Innovative Approaches to HPLC Method Development
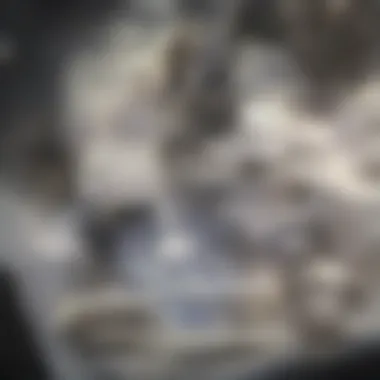
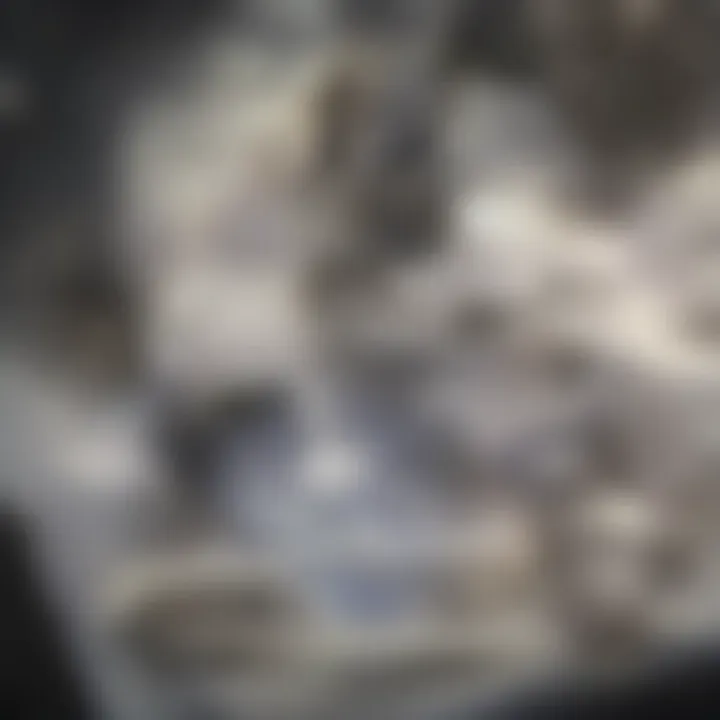
Article Overview
Purpose of the Article
This article aims to clarify the process of developing analytical methods for High-Performance Liquid Chromatography, known as HPLC. It is designed to assist students, researchers, educators, and professionals in grasping the systematic approach needed in the method development process. By addressing crucial elements like optimization and validation, the article serves as a comprehensive guide for anyone engaged in analytical chemistry. Special emphasis is placed on recent advancements which can enhance method efficacy.
Relevance to Multiple Disciplines
Research Background
Historical Context
HPLC emerged in the late 1960s as a powerful analytical tool for separating compounds. Over the decades, its evolution has made it indispensable in various laboratories worldwide. Initially based on classical liquid chromatography, HPLC has integrated advancements that enable higher resolution and faster analyses, providing precise quantification of substances in complex matrices.
Key Concepts and Definitions
Understanding some basic terminologies is essential to navigate through HPLC concepts effectively:
- Chromatography: A technique for separating mixtures into individual components.
- Mobile Phase: The solvent or mixture that transports the analytes through the column.
- Stationary Phase: The material inside the column that interacts with the analytes, facilitating separation.
- Retention Time: The time it takes for a compound to travel through the column to the detector.
HPLC employs these principles to separate and analyze components within a sample, providing invaluable data for decision-making in various research and industrial contexts. Following are some notable points about HPLC performance:
- High Resolution: HPLC can effectively resolve compounds that are very similar.
- Sensitivity: It detects even minute quantities of a substance.
- Versatility: HPLC can be used for a wide range of substances, from small molecules to large biomolecules.
Foreword to HPLC
High-Performance Liquid Chromatography (HPLC) has become a critical technique in the realm of analytical chemistry. Understanding its principles and applications is essential for many fields including pharmaceuticals, environmental analysis, and food testing. This section delves into the significance of HPLC, laying the groundwork for exploring its features, complexities, and advancements in method development.
HPLC allows for the effective separation, identification, and quantification of components in a mixture. The appeal of HPLC lies in its ability to analyze complex samples with high reliability and accuracy. This precision is vital in industries where the integrity of data can influence safety and regulatory compliance. Thus, it is important to elevate the discussion surrounding HPLC method development, focusing on systematic approaches that facilitate optimal results.
Definition of High-Performance Liquid Chromatography
High-Performance Liquid Chromatography refers to a sophisticated technique that employs liquid as the mobile phase for the separation of compounds in a sample. The essence of HPLC lies in its high efficiency and resolution compared to traditional liquid chromatography methods. In HPLC, the sample mixture is pushed through a column filled with a stationary phase. The components in the mixture interact differently with the stationary phase based on their unique chemical properties. This variation in interaction results in distinct separation, enabling further analysis.
Historical Development of HPLC
The historical context of HPLC showcases its evolution from early liquid chromatographic techniques. Initially, chromatography was a slow and often inefficient process, but advancements in technology led to considerable improvements in speed and resolution. The late 1960s marked a turning point when new packing materials and high-pressure pumps were introduced, enhancing the efficiency of separations.
By the 1980s, HPLC was widely adopted across various scientific fields. The validation of method performance became necessary, leading to the establishment of guidelines that ensure reproducibility and reliability within analytical laboratories. Today, HPLC methods continue to evolve, with innovations such as ultra-performance liquid chromatography (UPLC) pushing the boundaries of speed and resolution even further.
As HPLC continues to advance, understanding its past is integral to appreciating its current role in analytical science and the implications for future development.
As HPLC continues to advance, understanding its past is integral to appreciating its current role in analytical science and the implications for future development.
Principles of HPLC
The principles of High-Performance Liquid Chromatography (HPLC) lay the foundation for understanding how this analytical technique achieves its high resolution and efficiency. In method development, grasping these principles is essential for optimizing performance and ensuring accurate results. HPLC operates on the principles of liquid chromatography, where the separation of compounds is achieved based on their interactions with the stationary and mobile phases. This separation leads to insights about specific compounds in a sample and is crucial across multiple disciplines.
Theoretical Foundations
The theoretical base of HPLC involves the interactions between different phases during the separation process. The mobile phase is often a solvent or mixture of solvents that carries the sample through the stationary phase. This phase can be either a solid or liquid contained within a column. The differences in affinities between sample components and these phases dictate the overall separation.
Key aspects include:
- Partitioning: Samples distribute between the phases according to their solubility.
- Adsorption: This refers to the surface interaction of sample components with the stationary phase.
- Size Exclusion: Larger molecules are often excluded from entering pores in the stationary phase, affecting their retention time.
Overall, a robust understanding of these theories informs the selection of method parameters and helps refine the process for specific applications.
Components of HPLC System
The HPLC system comprises several critical components, each contributing uniquely to the overall functionality. Understanding these components helps in troubleshooting and improving methods.
Pump
The pump is responsible for delivering the mobile phase through the system at a consistent flow rate. Its ability to maintain pressure is vital in ensuring that the solvent remains in the liquid state under high pressure. The key characteristic of a pump is its precision in flow rate, which significantly affects resolution and reproducibility in results.
A popular choice in the HPLC community is the binary pump, which allows for mixing two solvents in varying proportions. This choice offers flexibility, making it beneficial for gradient elution techniques where the composition of the mobile phase varies over time. However, the complexity of this system can introduce challenges in maintenance and calibration.
Injector
The injector is another essential component that introduces the sample into the flow of the mobile phase. Its pivotal role is to ensure that the sample is added at the right moment and volume. A common type of injector is the autosampler, allowing for automated sample handling, which is efficient in routine analyses.
Injectors can provide high precision and low carryover of samples, which is essential for accurate quantification. Despite these advantages, injectors may require careful calibration to avoid variability that could impact results.
Column
The column is where the magic of separation occurs. It contains the stationary phase and is where different components of the sample interact differently. One key characteristic of columns is their length and internal diameter, which significantly influence separation efficiency.
The use of high-performance columns allows for finer packing and reduced particle size, yielding better resolution and shorter analysis times. However, these columns can be more expensive and may have specific temperature and pressure constraints that need to be adhered to for effective operation.
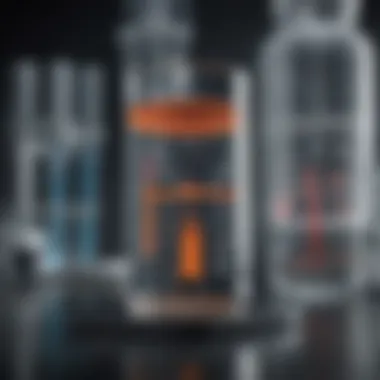
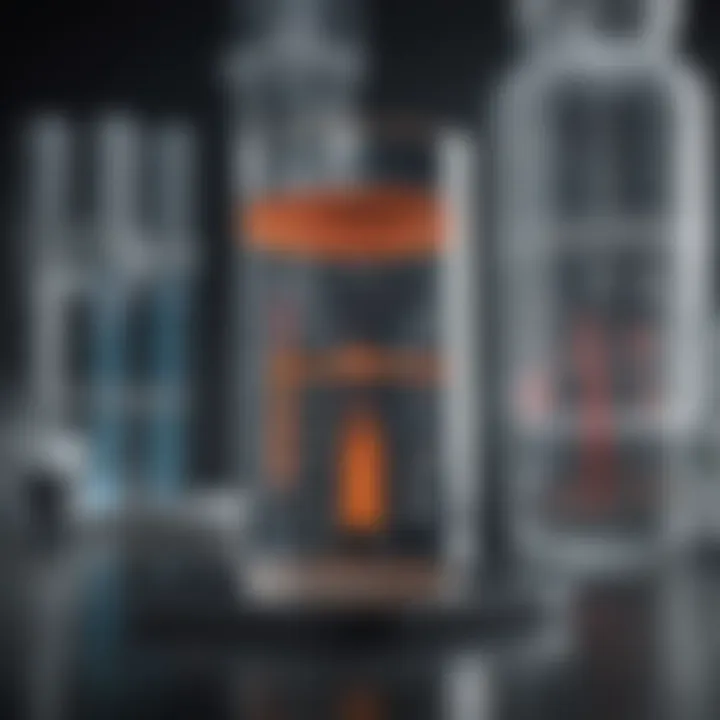
Detector
Finally, the detector is vital for identifying and quantifying components after separation. Different types of detectors exist, with the UV-Vis detector being the most widely used due to its sensitivity and ability to detect a wide range of compounds. This characteristic makes it a beneficial choice for many analytical applications.
Detectors operate by monitoring changes in the properties of the mobile phase as it passes through. Sensitivity and selectivity are critical features, impacting how low concentrations can be detected reliably. While UV-Vis detectors are abundant, they may not suffice for substances that do not absorb UV light, leading to the use of alternative detectors such as mass spectrometers.
With a thorough understanding of these components, researchers can better tailor HPLC methodologies to suit specific analytical needs, ensuring optimized performance and reliable results.
With a thorough understanding of these components, researchers can better tailor HPLC methodologies to suit specific analytical needs, ensuring optimized performance and reliable results.
This detailed comprehension of HPLC's principles and its components enables researchers and professionals to effectively develop analytical methods, paving the way for successful applications in various fields, from pharmaceuticals to environmental testing.
Method Development Process
The method development process is crucial in ensuring that HPLC (High-Performance Liquid Chromatography) analytical methods produce reliable and accurate results. It involves a systematic approach that aims to optimize performance and adapt to specific analytical needs. This process includes a variety of steps that help refine the method and ensure it achieves the desired level of identify, purity, and quantitation of analytes in complex mixtures.
When developing an HPLC method, one must consider several factors. These include the type of samples, the complexity of matrices, and regulatory guidelines. The robustness, precision, and reproducibility of the method are paramount. A well-defined process facilitates the elimination of variables that may affect results, thereby increasing confidence in the findings.
Objectives of Method Development
The objectives of method development encompass a series of goals aimed at enhancing the analytical capabilities of HPLC techniques. The primary objectives include:
- Establishing the method's selectivity to differentiate between closely related compounds.
- Maximizing the resolution to separate analytes effectively.
- Ensuring accuracy and precision for quantification.
- Determining the method's stability and robustness under various conditions.
Achieving these objectives not only enhances analytical performance but also builds a foundation for compliance with industry standards. This is essential for sectors like pharmaceuticals, where precise formulation is critical for the safety and efficacy of products.
Stage-wise Approach
The stage-wise approach to method development is systematic and allows for the careful examination of various parameters. This includes three key phases:
Selectivity Assessment
Selectivity assessment is the process of determining how well a method can differentiate targeted analytes from others present in a mixture. This is a significant step as it focuses on the unique characteristics that define each analyte.
In this context, selectivity is beneficial because it provides essential data concerning interference from non-target compounds. An effective selectivity assessment can lead to a method that accurately identifies and quantifies compounds, even in complex matrices. However, achieving high selectivity may sometimes require extensive optimization, which could be resource-intensive.
Resolution Optimization
Resolution optimization refers to improving the separation of two or more analytes in an HPLC system. High resolution ensures that peaks representing different compounds do not overlap, allowing for precise quantification.
A key characteristic of resolution optimization is the focus on peak shape and width. The resolution obtained indicates the effectiveness of the chromatographic system. A highly resolved method provides clean and interpretable results. However, increasing resolution can sometimes lead to longer analysis times, which may reduce throughput. Thus, a balance must be found to meet practical demands while maintaining data accuracy.
Throughput Considerations
Throughput considerations focus on the efficiency and speed of the analytical method. This aspect plays a critical role in high-volume laboratories that require rapid data collection.
The main characteristic of throughput is the number of samples analyzed in a given timeframe. High throughput methods minimize downtime and maximize productivity. While optimizing for throughput, one must ensure that quality does not suffer. Fast methods may compromise resolution or selectivity, which can lead to unreliable results. Therefore, it is vital to strike a balance between speed and analytical quality.
"A nuanced approach to method development allows researchers to build a foundation of reliability and accuracy in HPLC analyses."
"A nuanced approach to method development allows researchers to build a foundation of reliability and accuracy in HPLC analyses."
In summary, the method development process is pivotal for achieving reliable results in HPLC applications. Understanding the objectives, selectivity assessment, resolution optimization, and throughput considerations forms a comprehensive framework that enhances analytical methodology.
Optimization Techniques
Optimization techniques are essential in the development of analytical methods for High-Performance Liquid Chromatography (HPLC). They help in fine-tuning the variables affecting the separation process to achieve the best possible results. Effective optimization not only improves the resolution of analytes but also enhances the method's overall reliability and efficiency. Moreover, these techniques enable researchers and professionals to systematically assess the impact of various parameters, resulting in a more effective analytical procedure.
Variable Selection
In HPLC method development, selecting the right variables is critical. Variables like solvent composition, flow rate, and column temperature can significantly affect the separation process. Each variable can interact with other parameters, creating complex outcomes. Therefore, careful consideration must be given to choose which variables to test and optimize. Typically, the goal is to identify those variables that play a pivotal role in enhancing method performance while reducing time and resource expenditure.
Experimental Design
Experimental design refers to the strategic planning of experiments to ensure that data collected are robust and informative. It allows for systematic variation of selected variables to glean meaningful insights about their impact on separation performance.
Response Surface Methodology
Response Surface Methodology (RSM) is highly regarded in HPLC method optimization. The specific aspect of RSM lies in its capability to model the relationships between multiple variables and responses. By using mathematical and statistical techniques, RSM helps in identifying the optimal conditions for separation.
One key characteristic of RSM is its focus on understanding how various factors interact with each other. Such insight provides researchers with a more comprehensive view of method performance than traditional optimization methods. This makes it a popular choice in analytical development.
A unique feature of RSM is its ability to visualize the effects of variables through surface plots, making it easier to interpret results. However, RSM may require a significant amount of data and can be computationally intensive, which might be a disadvantage for smaller laboratories.
Fractional Factorial Designs
Fractional Factorial Designs (FFD) serve another important role in HPLC optimization. This experimental design technique is used for testing a fraction of all possible combinations of variables. Its contribution to method development lies in its efficiency, allowing researchers to determine the influence of several variables without needing to conduct exhaustive experiments.
The key characteristic of FFD is its ability to screen multiple factors simultaneously, thus saving both time and resources. This makes it a beneficial choice for method developers who need to identify key variables quickly.
A unique feature of FFD is its capability to identify interactions between factors without full factorial experimentation. However, while FFD can be efficient, it can also miss some important interactions if not carefully planned. This makes it essential for practitioners to use it wisely in their method development processes.
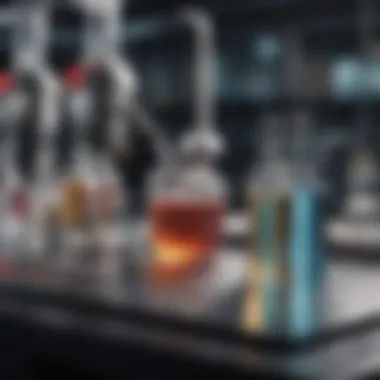
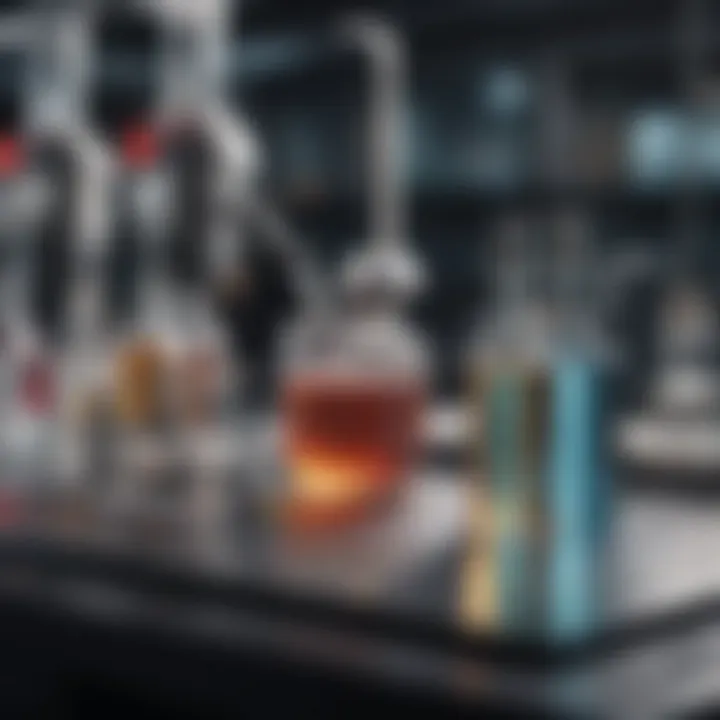
Validation of HPLC Methods
Validation in HPLC methods is a critical step that ensures analytical procedures are both accurate and reliable. The process serves to confirm that the methods applied can consistently yield valid results, meeting defined standards of performance. In settings such as pharmaceuticals, environmental testing, and food safety, where results have significant implications, robust validation of HPLC methods cannot be overlooked.
The validation process encompasses various parameters that assess different facets of method performance. This collective scrutiny assists in establishing a framework where methods are reproducible and suited for their intended purpose. As such, an understanding of validation becomes paramount for anyone involved in analytical chemistry.
Importance of Validation
Validation provides numerous benefits that extend throughout the research and development phases. It establishes a system of checks and balances for analytical methods, thereby strengthening the reliability of results yielded by High-Performance Liquid Chromatography. One intrinsic advantage is that it ensures compliance with regulatory standards. Many industries require strict adherence to guidelines, thus validating methods not only aligns with such needs but also enhances overall credibility.
Additionally, validation can aid in minimizing the possibility of errors. When methods are thoroughly validated, one can be more confident in their results, which translates to better decision-making in applications such as drug formulation and quality control.
Validation Parameters
The parameters involved in validation are distinct yet interconnected. Each parameter plays a role in demonstrating that the method performs as intended.
Linearity
Linearity is a measure of a method’s ability to produce results that are directly proportional to the concentration of the analyte in a sample. This aspect is vital because it indicates how well the method can quantify unknown samples across a range of concentrations. A key characteristic of linearity is that it allows for the establishment of calibration curves, serving as benchmarks for quantitatively assessing samples.
The unique feature of linearity lies in its straightforwardness. If the method shows good linearity, it is often considered more reliable for quantification tasks. However, it may require frequent recalibration, which could be a drawback in high-throughput labs.
Accuracy
Accuracy reflects the closeness of a measured value to the true value. It indicates whether the method can produce a result, that is a true representation of the concentration of the analyte. This makes accuracy a central pillar in assessing method effectiveness.
One of the benefits of ensuring high accuracy is that it instills confidence among users regarding the validity of data produced. When results are accurate, decisions based on those results are sound and trustworthy. However, the challenge resides in consistently achieving accuracy across different laboratories or even across different sample types due to variations in handling and preparation.
Precision
Precision refers to the consistency of results when the same method is applied multiple times under the same conditions. High precision indicates that the method can produce similar results repeatedly, which is especially important when evaluating biological samples where variability is high.
The key feature of precision is that it helps in understanding the reproducibility of a result. This is beneficial as it builds a case for the reliability of data outputs. Nonetheless, precision does not confirm accuracy; therefore, a method can be precise yet not accurate, which poses a significant weakness if left unaddressed.
Robustness
Robustness is the ability of a method to remain unaffected by small but deliberate variations in method parameters and provides an indication of its reliability during normal operational conditions. This helps in demonstrating method reliability in real-world applications, where conditions can often be unpredictable.
A noteworthy characteristic of robustness is that it aids in identifying aspects of method development that can be adjusted without impacting the overall performance. However, it should be noted that establishing robustness may require additional time and resources, which could be a disadvantage in fast-paced settings.
"Validation is not just a regulatory requirement; it is a cornerstone for ensuring method integrity and trustworthiness in results."
"Validation is not just a regulatory requirement; it is a cornerstone for ensuring method integrity and trustworthiness in results."
In essence, the validation of HPLC methods serves as a foundation upon which the credibility of analytical results rests. Through understanding the importance and parameters of validation, professionals can enhance the quality of their analytical methodologies.
Applications of HPLC
High-Performance Liquid Chromatography (HPLC) has a wide array of applications across several fields. Its longevity and adaptability in various sectors attest to its significance. This part of the article outlines the principal applications of HPLC, emphasizing its role in providing accurate and reliable analytical results. The benefits of utilizing HPLC include its ability to separate complex mixtures, analyze various components reliably, and its compatibility with numerous sample types. Moreover, HPLC plays a crucial role in ensuring regulatory compliance in various industries.
Pharmaceutical Industry
HPLC is indispensable in the pharmaceutical sector. It is the method of choice for quality control testing and stability studies. The pharmaceutical industry relies on HPLC to ensure the purity and concentration of active pharmaceutical ingredients (APIs). By applying this technique, researchers can identify impurities and degradation products in drug formulations.
The process of HPLC in drug testing includes method development, optimization, and validation. Often, the goal is to create methods that are sufficiently sensitive to detect trace amounts of contaminants.
Key benefits of using HPLC in pharmaceuticals include:
- Enhanced precision in measuring drug concentrations.
- Time efficiency resulting in quicker analysis.
- The ability to separate and identify multiple compounds in a single run.
Without reliable methods for analysis, it would be challenging to adhere to the stringent regulations mandated by health authorities. HPLC helps companies meet these guidelines.
Environmental Analysis
In environmental science, HPLC is essential for analyzing pollutants and natural compounds in various samples. This technique is utilized to measure contaminants in water, soil, and air samples. The accuracy of HPLC allows for effective assessment of environmental health risks.
Analyzing environmental samples requires overcoming challenges such as complex matrices and variable concentrations. HPLC offers the selectivity needed to discern subtle differences in sample composition.
The key applications include:
- Detection of pesticides in water sources.
- Monitoring of heavy metals in soil samples.
- Analysis of volatile organic compounds in air.
Regular monitoring and reporting of these substances are crucial. HPLC contributes to these efforts by providing precise data that aids in regulatory compliance and environmental protection.
Food and Beverage Testing
HPLC has a significant impact on food safety by identifying additives, preservatives, and natural substances in food and beverages. The method's ability to analyze multiple components simultaneously makes it valuable in ensuring that products meet quality and safety standards.
Applications in this sector include:
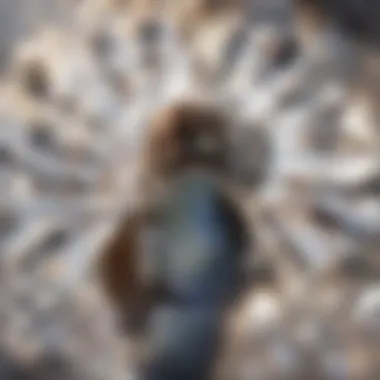
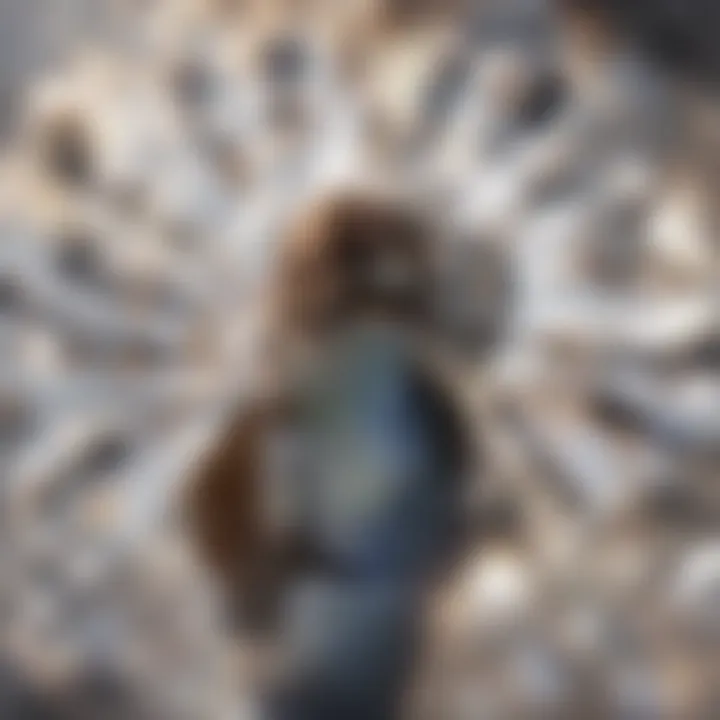
- Determination of food colorants and flavors.
- Measurement of vitamins and nutrients in supplements and food products.
- Analysis of contaminants such as pesticides and mycotoxins.
Using HPLC, manufacturers can ensure their products are safe for consumption. High accuracy and reliability in testing are essential for consumer trust and regulatory compliance in the food industry.
"The versatility of HPLC enables it to serve multiple industries with the highest standards of analysis."
"The versatility of HPLC enables it to serve multiple industries with the highest standards of analysis."
Emerging Trends in HPLC
The landscape of High-Performance Liquid Chromatography (HPLC) is continually evolving, reflecting the broader trends in analytical chemistry and technology. These emerging trends are crucial as they shape the future of analytical methods, enhancing precision, efficiency, and accessibility in various applications. As industries adapt to these advancements, understanding these trends becomes essential for students, researchers, educators, and professionals in the field.
Miniaturization of HPLC Systems
Miniaturization is a significant trend in HPLC, driven by the demand for efficient and economical analysis. Smaller systems require less sample and solvent, minimizing waste and reducing costs. This compact design also leads to faster analysis times, which is critical in fast-paced environments like pharmaceuticals and environmental testing.
Benefits of miniaturized HPLC systems include:
- Efficiency: Smaller systems can operate faster, providing quicker results.
- Reduced Costs: They use less solvent and sample, leading to cost savings.
- Portability: Miniaturized systems offer opportunities for field work and onsite analysis.
With ongoing advancements in technology, miniaturization will likely continue, making HPLC more accessible across many fields. Researchers can leverage these systems for more frequent testing without the burden of high costs or extensive resources.
Integrated Analysis Platforms
Integrated analysis platforms represent another significant trend in HPLC. These systems combine multiple analytical techniques into one platform, allowing for a more comprehensive approach to analysis. For example, platforms that integrate HPLC with mass spectrometry (MS) enhance detection capabilities and provide more detailed analytical results.
This integration offers several advantages:
- Comprehensive Data: Multiple analytical techniques provide a broader understanding of sample composition.
- Time-Saving: Integrated platforms streamline workflow, allowing for simultaneous analyses.
- User-Friendly: These systems often come with advanced software that aids in data analysis and interpretation.
As the need for multi-faceted analyses grows, integrated platforms become invaluable for research and industry. They ensure that users can obtain quality data efficiently.
Embracing emerging trends in HPLC will not only keep laboratories competitive but also ensure that analytical methods meet the evolving needs of science and technology.
Embracing emerging trends in HPLC will not only keep laboratories competitive but also ensure that analytical methods meet the evolving needs of science and technology.
Challenges in HPLC Method Development
The process of developing analytical methods for High-Performance Liquid Chromatography (HPLC) is not without its challenges. Understanding these challenges is vital for researchers and professionals engaged in method development. Addressing these issues can significantly enhance the efficiency and accuracy of the analytical results achieved.
Complex Sample Matrices
One of the most significant challenges faced in HPLC method development is dealing with complex sample matrices. Samples from real-world environments often contain various interfering substances. These can include proteins, lipids, carbohydrates, and inorganic materials, which can complicate the analysis. It is crucial to separate the target analytes from these extraneous components to ensure accurate measurements.
Addressing complex matrices involves several strategic approaches:
- Sample Preparation: Techniques such as solid-phase extraction or liquid-liquid extraction can help eliminate unwanted substances.
- Method Optimization: Adjusting elution conditions, such as pH and solvent composition, can improve separation efficiency.
- Selectivity Assessment: It is essential to establish the selectivity of the method for the target analytes over matrix components.
Managing these aspects can lead to better specificity and sensitivity, thus fulfilling the analytical requirements. The following is true for most challenges in HPLC method development:
"Addressing challenges in analytical methods can transform potential limitations into opportunities for enhanced reliability and functionality."
"Addressing challenges in analytical methods can transform potential limitations into opportunities for enhanced reliability and functionality."
Regulatory Compliance Issues
Another considerable challenge in HPLC method development is ensuring compliance with regulatory standards. Organizations such as the FDA and EMA have set forth stringent guidelines to ensure the safety and efficacy of pharmaceuticals and other products. These guidelines mandate rigorous validation for methods used in quality control and assurance.
Key considerations for meeting regulatory compliance include:
- Validation Parameters: Methods must be validated for aspects like linearity, accuracy, precision, and robustness. Each of these factors must meet specific criteria established by regulatory bodies.
- Documentation: Comprehensive documentation is vital. This documentation should include not only the method protocol but also records of validation studies.
- Continuous Monitoring: Regulatory compliance is not a one-time task. Continuous monitoring and validation are required to ensure ongoing reliability and adherence to standards.
Understanding these aspects is crucial for scientists and professionals engaged in the HPLC method development process. Successfully navigating these challenges promotes more reliable results while ensuring adherence to industry standards.
Culmination
The development of analytical methods for High-Performance Liquid Chromatography (HPLC) is crucial for achieving reliable and accurate results across various fields. This article highlights the significance of systematic method development, optimization techniques, and validation processes. Each of these aspects plays a fundamental role in ensuring that analytical methods meet the required standards for precision and robustness.
In the realm of HPLC, understanding the method development process lays the foundation for effective application in industries such as pharmaceuticals, environmental testing, and food safety. By following well-structured methodologies, researchers can systematically approach challenges posed by complex sample matrices and stringent regulatory requirements. This structured methodology not only aids in overcoming these challenges but also drives innovation and improvements in analytical techniques.
"A well-developed analytical method enhances confidence in results, ensuring public safety and product quality."
"A well-developed analytical method enhances confidence in results, ensuring public safety and product quality."
The benefits of a thorough method development process extend far beyond immediate analytical needs. They encompass broader implications in research and industrial applications, where reliability in data can influence decision-making and regulatory compliance. Furthermore, accurate method validation secures trust in the results produced, making it indispensable.
Summation of Key Points
- Method development is systematic and structured, involving various stages like optimization and validation.
- Optimizing method parameters is key to achieving selectivity and resolution.
- Validation ensures that methods adhere to required standards and are reliable.
- Emerging technologies are reshaping the landscape of HPLC, enabling miniaturization and integrated analysis.
Future Directions in HPLC Research
The future of HPLC method development is likely to witness several transformative advancements. Research is currently focusing on:
- Miniaturized HPLC Systems: Smaller systems will offer portability and reduced solvent consumption.
- Integrated Platforms: Combining various analytical techniques could provide comprehensive analytical insights.
- Automation and AI: Machine learning tools can assist in identifying optimal conditions for method development quickly.
- Real-Time Analysis: Developing methods that allow for immediate data acquisition could revolutionize process control in industries.
As the field evolves, continuously refining HPLC methods in response to new challenges and technologies will be essential for maintaining analytical integrity.