can lead to substantial energy savings, making warmth more manageable while lowering costs. The integration of such technology highlights a shift towards more sustainable and efficient energy use in residential settings.
Transportation Systems
Transportation is another sector witnessing advancements in energy transfer solutions. An example of this is the emerging use of electric vehicle (EV) charging stations. These platforms not only facilitate the transfer of electricity to EVs but do so in increasingly innovative ways. Fast-charging stations, for instance, can recharge vehicles significantly quicker than traditional chargers, addressing concerns about the practicality of EVs in daily life.
Moreover, the incorporation of regenerative braking systems in electric and hybrid vehicles showcases a remarkable use of energy transfer technology. By capturing energy usually lost during braking and returning it to the battery, these systems enhance overall efficiency and extend battery life.
As transportation evolves, these case studies underscore the ongoing need for effective energy transfer methods. They contribute to greener transportation solutions while also meeting societal needs for mobility and environmental stewardship.
"Understanding practical applications of energy transfer solutions provides a critical foundation for future developments in this field."
"Understanding practical applications of energy transfer solutions provides a critical foundation for future developments in this field."
Case studies reveal the diverse applications of energy transfer solutions across sectors. They reinforce the importance of innovation and efficiency in meeting modern energy demands.
Societal Implications of Energy Transfer Solutions
Energy transfer solutions play a crucial role in shaping the modern world. They affect various aspects of society, influencing both our economy and environment. As energy needs continue to grow, understanding these implications is essential. This section covers three significant dimensions of societal implications: economic considerations, environmental impact, and the role of policy and regulation.
Economic Considerations
Energy transfer solutions impact the economy directly and indirectly. On one hand, they foster job creation in sectors such as renewable energy, manufacturing, and technology. Innovations in energy transfer technologies can lead to significant cost savings for businesses. For instance, advancements in energy-efficient systems can lower operational costs, boosting a company’s bottom line.
On the other hand, transitioning to more sustainable energy solutions carries economic challenges. Initial investments may be high, deterring some companies from adopting new technologies. There can be resistance within industries reliant on traditional energy sources, which may impact job security for those workers. Therefore, while energy transfer innovations can enhance economic productivity, careful planning and investment strategies are necessary to minimize disruption and benefit various socioeconomic groups.
Environmental Impact
The environmental implications of energy transfer solutions are profound. Optimizing energy transfers can significantly reduce carbon footprints, contributing to global efforts against climate change. Efficient energy transfer methods help decrease waste and minimize pollution. For example, electric vehicles utilize advanced energy transfer technologies, reducing emissions in urban areas.
However, not all energy transfer methods are created equal. Some technologies may involve harmful processes during production or disposal phases. It is essential to assess the entire lifecycle of energy solutions to ensure they fulfill their promise of sustainability. Mitigating negative effects on biodiversity and natural habitats is also critical as we develop new solutions. Therefore, a meticulous evaluation of environmental impacts can ensure the long-term sustainability of energy transfer innovations.
Policy and Regulation
Effective policies and regulations are fundamental to fostering advancements in energy transfer solutions. Governments must establish frameworks that promote research and development while encouraging private sector investment. Clear regulations can provide guidelines that drive innovation safely and sustainably.
Additionally, international cooperation is vital. Energy transfer technologies are not confined by borders. Joint initiatives between countries can result in shared knowledge and resources, enhancing overall effectiveness. Policymakers must balance innovation with safety and community welfare.
"Effective policies can pave the way for sustainable energy solutions, reducing environmental impact and fostering economic growth."
"Effective policies can pave the way for sustainable energy solutions, reducing environmental impact and fostering economic growth."
Comparative Analysis of Energy Transfer Solutions
Analyzing energy transfer solutions is crucial in understanding how different methods can either enhance or hinder the efficiency of energy use. By comparing traditional and modern approaches, readers can appreciate the evolution of technology and its impact on energy systems. This section elucidates the variations in methodology, efficiency, and the implications of these differences.
Traditional vs. Modern Methods
Traditional methods of energy transfer often rely on established practices and technologies. For example, conduction in solid materials has been the primary mechanism of heat transfer for centuries. Materials like metals exemplify this approach, as they conduct heat efficiently enabling various applications such as cooking and heating.
Modern methods, in contrast, attempt to circumvent limitations of traditional techniques through innovative technology. Advanced composite materials and coating technologies are utilized for rooms that retain energy while minimizing heat loss. The advent of materials like aerogel showcases a modern approach which significantly improves insulation efficiency without adding bulk.
The differences between these two categories can be summarized as follows:
- Efficiency: Modern methods often incorporate smart technologies that optimize energy flow, compared to traditional systems that may not maximize utility.
- Sustainability: Contemporary solutions tend to consider environmental impact more seriously, promoting renewable energy and reducing emissions.
Energy Efficiency Metrics
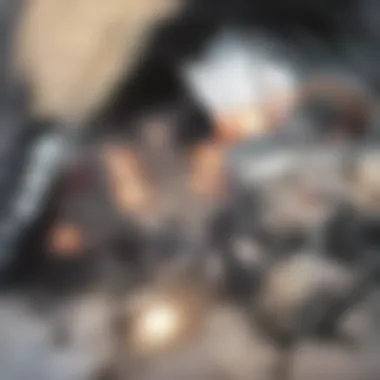
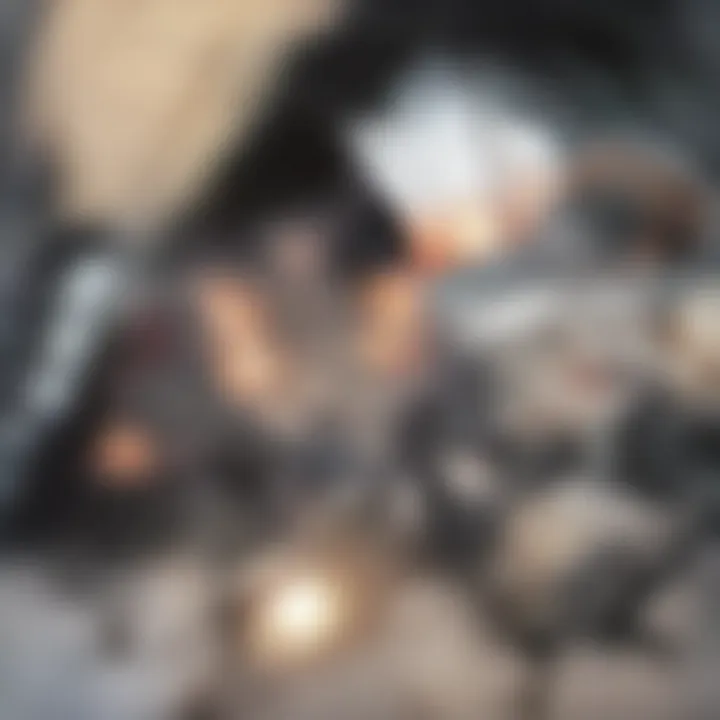
Energy efficiency metrics provide essential insights into the performance and sustainability of energy transfer solutions. These metrics include various measures that quantify how effectively energy is utilized and conserved in different systems.
Some important energy efficiency metrics are:
- Coefficient of Performance (COP): This shows the efficiency of heating and cooling systems by comparing energy output to energy input.
- Energy Efficiency Ratio (EER): Utilizing the same principle, this metric is used particularly for air conditioning systems, indicating how much cooling is produced per energy unit consumed.
- Annual Energy Use: This metric captures the overall consumption per year, helping identify long-term trends and areas for improvement.
Effective analysis of these metrics will allow stakeholders to make informed decisions and optimize their energy transfer systems, which ultimately leads to reduced costs and improved environmental outcomes.
Effective analysis of these metrics will allow stakeholders to make informed decisions and optimize their energy transfer systems, which ultimately leads to reduced costs and improved environmental outcomes.
Future Directions in Energy Transfer Research
The field of energy transfer research is at a pivotal point. New advancements and interdisciplinary efforts are essential in addressing growing energy demands sustainably. Researchers and industry professionals must explore innovative solutions that enhance efficiency, accessibility, and reliability of energy transfer methods. Understanding the future directions in this domain provides valuable insight into upcoming trends and potential breakthroughs.
Innovations on the Horizon
Innovation serves as the backbone of progress in energy transfer solutions. Several exciting developments are anticipated:
- Solid-state batteries are being developed as a safer and more efficient alternative to conventional lithium-ion batteries. These batteries can enable improved energy storage capacity.
- Thermal energy transfer systems are evolving, allowing for better heat management in buildings and industry. Smart materials that change their properties based on temperature are becoming a focus for researchers.
- Wireless energy transfer technologies are gaining traction. They promise to eliminate cables and improve user convenience, especially in consumer electronics and electric vehicles.
- Artificial intelligence and machine learning can optimize energy management systems. This new trend can lead to smarter grids, capable of predicting consumption patterns and adjusting supply accordingly, improving overall efficiency.
Each of these innovations has substantial implications for various industries, potentially leading to significant efficiency improvements and lower operational costs.
Interdisciplinary Approaches
The complexity of energy transfer necessitates a collaborative approach across various disciplines:
- Engineering and technology play crucial roles in creating optimized systems. Research teams that include experts from these fields are essential for the development of new materials and designs.
- Environmental science informs the sustainability of energy solutions. Understanding the ecological impact of energy transfer methods helps in shaping policies and innovations that minimize harm to the environment.
- Economics and social sciences contribute by assessing the feasibility and accessibility of new technologies. This perspective ensures that innovations are not only technically sound but also economically viable and socially acceptable.
Fostering collaborations among these diverse fields enhances the capability to confront challenges in energy transfer. This approach is critical as it creates a well-rounded understanding of both the technological possibilities and the societal implications.
"The most promising strategies for future energy solutions will emerge from the collaboration of interdisciplinary teams, addressing both the scientific and social dimensions of energy transfer."
"The most promising strategies for future energy solutions will emerge from the collaboration of interdisciplinary teams, addressing both the scientific and social dimensions of energy transfer."
Challenges in Energy Transfer Solutions
Understanding the challenges in energy transfer solutions is critical to optimizing their application. As technology evolves, so does the complexity of these systems. This section delves into two primary aspects that hinder progress: technical barriers and economic constraints. By addressing these challenges, stakeholders can enhance performance and support sustainable energy practices.
Technical Barriers
Technical barriers present significant obstacles in the development and implementation of energy transfer solutions. These barriers often arise from limitations in current technology and processes. For example, the efficiency of thermal energy transfer can be compromised by poor material choices or inadequate system designs. System interoperability also poses a challenge. Often, devices and technologies produced by different manufacturers do not communicate effectively. This can lead to inefficiencies and increased maintenance costs.
Specific technical barriers include:
- Material Limitations: Many energy transfer systems rely on materials that may not withstand extreme temperatures or environmental conditions. Finding durable alternatives is essential.
- Insufficient Research: Certain energy transfer methodologies may lack comprehensive research, limiting innovation and practical application in the industry.
- Complex Integration: Integrating various systems, like renewable resources and traditional power grids, often leads to complications in system design and customer interfaces.
"The integration of new technologies into existing frameworks remains complex and costly."
"The integration of new technologies into existing frameworks remains complex and costly."
Addressing these technical barriers requires collaboration among engineers, researchers, and policymakers. Investing in research and development can ensure advancements in materials and methods used in energy transfer.
Economic Constraints
Economic constraints significantly impact the implementation of energy transfer solutions. These constraints can limit funding for innovation and affect the affordability of new technologies. Investors are often risk-averse when it comes to sectors seen as unstable or lacking in immediate profitability.
Factors contributing to economic constraints include:
- High Initial Costs: The upfront investment needed for new energy transfer technologies can be substantial, discouraging organizations from pursuing them.
- Market Uncertainty: Fluctuations in energy prices and regulatory changes can create an uncertain market environment, making it difficult for companies to plan long-term investments.
- Limited Incentives: In some regions, existing policies may not provide sufficient incentives for transitioning to more efficient energy transfer methods, affecting adoption rates.
In navigating these economic constraints, governmental support and private sector involvement are crucial. Policies that provide financial incentives for innovation can promote broader acceptance of new energy transfer technologies.
In summary, tackling the challenges in energy transfer solutions is vital for future progress in the field. By addressing both technical barriers and economic constraints, the industry can enhance energy efficiency and pave the way for sustainable practices.
Finale and Summary of Findings
In this article, we have explored the multifaceted realm of energy transfer solutions, delving into principles, innovations, and practical applications. Energy transfer is crucial in numerous contexts, from residential heating systems to large-scale industrial processes. This conclusion serves to emphasize the importance of efficient energy transfer mechanisms in fostering sustainability and enhancing operational effectiveness across diverse sectors.
The key elements discussed throughout the article reflect the interplay between technological advances and practical methodologies. Understanding how energy flows—and identifying methods to optimize this flow—can lead to significant improvements in efficiency. These improvements not only conserve resources but also reduce costs for consumers and industries alike. Consequently, stakeholders are urged to consider the findings presented herein to enhance their own energy transfer systems.
In summary, the implications of energy transfer solutions extend far beyond operational benefits. They touch on economic, environmental, and technological aspects that are vital as societies strive toward sustainability. The findings indicate that investing in better energy transfer methods can yield dividends in various contexts, making this topic profoundly relevant.
Key Takeaways
- Efficiency: Optimizing energy transfer leads to enhanced operational efficiency and reduced costs.
- Sustainability: Improved methods of energy transfer contribute to environmental sustainability through resource conservation.
- Technological Integration: Emerging technologies such as smart grids show great potential in revolutionizing energy transfer processes.
- Interdisciplinary Approaches: Collaboration across various fields is necessary to address energy transfer challenges effectively.
Call for Further Research
Ongoing research is essential to keep pace with the rapid developments within the field of energy transfer. There is a growing need for studies focusing on:
- Innovative Materials: Research into materials that can better facilitate heat conduction or improve insulation.
- Data Analytics: Utilizing data to optimize energy distribution in real-time, particularly in smart grids.
- Policy Implications: Understanding the regulatory and economic frameworks necessary to support the transition to improved energy transfer solutions.