The Epigenetic Clock: Foundation and Development
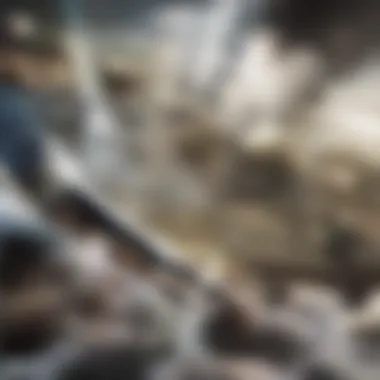
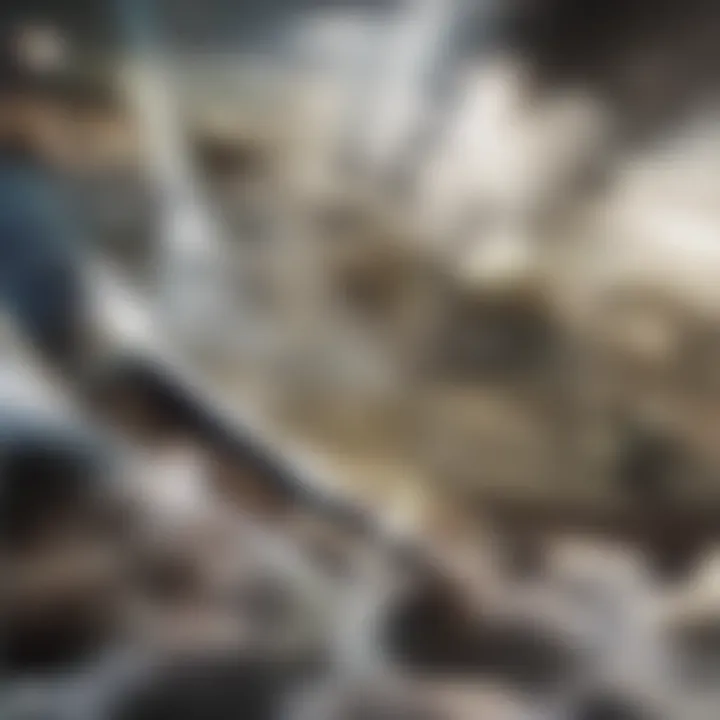
Intro
The concept of the epigenetic clock has gained prominence in the study of aging and longevity. As researchers look to understand how aging occurs at the molecular level, the role of epigenetic mechanisms becomes crucial. The epigenetic clock essentially measures the biological age of cells based on epigenetic changes, rather than chronological age. Understanding this concept can revolutionize our approach to aging-related diseases and overall health.
Article Overview
Purpose of the Article
This article aims to provide a comprehensive overview of the epigenetic clock, discussing its foundational principles and developments in the field of epigenetics. It sheds light on how epigenetic changes can influence biological aging and health outcomes. Additionally, the article explores methodologies for measuring these changes and the implications of this research for future medical applications.
Relevance to Multiple Disciplines
The topic of epigenetic aging intersects various scientific disciplines. It brings together genetics, molecular biology, and environmental science. Researchers in these fields can gain insights that may lead to breakthroughs in understanding aging and developing potential therapies. The implications stretch beyond biology to areas such as public health and social sciences, where understanding aging can inform policies on health care and aging populations.
Research Background
Historical Context
The exploration of epigenetics began with the observation of gene regulation mechanisms. Scientists identified that the environment and lifestyle choices could influence gene expression without altering the DNA sequence itself. The term "epigenetics" became widely accepted by the early 2000s, setting the foundation for further studies.
Key Concepts and Definitions
The concept of the epigenetic clock refers to a biological measure that reflects the cumulative effects of epigenetic changes over time. Key terms used in this context include:
- Epigenetic modifications: Changes that affect gene expression, such as DNA methylation and histone modification.
- Biological age: Age determined by the biological state of multiple cellular systems, as opposed to chronological age.
- DNA methylation: The addition of a methyl group to DNA, often altering gene expression.
Understanding these terms helps in grasping the significance of the epigenetic clock in biological aging.
"The epigenetic clock serves as a robust biomarker for biological aging, offering insights into cellular health and the aging process."
"The epigenetic clock serves as a robust biomarker for biological aging, offering insights into cellular health and the aging process."
This article will further explore the methodologies for measuring the epigenetic clock and the implications of such research on health and longevity.
Understanding the Epigenetic Clock
Understanding the epigenetic clock is crucial to comprehending how biological aging occurs at the molecular level. This concept integrates knowledge from various disciplines such as genetics, molecular biology, and aging research. The epigenetic clock offers insights into how environmental factors, lifestyle, and genetic predispositions affect an individual’s aging process. As we explore the epigenetic clock, we uncover foundational principles that may lead to breakthroughs in health interventions and longevity strategies.
Definition and Scope
The epigenetic clock refers to a method of estimating biological age based on epigenetic changes in the DNA. It does not merely count the years of life but focuses on biochemical modifications that accumulate over time. These modifications include DNA methylation patterns, which function as markers to assess how organs age and how external factors influence this aging process.
The scope of the epigenetic clock extends beyond merely determining age. It aims to bridge the gap between chronological age and biological age. Understanding this distinction can have significant implications for predicting disease risk, assessing overall health, and guiding medical treatments. Additionally, it provides a framework for evaluating how various interventions might reverse or slow down the aging process.
Historical Context
The concept of an epigenetic clock has evolved considerably over the past few decades. Initial studies in the field of epigenetics revealed how environmental stresses, lifestyle choices, and genes interact to influence biological outcomes. Pioneering research by scientists such as Steve Horvath laid the groundwork for establishing DNA methylation patterns as reliable indicators of aging.
In the early stages, epigenetics was primarily related to gene regulation without a clear connection to aging. As more studies emerged, researchers began linking specific epigenetic markers to aging-related phenotypes. This prompted further investigation into how these markers could be systematically measured and integrated into the broader understanding of human health and longevity.
Understanding the historical context is vital. It shows us how the epigenetic clock is not merely an abstract concept but a well-researched area that can lead to practical applications in healthcare. Furthermore, examining previous studies allows current researchers to refine methodologies and enhance the relevance of their findings, helping to advance the science of aging dramatically.
"The epigenetic clock presents a critical opportunity to address aging, enabling us to redefine how we view health and longevity."
"The epigenetic clock presents a critical opportunity to address aging, enabling us to redefine how we view health and longevity."
Through this exploration of the epigenetic clock, we prepare to delve deeper into the biological mechanisms that underpin these changes and their implications for health and disease.
Biological Mechanisms of Epigenetics
Understanding the biological mechanisms of epigenetics is crucial for comprehending how epigenetic changes influence gene expression and cellular function. This area of study sheds light on various processes that do not alter the underlying DNA sequence but still significantly impact how genes are turned on or off. The relevance of epigenetics is underscored in the context of aging, disease, and developmental biology.
The mechanisms explored here include DNA methylation, histone modifications, and non-coding RNA contributions. Each plays a distinct but interconnected role in regulating genetic expression. Delving into these mechanisms presents insights that could lead to innovative interventions in medical science and provides a framework for understanding the complexities of genetic regulation. By grasping these concepts, we can better appreciate the interplay between environment, lifestyle, and genetic predispositions.
DNA Methylation
DNA methylation involves the addition of a methyl group to DNA, typically at cytosine bases that precede a guanine, forming what is known as CpG sites. This process is essential for regulating gene expression and maintaining genomic stability. When methylation occurs at gene promoters, it often leads to gene silencing.
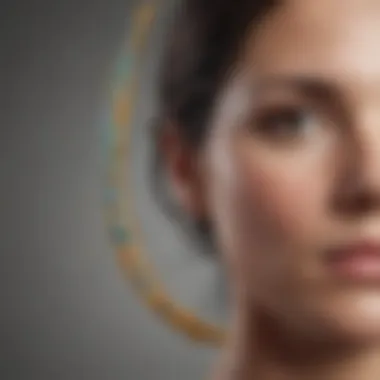
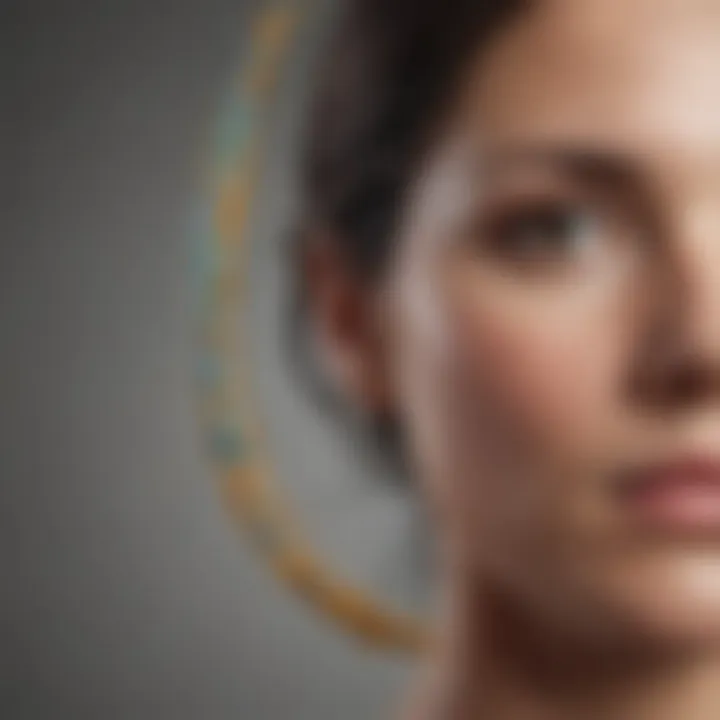
The significance of DNA methylation extends beyond mere gene regulation. It plays a pivotal role in cellular differentiation and development. For instance, in embryonic development, specific genes must be silenced or activated at the right times, influencing cell fate decisions.
Moreover, changes in DNA methylation patterns have been associated with various diseases, particularly cancers. Uncontrolled cell growth can result from aberrant methylation, highlighting the importance of this mechanism in disease pathology. Tracking methylation changes can serve as a biomarker for aging and disease progression, providing valuable insights for researchers.
Histone Modification
Histones are proteins that package and order DNA into structural units called nucleosomes. They are subject to various chemical modifications, such as acetylation, methylation, phosphorylation, and ubiquitination. These modifications can alter the chromatin structure, influencing gene accessibility and expression levels.
Acetylation, for instance, typically results in gene activation, as it relaxes the DNA-histone interaction, making the DNA more accessible. Conversely, some forms of histone methylation can lead to gene repression, depending on the context and the specific residue that is modified.
The implications of histone modifications are profound. They are involved in processes such as DNA repair, cell cycle regulation, and response to environmental stressors. Studying these modifications provides insights into how cells adapt to changes and contributes significantly to understanding diseases linked to epigenetic dysregulation.
Non-coding RNA
Non-coding RNAs, which do not translate into proteins, have emerged as key regulators of gene expression. They are involved in various cellular processes, including chromatin remodeling, transcriptional regulation, and post-transcriptional modifications. Two notable classes are microRNAs and long non-coding RNAs (lncRNAs).
MicroRNAs bind to complementary mRNA sequences, leading to mRNA degradation or inhibition of translation. This interaction ensures that gene expression is finely tuned. Long non-coding RNAs can interact with chromatin and transcription machinery, influencing gene expression patterns in more complex ways.
The study of non-coding RNAs has revealed their potential as therapeutic targets. Understanding how these molecules modulate gene expression can illuminate pathways involved in aging and diseases, potentially guiding new treatment approaches.
"The biological mechanisms of epigenetics form a complex and intricate system that continues to unveil the layers of gene regulation and its implications in health and disease."
"The biological mechanisms of epigenetics form a complex and intricate system that continues to unveil the layers of gene regulation and its implications in health and disease."
In summary, the biological mechanisms of epigenetics, particularly DNA methylation, histone modifications, and non-coding RNAs, are fundamental to gene expression regulation. These processes not only shape development and cell phenotype but are also critical for understanding the etiology of diseases. Their relevance in aging research further elevates their importance, demonstrating the need for continued investigation in this dynamic field.
Development of the Epigenetic Clock
The development of the epigenetic clock is a critical area of focus within the broader context of aging research. This clock offers a mechanism to assess biological age, which may differ from chronological age. Understanding this area can lead to significant advancements in healthspan and lifespan interventions. Recognizing the importance of the epigenetic clock in aging also prompts discussions on preventive healthcare measures and therapeutics.
Key Research Milestones
Several key research milestones have shaped the understanding of the epigenetic clock. Key discoveries include the identification of specific epigenetic markers associated with aging, such as DNA methylation patterns. In 2013, researchers like Andrew Feinberg and Steve Horvath introduced a well-defined biological clock based on DNA methylation levels. This innovation allowed for the first time a reliable estimate of biological aging using a relatively simple blood test.
Research has continued to evolve. Large cohort studies have validated these findings. For example, the Horvath's clock, which analyzes 353 specific CpG sites, has been extensively used in different studies to correlate biological age with age-related diseases.
Another milestone was the recognition that various environmental factors can influence epigenetic changes over time, not just genetic inheritance. This interplay between genetics and environment has further enriched the concept of the clock, making it a powerful tool for future agin research and public health interventions.
Innovative Methodologies
The methodologies employed in the development of the epigenetic clock are varied and innovative. High-throughput sequencing technologies form a backbone for many studies. These technologies allow for the comprehensive analysis of DNA methylation patterns across the genome.
One such technique is bisulfite sequencing. This method enables scientists to determine methylation status at single-base resolution. It provides a deep understanding of how specific genes are regulated over time and how their regulation may contribute to aging.
Another innovative approach involves machine learning algorithms. By analyzing vast amounts of epigenetic data, these algorithms can identify patterns that might not be apparent through traditional statistical methods. This enhances not only the accuracy of biological age estimations but also improves the prediction of age-related health outcomes.
Data integration from different sources, including genomics, transcriptomics, and proteomics, also represents an emerging trend in the field. This interdisciplinary approach promises to provide a more holistic understanding of the aging process, paving the way for targeted therapies and interventions.
"The epigenetic clock stands at the intersection of genetics and environmental influence, marking a significant accomplishment in precision medicine."
"The epigenetic clock stands at the intersection of genetics and environmental influence, marking a significant accomplishment in precision medicine."
In summary, the development of the epigenetic clock is essential for understanding aging from both a biological and practical perspective. It embraces the dynamic nature of epigenetics and underscores the necessity of integrative research approaches.
Measuring the Epigenetic Clock
Understanding how to measure the epigenetic clock is crucial for research in aging and longevity. The mechanisms of epigenetic changes play a pivotal role in biological processes. These measurements allow scientists to assess biological age and make predictions about life expectancy, which is vital for various applications in health and medicine. By systematically measuring epigenetic marks, researchers are uncovering how the environment and lifestyle influence aging pathways.
As science progresses, precise measurement techniques become increasingly important. Such techniques not only improve our understanding of aging but also inform clinical strategies targeting age-related diseases. Employing advanced techniques minimizes biases, ensuring that data analysis is robust and reproducible.
Techniques for Measurement
Multiple methodologies exist for measuring the epigenetic clock, with each offering distinct advantages. One widely used technique is DNA methylation analysis. This method examines specific cytosine bases in DNA to determine methylation patterns. These patterns have been closely linked with biological age. The two common approaches in this area involve targeted sequencing and array-based methods.
Another important technique is the use of bisulfite sequencing. In this process, DNA is treated with bisulfite, converting unmethylated cytosines into uracils while leaving methylated cytosines unchanged. This method enables precise determination of methylation levels, providing insight into the aging process.
We also have mass spectrometry. This technique quantifies post-translational modifications of histones, revealing crucial information about epigenetic changes associated with aging. Histone profiling has shown potential in identifying age-related modifications that differentiate old samples from young ones.
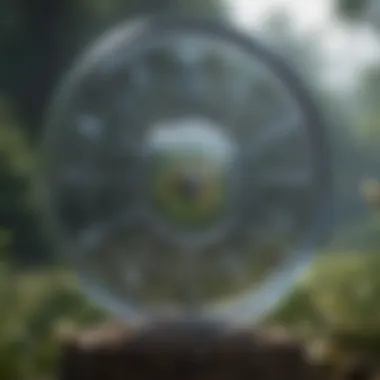
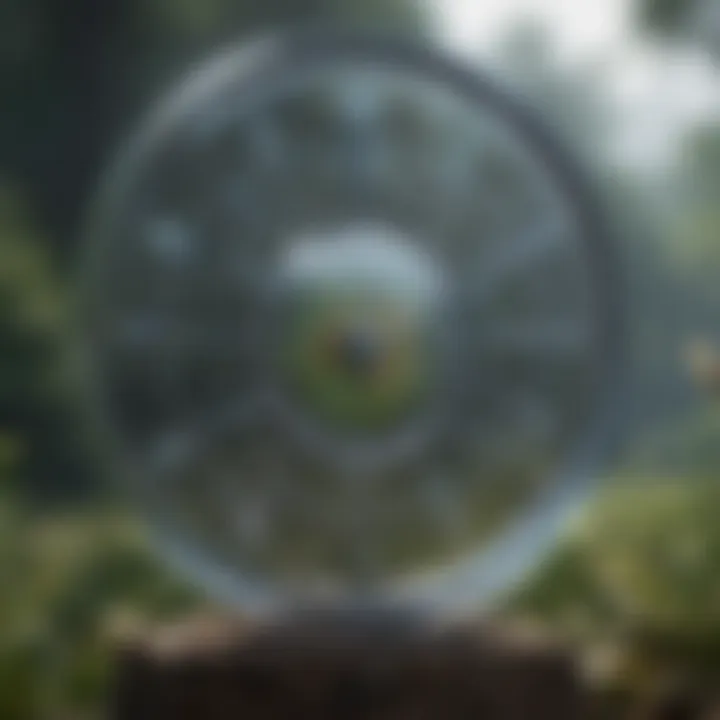
Moreover, emerging technologies such as single-cell epigenomics are becoming impactful. This allows the evaluation of epigenetic alterations at a single-cell resolution, paving the way for personalized medicine approaches in aging.
Data Analysis Approaches
Effective analysis of data derived from epigenetic clock measurements necessitates a systematic approach. Various computational tools have been developed to handle the large volumes of data generated from high-throughput sequencing methods. These tools allow researchers to identify significant changes in methylation patterns linked to age and health conditions.
One popular method for data analysis is machine learning. Algorithms can be trained to recognize age-related methylation patterns, offering predictions on biological age based on a sample's methylation profile. These predictions can, in some instances, outperform traditional biological markers of age.
Another approach is statistical modeling. Models help in understanding the relationships between various epigenetic markers and biological aging. Techniques like linear regression or mixed-effects models can uncover associations that are not immediately apparent.
"As research progresses, robust data analysis will play a crucial role in validating findings related to the epigenetic clock."
"As research progresses, robust data analysis will play a crucial role in validating findings related to the epigenetic clock."
Furthermore, integrating multi-omics data enhances analytical insights. By considering genomic, transcriptomic, and epigenomic information collectively, researchers can build a more comprehensive understanding of aging processes. This convergence is crucial for advancing personalized health interventions and exploring potential therapeutic targets.
Implications of the Epigenetic Clock
The implications of the epigenetic clock extend far beyond a mere understanding of biological aging. This concept, which quantifies the biological age of an organism based on epigenetic changes, offers profound insights into how we approach health, wellness, and longevity. It not only aids in the comprehension of the aging process but also highlights potential interventions that could alter our life trajectories in significant ways. Below are key areas where the implications of the epigenetic clock are particularly noteworthy.
Understanding Biological Aging
Biological aging is a complex process influenced by genetic and environmental factors. The epigenetic clock serves as a reliable measure of this process, as it reflects changes in DNA methylation patterns that occur over time. Research in this area reveals several fundamental aspects of aging:
- Biomarker of Aging: The epigenetic clock acts as a biomarker for aging, providing a quantifiable metric that can differentiate between chronological age and biological age. This distinction is crucial for understanding health risks associated with aging-related diseases.
- Predictive Power: The ability of the epigenetic clock to predict various health outcomes, including susceptibility to diseases and overall lifespan, is a major area of research. Studies show that individuals with a biological age that is significantly older than their chronological age may face increased risks for chronic diseases such as heart disease, diabetes, and neurodegenerative conditions.
- Mechanisms of Aging: The insights gained from studying the epigenetic clock shed light on the underlying biological mechanisms of aging. For instance, specific patterns of DNA methylation have been linked to key pathways involved in cellular repair and regeneration.
"Understanding biological aging through the lens of the epigenetic clock revolutionizes our perspective on health and disease in later life."
"Understanding biological aging through the lens of the epigenetic clock revolutionizes our perspective on health and disease in later life."
Potential for Longevity Interventions
The implications of the epigenetic clock also suggest promising avenues for longevity interventions. As researchers continue to unveil the relationships between epigenetic changes and lifestyle choices, several potential strategies have emerged:
- Lifestyle Modifications: Interventions such as diet and exercise have shown the potential to positively impact the epigenetic clock. Incorporating regular physical activity and a balanced diet rich in antioxidants may slow down biological aging.
- Pharmacological Interventions: Emerging studies explore the potential impact of certain drugs that target epigenetic mechanisms. For example, compounds like resveratrol, found in red wine, have been suggested to promote longevity by influencing DNA methylation processes.
- Personalized Medicine: Advances in epigenetic research may pave the way for personalized medical approaches. By understanding an individual's epigenetic profile, healthcare providers could tailor interventions to optimize health outcomes and slow biological aging.
In summary, the implications of the epigenetic clock present both a profound understanding of biological aging and a framework for potential interventions that enhance longevity. This domain holds considerable promise for advancing medical science and personal health strategies, making it a focal point for future research.
Environmental Influences on the Epigenetic Clock
Understanding the environmental influences on the epigenetic clock is critical. These factors provide insights into how external elements can modify gene expression and biological aging. This section investigates how lifestyle choices and stressors impact the intricate mechanisms of epigenetics. Such knowledge may lead to improved applications in medicine and public health.
Role of Lifestyle Factors
Lifestyle choices are pivotal in the modulation of the epigenetic clock. Diet, exercise, and sleep patterns can cause significant epigenetic changes and influence health outcomes.
- Nutrition: A balanced diet rich in nutrients is linked to favorable epigenetic changes. For instance, diets high in folate, found in leafy greens, may promote DNA methylation processes.
- Physical Activity: Regular exercise reduces the risk of chronic diseases and has been associated with reversible epigenetic alterations. This shows how movements can impact gene expression positively.
- Sleep: Quality sleep is essential for maintaining healthy epigenetic regulation. Sleep deprivation can result in dysregulation of genes involved in metabolism and stress response.
Lifestyle factors contribute not only to an individual's health but also to the biological aging process. Therefore, adapting healthier habits could potentially delay the progression of age-related diseases.
Impact of Stressors
Stressors, both psychological and environmental, significantly influence the epigenetic clock. They induce alterations in DNA methylation and histone modification, which can have profound effects on health.
- Psychological Stress: Chronic stress has been shown to alter the expression of genes related to inflammation and immune response. Elevated cortisol levels can lead to adverse epigenetic changes, potentially accelerating aging.
- Environmental Toxins: Exposure to pollutants and chemicals can modify epigenetic markers. Substances like heavy metals and endocrine disruptors may induce detrimental epigenetic modifications, influencing various diseases.
- Social Factors: Socioeconomic status and social support are also linked to stress levels and overall health. Those with higher stress levels might experience accelerated aging, as indicated by epigenetic clocks.
In summary, both lifestyle factors and stressors are crucial in shaping the epigenetic landscape. Addressing these influences can lead to strategies aimed at improving health and longevity.
"Understanding the environmental factors affecting the epigenetic clock opens pathways for interventions that could enhance quality of life and longevity."
"Understanding the environmental factors affecting the epigenetic clock opens pathways for interventions that could enhance quality of life and longevity."
By recognizing the interplay between the environment and epigenetic mechanisms, researchers can develop targeted approaches for healthier living, potentially offering new avenues in longevity research.
Epigenetics in Disease Context
Epigenetics plays a crucial role in understanding various diseases, providing insights into how genetic expression can be altered without changing the underlying DNA sequence. This section will explore the significance of epigenetic mechanisms in the context of disease, focusing on their implication in aging-related diseases and cancer. These insights drive research and foster the development of novel therapeutic strategies.
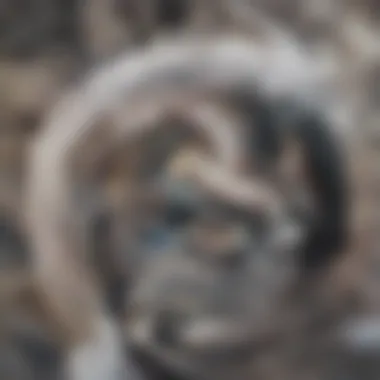

Aging-Related Diseases
Aging-related diseases, such as Alzheimer's, Parkinson's, and cardiovascular ailments, showcase the intricate relationship between epigenetic changes and aging. As individuals age, their epigenetic profiles undergo modifications that can contribute to disease emergence. For instance, DNA methylation patterns can shift with age, which affects gene expression linked to inflammation and cellular repair.
Key components to consider in this context include:
- DNA Methylation Changes: Research shows that increased methylation at certain gene promoters is linked with the onset of age-related diseases. For example, genes involved in immune responses may be hypo-methylated in older adults, contributing to diminished immune function.
- Histone Modifications: Changes in histone proteins can affect chromatin structure. Altered histone acetylation and methylation patterns are observed in aging tissues and are correlated with various aging-related diseases.
- Epigenetic Biomarkers: Identifying epigenetic markers can help predict susceptibility to these diseases. For instance, specific methylation patterns could serve as predictive markers for Alzheimer's disease.
These points underline the importance of studying epigenetics in the context of aging-related diseases as they may inform preventative measures and therapeutic interventions.
Cancer and Epigenetic Changes
The connection between cancer and epigenetic changes is profound. Tumorigenesis can often be traced back to aberrant epigenetic modifications that lead to uncontrolled cell growth and division. Cancer cells frequently exhibit a unique epigenetic landscape compared to normal cells, which significantly contributes to their behavior.
Significant aspects include:
- Gene Silencing via Methylation: In many cancers, tumor suppressor genes are silenced due to hypermethylation. This silencing removes critical growth inhibitory signals that would typically prevent tumor expansion.
- Histone Alterations: Alterations in histone acetylation levels can lead to gene expression changes that promote cancer cell growth. For instance, increased acetylation generally correlates with gene activation, potentially leading to oncogenic processes when the genes activated are those that promote tumor growth.
- Targeting Epigenetic Modifications: There is growing interest in developing epigenetic therapies, such as inhibitors targeting DNA methyltransferases or histone deacetylases. Clinical studies show that these interventions have the potential to restore normal gene expression patterns in cancer cells.
"Understanding the interplay between epigenetic modifications and disease processes opens new avenues for therapeutic interventions that could vastly improve patient outcomes."
"Understanding the interplay between epigenetic modifications and disease processes opens new avenues for therapeutic interventions that could vastly improve patient outcomes."
In summary, investigating the role of epigenetics in the context of diseases, especially those related to aging and cancer, is vital. This understanding can lead to the development of targeted therapies and contribute to personalized medicine approaches in treatment.
Future Directions in Epigenetic Research
The field of epigenetics continues to evolve dramatically, with the epigenetic clock at its forefront. Understanding future directions in epigenetic research is crucial. It holds immense potential for deepening our insights into biological aging, disease mechanisms, and therapeutic strategies. Researchers are increasingly focusing on the integration of new technologies and collaborations across diverse scientific disciplines.
Emerging Technologies
Emerging technologies greatly enhance the capabilities of epigenetic studies. These technologies are crucial for refining the measurement of epigenetic modifications and understanding their implications.
- Next-Generation Sequencing (NGS): This technology has transformed the ability to analyze epigenetic landscapes. NGS provides data on DNA methylation patterns with unprecedented resolution. It allows for the analysis of many samples simultaneously, leading to robust conclusions about epigenetic changes.
- Single-cell Epigenomics: This approach permits the examination of epigenetic variations at the single-cell level. It reveals heterogeneity among cells, which is essential for understanding complex traits such as aging and disease.
- CRISPR Technology: The CRISPR-Cas9 system's application in epigenetics offers the potential for targeted epigenetic editing. Its ability to specifically modify gene expression opens pathways for new therapies targeting aging-related diseases and other conditions.
- Bioinformatics: Advanced computational tools are critical for interpreting large datasets in epigenetic research. Machine learning algorithms can uncover patterns and predict biological outcomes, enhancing the understanding of epigenetic mechanisms.
These technologies are not only important for academic research but can also lead to practical applications in medicine and health.
Interdisciplinary Approaches
The future of epigenetic research demands interdisciplinary approaches. Collaboration between various fields fosters innovation and helps address complex questions.
- Collaboration with Clinical Researchers: To translate findings into practice, epigeneticists should work alongside clinicians. This partnership can help develop targeted therapies and preventive strategies.
- Integration with Environmental Sciences: Studying how environmental factors interact with epigenetic mechanisms is vital. Knowledge from environmental science can inform public health policies and strategies to mitigate adverse effects of lifestyle choices.
- Social Sciences: Understanding the epigenetic clock also requires insights from social sciences. Researchers can examine how social determinants influence health and contribute to epigenetic changes over a lifespan.
"Collaboration across disciplines is key to unlocking the potential of epigenetic research and translating knowledge into actionable insights."
"Collaboration across disciplines is key to unlocking the potential of epigenetic research and translating knowledge into actionable insights."
This collaborative ethos will strengthen the field and pave the way for groundbreaking research. It will enable scientists to address the multifaceted nature of aging and its related diseases more effectively.
In summary, the future direction of epigenetic research is promising. With emerging technologies and interdisciplinary collaborations, significant strides will be made in understanding the epigenetic clock. These developments could lead to innovative solutions that impact health and longevity.
Ethical Considerations
Ethical considerations are pivotal in the discourse surrounding the epigenetic clock. As this field advances, understanding the implications of epigenetic research on society and individual lives is essential. This section evaluates the relevance of ethical principles as they pertain to research ethics and societal impacts.
Research Ethics
Research in epigenetics demands careful ethical scrutiny. The complexities of manipulating genetic information raise questions about consent, privacy, and the potential for misuse. Ethical frameworks must ensure that participants in studies are fully informed about the risks and benefits associated with their involvement.
Additionally, the collection and analysis of genetic data can lead to privacy concerns. Researchers need to implement robust measures to protect personal information. The potential for discrimination based on genetic information is another concern that has surfaced, necessitating legal protections to prevent misuse by employers or insurance companies.
"Understanding the ethical landscape is as important as scientific discovery itself."
"Understanding the ethical landscape is as important as scientific discovery itself."
Moreover, as epigenetic research evolves, scenarios may arise where genetic modifications are possible. This reality compels a conversation about the moral implications of such technology. Should society pursue enhancements to human capabilities? Striking a balance between scientific progress and ethical responsibility is crucial.
Implications for Society
The societal implications of epigenetic research extend far beyond the laboratory. Advancements in this field can pave the way for innovative medical treatments and preventive measures against diseases. However, they also present challenges that must be addressed.
- Equity in Access: As new therapies emerge, it is vital to ensure that they are accessible to diverse populations. Disparities in healthcare access may worsen if only privileged groups can benefit from these advances.
- Informed Decision-Making: Society must be educated about the implications of epigenetics. Individuals should be empowered to make informed decisions about their health, understanding risks and benefits of genetic testing and potential interventions.
- Regulatory Oversight: As epigenetic research intertwines with medical applications, effective regulation becomes essential. Policies must evolve to guide ethical research and clinical applications while safeguarding public interest.
Navigating these ethical waters will be fundamental as the epigenetic clock’s insights translate into real-world applications. Ensuring that scientific advancement aligns with ethical principles can foster trust in research and its outcomes.