Exploring 3D Accelerometers and Their Diverse Uses

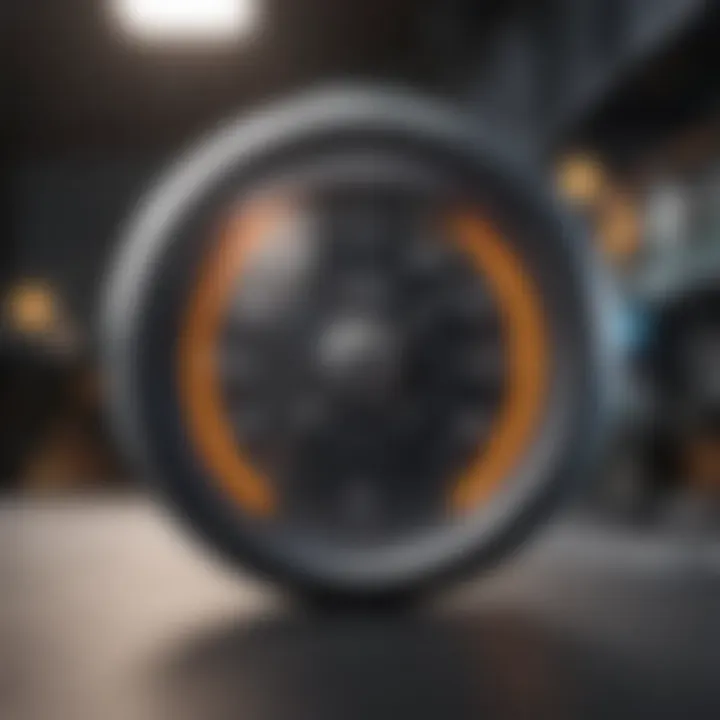
Article Overview
Purpose of the Article
This article seeks to comprehensively examine 3D accelerometers, detailing their operational principles and the various domains in which they are applied. By analyzing how these devices measure acceleration across three dimensions, this work aims to provide clarity about their functionality and significance. An understanding of 3D accelerometers is not only relevant to engineers and developers but also to educators, researchers, and students who aspire to grasp the impact of these technologies on modern innovation.
Relevance to Multiple Disciplines
3D accelerometers play a critical role in several fields:
- Consumer Electronics: These devices are widely used in smartphones, tablets, and gaming consoles to enhance user experience by detecting orientation and motion.
- Automotive Safety: In vehicles, 3D accelerometers contribute to safety systems, including airbag deployment and vehicle stability control.
- Robotics: In robotics, they enhance navigation and balance, allowing robots to interact with dynamic environments effectively.
- Healthcare: In medical applications, they assist in movement analysis, rehabilitation, and monitoring patient activity.
The versatility of 3D accelerometers across these sectors illustrates their importance in advancing technologies and improving operational efficiencies, refining user experiences, and fostering innovations.
Research Background
Historical Context
The development of accelerometers has come a long way since their inception. Originally, accelerometers were mechanical devices predominantly used in aerospace and military applications. With advancements in technology, miniaturization has allowed the integration of solid-state sensors in consumer products. The evolution to 3D accelerometers represents a significant advancement in how motion and orientation can be effectively measured and analyzed.
Key Concepts and Definitions
To better understand 3D accelerometers, itโs important to define some key concepts:
- Acceleration: Defined as the rate of change of velocity of an object.
- Axes: In 3D accelerometers, the three axes (X, Y, and Z) represent the three dimensions in which acceleration is measured. The X-axis code for side-to-side movement, the Y-axis measures forward and backward, and the Z-axis tracks up and down.
- Calibration: A process that involves adjusting the accelerometer to ensure accurate measurements, especially in varying conditions.
"Understanding the principles behind 3D accelerometers is essential for those looking to engage in fields reliant on motion detection technology."
"Understanding the principles behind 3D accelerometers is essential for those looking to engage in fields reliant on motion detection technology."
This exploration into the workings and challenges of 3D accelerometers will elucidate their technological landscape and clarify their potential as we advance into the future.
Intro to 3D Accelerometers
3D accelerometers serve as pivotal instruments in understanding motion. By measuring acceleration across three orthogonal axes, they play an essential role in various industries, including consumer electronics, automotive systems, healthcare, and robotics. The importance of diving into this topic lies in the pervasive application of these sensors in todayโs technology-driven world. Their ability to accurately detect changes in velocity makes them indispensable for functionality in modern devices.
Definition and Functionality
A 3D accelerometer is a sensor designed to detect acceleration along three different axes: X, Y, and Z. This capability allows it to measure the speed and direction of movement. The primary function involves converting inertial forces into readable electrical signals. As a result, devices can interpret physical movements and respond accordingly. For example, smartphones utilize accelerometers to adjust screen orientation based on how a user tilts or moves the device. This innovation enhances user interaction and offers a more dynamic experience.
Historical Development
The evolution of accelerometers traces back several decades. Initially, sensors were bulky, resembling mechanical devices that were limited in application. However, with advances in technology, particularly in microelectromechanical systems (MEMS), these sensors have become considerably more compact and efficient.
In the late 20th century, as the demand for portable electronic devices grew, the need for smaller and more accurate accelerometers surged. Companies began investing in research and development, leading to significant breakthroughs. Todayโs accelerometers are not only smaller but also offer higher precision and improved data processing capabilities.
The shift towards digital technology further propelled their incorporation into mainstream products, from gaming consoles to automotive safety systems. This development reflects a broader trend in engineering and technology, where the integration of sensors plays a crucial role in enhancing functionality.
"The evolution of 3D accelerometers illustrates a remarkable journey of innovation, transforming bulky mechanical systems into sophisticated electronic sensors that drive contemporary technology forward."
"The evolution of 3D accelerometers illustrates a remarkable journey of innovation, transforming bulky mechanical systems into sophisticated electronic sensors that drive contemporary technology forward."

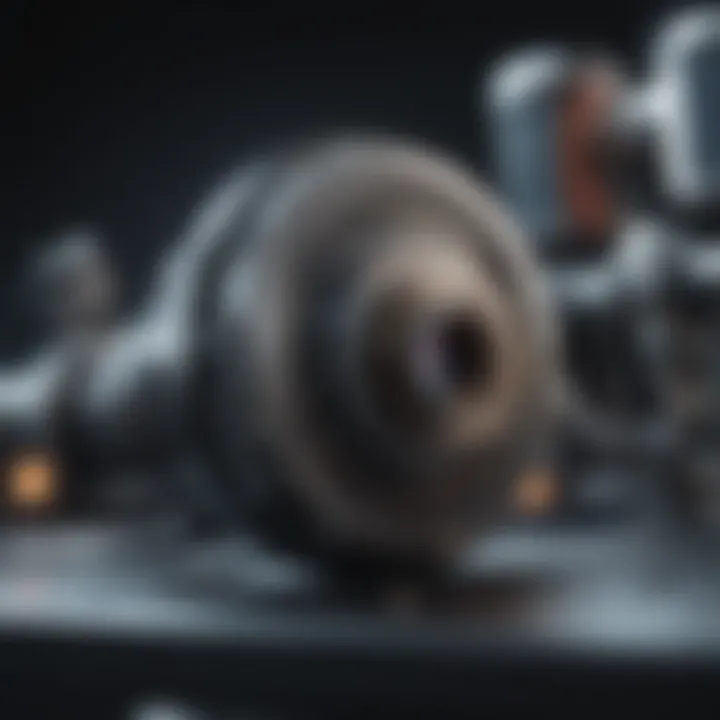
As we explore further, understanding these foundational aspects of 3D accelerometers lays the groundwork for appreciating their applications and significance in various domains.
Principles of Operation
Understanding the principles of operation for 3D accelerometers is crucial. These principles encompass how the sensors measure acceleration, as well as the various measurement techniques implemented. Knowing these fundamental aspects can help in evaluating the effectiveness and accuracy of these devices in different applications.
Sensor Types
Different types of accelerometers serve unique purposes. The main categories are capacitive, piezoelectric, and MEMS accelerometers. Each type has distinct operational mechanisms that contribute to their effectiveness in specific applications.
Capacitive Accelerometers
Capacitive accelerometers operate based on variations in capacitance. They typically utilize two conductive plates that change distance relative to motion. The main characteristic of these sensors is their high sensitivity. This makes them a popular choice in applications requiring precise measurements. A unique feature is the ability to detect low-frequency vibrations effectively. However, they may struggle with extremely high-frequency signals or environments with significant electromagnetic interference.
Piezoelectric Accelerometers
Piezoelectric accelerometers function by generating charge in response to mechanical stress. Their key characteristic is the ability to measure dynamic acceleration over a broad frequency range. This makes them ideal for environments that experience rapid movements, like aerospace or automotive applications. The unique aspect of piezoelectric sensors is their robustness against thermal variations. However, they can be less effective for static measurements, which limits their use in applications requiring constant acceleration readings.
MEMS Accelerometers
MEMS (Micro-Electro-Mechanical Systems) accelerometers are compact and highly prevalent in everyday devices. They use microstructures to detect changes in acceleration. The primary advantage of MEMS sensors is their small size and low cost. This characteristic supports their extensive use in consumer electronics. A unique feature is their ability to integrate with other sensors, enabling versatile applications. However, MEMS accelerometers can have lower accuracy than larger, metric counterparts, which might be relevant in precision applications.
Measurement Techniques
To effectively utilize the data from 3D accelerometers, understanding different measurement techniques is necessary. These techniques are crucial for interpreting how accelerations are recorded in various situations.
Static and Dynamic Measurements
Static measurements refer to the evaluation of acceleration when an object is not in motion. Dynamic measurements occur when an object is accelerating. This duality is essential because it helps in distinguishing static states from movement-induced accelerations. The main characteristic of this technique is its versatility in applications ranging from simple motion detection in smartphones to complex data gathering in aerospace engineering. A key feature is its ability to provide real-time data on object status, thus contributing significantly to safety and performance.
Integration of Gyroscopes
The integration of gyroscopes with accelerometers can enhance measurement accuracy. Gyroscopes can provide information about orientation, helping to mitigate the effects of drift that might occur in accelerometers alone. This hybrid setup is particularly beneficial in robotics and automotive safety applications. A prominent characteristic is the comprehensive data that allows more complex calculations of motion, benefiting overall system performance. However, the added complexity and cost of integrating these technologies can be a drawback in simpler applications.
Data Processing
The precise processing of acceleration data is vital for meaningful interpretation and application. Synchronous sampling and noise filtering algorithms play key roles in refining the data output.
Synchronous Sampling
Synchronous sampling occurs when multiple outputs are sampled at the same time, ensuring that all data points are time-coherent. This method is important for accurate representation of dynamic systems and is widely used in real-time applications. Its key characteristic is that it helps in reducing data inconsistencies. A unique advantage is improved precision in multi-channel systems, but this requires more sophisticated data acquisition systems, which can increase costs.
Noise Filtering Algorithms
Noise filtering algorithms are used to improve the accuracy of the collected data by minimizing the effects of unwanted disturbances. These algorithms are essential for producing clean signals, which is particularly critical in environments with high background noise. The main advantage is the significant enhancement of the signal-to-noise ratio, which helps in obtaining reliable data. However, designing these algorithms can be complex and might introduce computational delays, affecting real-time analysis.
"The choice of sensor type and measurement method greatly influences the performance and application of 3D accelerometers."
"The choice of sensor type and measurement method greatly influences the performance and application of 3D accelerometers."
In summary, the principles of operation for 3D accelerometers determine their effectiveness across various applications. Understanding sensor types and measurement techniques is essential for evaluating their role in innovative technologies.
Key Applications of 3D Accelerometers
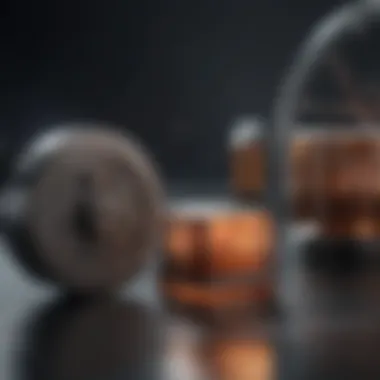
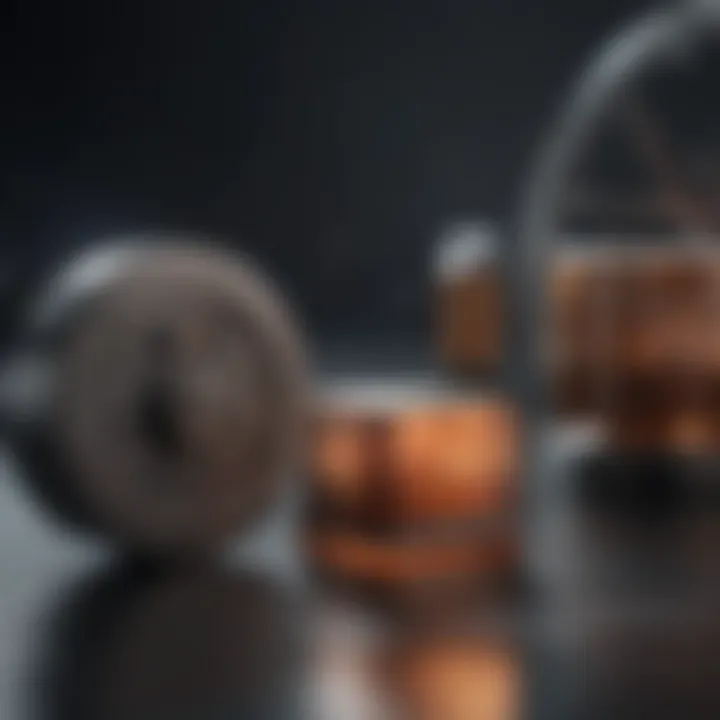
The key applications of 3D accelerometers are critical in understanding how these devices innovate various fields. Their importance spans from enhancing user experience in consumer electronics to increasing safety in automotive systems. Furthermore, they offer advancements in healthcare monitoring and enhance capabilities in robotics. This section will discuss these applications in detail, focusing on the specific elements that showcase the benefits and considerations of 3D accelerometers.
Consumer Electronics
Smartphones and Tablets
Smartphones and tablets leverage 3D accelerometers primarily for motion detection and orientation purposes. These devices make use of accelerometers to automatically adjust screen orientation, turn navigation maps, and enhance gaming experiences. This capability is a cornerstone feature of most modern smartphones. The key characteristic here lies in real-time responsiveness, which provides users with a seamless experience while using applications. However, the reliance on these sensors can expose users to issues such as battery drain due to continuous tracking.
Gaming Devices
Gaming devices, such as motion-sensitive controllers, often use 3D accelerometers to create engaging gameplay experiences. The accelerometer detects subtle motions, allowing players to interact with games more intuitively. A significant advantage of this system is its ability to enhance immersion through physical movement. Nevertheless, the technology can struggle with high-speed motions, leading to inaccuracies that may disrupt gameplay.
Automotive Safety Systems
Airbag Deployment
In automotive safety, 3D accelerometers play a vital role in airbag deployment systems. These sensors detect sudden deceleration or changes in motion that suggest a collision. The main benefit is the ability to enable airbags to activate in the most critical moments, thus enhancing occupant safety. A challenge, however, is ensuring that false positives do not trigger airbags in non-collision situations, which could lead to injury.
Stability Control
Stability control systems utilize 3D accelerometers to improve vehicle handling and prevent skidding. The sensors monitor the vehicle's motion and provide data that assists in making split-second adjustments to braking and power delivery. This system's hallmark advantage is its ability to keep drivers and passengers safe during adverse driving conditions. On the downside, precision calibration is essential; inaccurate calibration can lead to failures in response during emergencies.
Healthcare Monitoring
Fall Detection Systems
In healthcare, fall detection systems incorporate 3D accelerometers for monitoring patients, particularly the elderly. These systems can detect falls accurately and trigger alerts for immediate assistance. The key characteristic of this application is timely response, which can be lifesaving. Nonetheless, false alarms can occur, leading to unnecessary interventions and distress.
Patient Activity Tracking
Patient activity tracking systems utilize 3D accelerometers to monitor daily movements and health metrics. These systems allow caregivers to gauge patient activity levels and general well-being. This capability is crucial for proactive healthcare management and rehabilitation. However, data privacy concerns arise, as sensitive health information may be collected and shared inadvertently.
Robotics and Automation
Navigation and Control
Robotics and automation entities rely on 3D accelerometers for navigation and control. The sensors provide real-time feedback about the robot's orientation and position relative to its environment. Over time, this enhances the precision of movements, which is crucial for tasks ranging from simple navigation to complex industrial operations. The tradeoff may involve higher computational demands, as processing real-time data can become resource-intensive.
Gesture Recognition
Gesture recognition technologies use 3D accelerometers to interpret user gestures and commands without physical input devices. This application succeeds in providing a more interactive and hands-free experience. The main benefit of this technology is the enhanced accessibility it offers, but the complexity of accurately interpreting gestures can sometimes limit performance in specific environments.
Challenges and Limitations
Understanding the challenges and limitations of 3D accelerometers is crucial for their optimal implementation across various fields. Despite their numerous applications, these sensors face specific hurdles that can affect their accuracy and reliability. Recognizing these limitations allows developers and engineers to address potential pitfalls, enhancing both the design and functionality of devices that depend on these sensors.
Accuracy and Precision
Accuracy and precision are essential in assessing the performance of 3D accelerometers. Accuracy refers to the degree to which a measurement reflects the true value, while precision indicates the consistency of repeated measurements. For instance, in automotive safety systems, a slight deviation in measurement could lead to critical failures during airbag deployment. Therefore, achieving high accuracy and precision is necessary to ensure the reliability of 3D accelerometers in sensitive applications. The calibration process and the choice of sensor type can significantly influence these factors.
Calibration Techniques
The calibration of 3D accelerometers is vital for maintaining their performance over time. Without proper calibration, sensors can exhibit drift, leading to inaccuracies. Techniques for calibration often involve comparing the accelerometer's readings against a known reference. Common methods include static calibration, where the device is subjected to gravitational acceleration, and dynamic calibration, where known accelerations are applied. Employing systematic calibration methodologies helps ensure the accurate functioning of accelerometers, particularly in high-stakes environments such as healthcare monitoring and automotive systems.
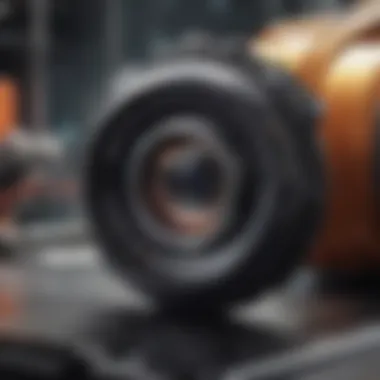
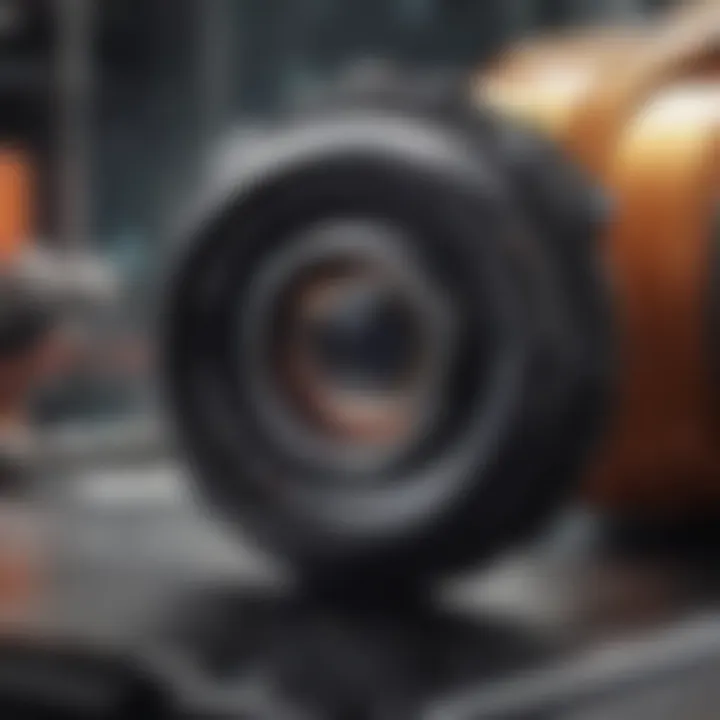
Environmental Factors
Environmental factors play a significant role in the performance of 3D accelerometers. Two main sub-factors are temperature variations and vibration effects.
Temperature Variations
Temperature variations can greatly influence the readings of 3D accelerometers. This effect is particularly pronounced in sensitive applications where precision is paramount. Changes in temperature can affect the material properties of the sensor components, leading to shifts in output signals. Understanding this aspect is essential for designing systems that can compensate for these variations. For example, compensating for temperature changes can enhance the reliability of medical monitoring devices used in varying conditions.
Vibration Effects
Vibration effects also present challenges to the performance of 3D accelerometers, especially in dynamic environments. External vibrations can induce noise in the sensor readings, making it difficult to obtain accurate data. This noise can lead to fluctuations in the output, creating challenges in contexts like robotics and automotive safety systems. Identifying the frequency and amplitude of vibration can help in designing filtering algorithms that effectively mitigate these effects. Thus, understanding how vibration influences performance is crucial for ensuring the integrity of measurements across various applications.
Future Trends and Innovations
The domain of 3D accelerometers is evolving rapidly. Various advancements are shaping the future landscape of this technology. Users are increasingly integrating these sensors into a diverse range of applications. These trends reflect not just technological evolution, but also real-world demands for accuracy, efficiency, and new functionalities.
Integration with IoT
The integration of 3D accelerometers with the Internet of Things (IoT) marks a significant transformation in how data is gathered and utilized. IoT-enabled accelerometers can connect to cloud platforms for real-time data analysis. This connectivity allows devices to share information instantaneously.
In applications like smart homes or smart cities, 3D accelerometers can monitor and optimize energy use. For instance, they can detect movements and adjust lighting or temperature accordingly. This automatic adjusting promotes energy efficiency, a pressing concern in contemporary society. Thus, integrating with IoT is not just about connectivity; it is about creating intelligent environments.
Advancements in Miniaturization
Miniaturization is another crucial trend. The ongoing reduction in size without compromising performance is vital for various applications, especially in consumer electronics. As devices become smaller and lighter, the role of compact 3D accelerometers grows. Devices like wearables and smart gadgets can now afford to integrate high-performance sensors seamlessly.
Manufacturers are also focusing on cost-effectiveness in miniaturization. This trend leads to greater accessibility for small-scale applications, from fitness trackers to drones.
Emerging Materials and Technology
Research into emerging materials is driving the next wave of innovations in 3D accelerometers. For example, utilizing flexible materials can create sensors that are not only lightweight but also durable. This flexibility may enable new applications, such as integration into clothing or medical devices. Furthermore, advancements in nanotechnology give rise to sensors that are more responsive and accurate, addressing the key limitations of previous models.
The combination of innovative materials and new technologies could enhance the usage scenarios for 3D accelerometers, making them applicable in fields ranging from environmental monitoring to aerospace.
Overall, the advancements in technology, coupled with the push towards integration in everyday lives, will continue to influence 3D accelerometer development for years to come.
Overall, the advancements in technology, coupled with the push towards integration in everyday lives, will continue to influence 3D accelerometer development for years to come.
These future trends indicate a continuous commitment to improving data collection methods, addressing user needs, and enhancing sensor capabilities in various fields.
End
The conclusion of this article is crucial in framing the insights gathered regarding 3D accelerometers and their diverse applications. It is essential to recognize how these devices have permeated various sectors, enhancing both functionality and safety. The integration of 3D accelerometers into everyday technology demonstrates their significance in fostering innovation. They serve not only as measurement tools but also as enablers of progress in numerous fields.
Summary of Findings
Throughout this exploration, we have identified several key findings regarding 3D accelerometers. These include:
- Functionality: The ability of accelerometers to measure movement across three axes is paramount in various applications, ranging from consumer electronics to healthcare.
- Operational Mechanics: Different types of accelerometers, such as capacitive and MEMS, provide unique benefits and limitations based on their design and intended use.
- Challenges: Issues related to calibration and environmental factors can impact the accuracy of measurements, which is a critical consideration for users.
- Innovative Applications: The role of 3D accelerometers in developing advanced automotive safety features, healthcare monitoring solutions, and robotics showcases their versatility.
The data also highlights the growing relevance of IoT integrations, which enable remote monitoring and control in innovative ways.
Future Outlook
Looking ahead, the future of 3D accelerometers appears promising. Key trends and developments to watch include:
- IoT Integration: The increasing connectivity of devices will likely enhance functionality and expand applications, making accelerometers more pervasive in different technological ecosystems.
- Miniaturization: The demand for smaller, more efficient devices will continue to drive innovations in manufacturing processes and design techniques. Innovations in material science could further aid these advances.
- Emerging Technologies: Breakthroughs in sensing technologies and algorithms may lead to accelerometers that provide even more precise data while minimizing calibration complications. These developments will open new avenues in fields like wearable tech and smart cities.
In closing, understanding the evolution and operational principles of 3D accelerometers allows us to appreciate their impact on technology and society. With the promise of continuous advancements and new applications, the relevance of these devices will only increase in the years to come.