Understanding the Global Carbon Cycle: A Comprehensive Analysis
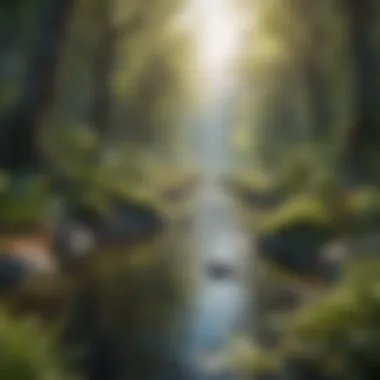
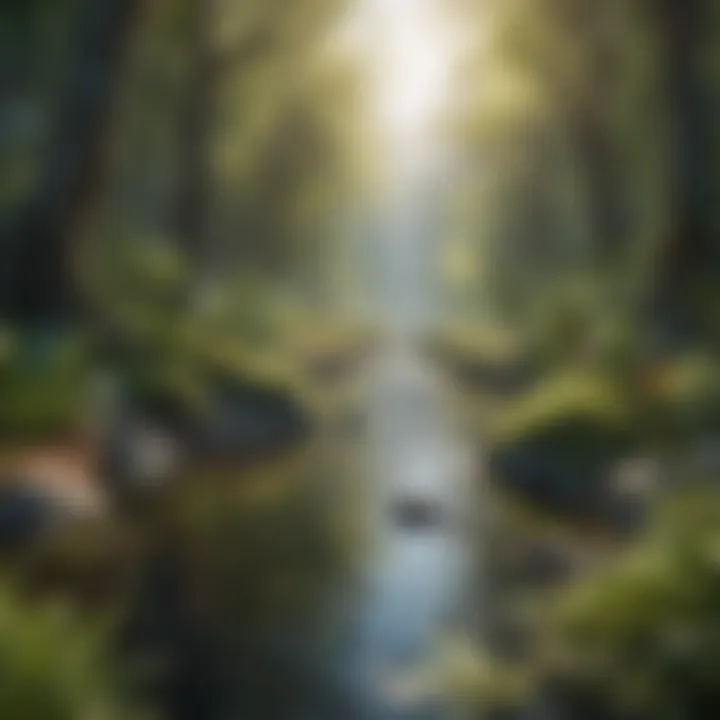
Intro
The global carbon cycle is an intricate system that governs how carbon moves across different parts of the Earth. It encompasses several components including the atmosphere, oceans, soil, and living organisms. Understanding this cycle is crucial for various fields such as environmental science, ecology, and climate policy. Through this exploration, we can understand how human activities disrupt this cycle and subsequently impact climate change.
Article Overview
Purpose of the Article
This article aims to demystify the global carbon cycle, presenting a detailed examination of each element within the system. By understanding the sources and sinks of carbon, we begin to see how essential these processes are for maintaining ecological balance. The objective here is not merely to inform but to provide readers with a framework to engage critically with climate-related issues.
Relevance to Multiple Disciplines
The global carbon cycle touches many academic and professional disciplines. For instance, biologists study its effects on ecosystems, while climatologists focus on its implications for climate models. Environmental policy makers need these insights to develop effective strategies to mitigate climate change. Understanding the carbon cycle therefore has broad implications, intersecting fields of science, sociology, and economics.
Research Background
Historical Context
The concept of the carbon cycle is not new. Early research laid the groundwork by identifying how carbon is stored and released in nature. From the Industrial Revolution onward, human impacts have increasingly altered these natural processes, leading to a surge in atmospheric carbon levels.
Key Concepts and Definitions
To navigate this complex topic, it's essential to clarify some key definitions:
- Carbon Sources: These are processes or activities that release carbon into the atmosphere, like burning fossil fuels and deforestation.
- Carbon Sinks: These act as reservoirs that absorb carbon from the atmosphere, with forests and oceans being prime examples.
- Ecosystems: Different ecosystems contribute to and are impacted by carbon cycling, establishing a delicate balance.
By grasping these concepts, readers will better understand how carbon dynamics influence natural and human systems.
Prolusion to the Carbon Cycle
The carbon cycle is fundamental to life on Earth, and its significance cannot be overstated. It represents the intricate relationship between the atmosphere, oceans, soil, and living organisms. This cycle is crucial for maintaining Earth's climate and supporting biodiversity. Understanding the carbon cycle is vital for students, researchers, educators, and professionals alike, as it lays the groundwork for comprehending larger ecological dynamics. It also informs discussions around climate change and environmental policy, making it a topic of critical importance.
The carbon cycle consists of various processes that transport carbon compounds through different Earth systems. The importance of studying this cycle lies in its implications for climate regulation and ecosystem health. As human activities increasingly disrupt this delicate balance, an in-depth exploration of the carbon cycle can provide insights into mitigation strategies and sustainable practices.
Definition of the Carbon Cycle
The carbon cycle refers to the continuous movement of carbon in various forms among the Earth's atmosphere, oceans, terrestrial biosphere, and geological deposits. Carbon exists in several states, including carbon dioxide (CO2), organic matter, and carbonate minerals. These forms play distinct roles across different ecosystems.
Key processes in the carbon cycle involve the exchange of carbon between various reservoirs. The atmosphere holds carbon dioxide, which plants utilize during photosynthesis, converting it into organic matter. Upon dying, plants return carbon to the soil as they decompose, while animals contribute through respiration and waste. The oceans also store vast amounts of carbon, acting as a significant sink.
Historical Context
Historically, the carbon cycle has been affected by natural processes and anthropogenic activities. In pre-industrial times, the balance of the carbon cycle remained relatively stable, with carbon exchanges occurring at a steady rate. However, since the onset of the industrial revolution in the 18th century, human activities have significantly altered this balance. The burning of fossil fuels, deforestation, and extensive agricultural practices have led to increased emissions of carbon dioxide into the atmosphere.
As a consequence, atmospheric CO2 levels have risen dramatically over the past century, leading to profound changes in climate patterns. Understanding the historical context of the carbon cycle elucidates the urgency with which we must approach carbon management today. The interactions within the carbon cycle continue to evolve, and this understanding informs ongoing climate-related discussions and actions.
Components of the Carbon Cycle
Understanding the components of the carbon cycle is crucial for recognizing how carbon moves through our environment. It covers the various forms of carbon storage and exchange, each playing its role. By categorizing these components, we can effectively analyze the balance of carbon among the atmosphere, terrestrial ecosystems, and oceans.
Atmospheric Carbon Dioxide
Atmospheric carbon dioxide is a primary gas in the carbon cycle. It is crucial for photosynthesis in plants. This process allows plants to convert CO2 into oxygen and biomass. When humans burn fossil fuels, they add more CO2 to the atmosphere, disrupting this natural balance. High levels of atmospheric CO2 lead to global warming. Thus, monitoring these levels is essential for climate science and mitigation strategies.
Biological Carbon Pools
Biological carbon pools refer to the carbon stored in living organisms. This pool consists of three main categories: plants, animals, and microorganisms.
Plants
Plants play a fundamental role in the carbon cycle. They absorb carbon dioxide from the atmosphere during photosynthesis, converting it into biomass. This process makes them a significant carbon sink. The key characteristic of plants is their ability to sequester carbon. This property is vital for mitigating climate change. A unique feature of plants is their capacity to store carbon in roots, leaves, and stems. However, deforestation reduces this capability, leading to increased atmospheric CO2 levels.
Animals
Animals also contribute to the carbon cycle, primarily through respiration. When animals breathe, they release CO2 back into the atmosphere. This characteristic highlights their role in maintaining atmospheric balance. Furthermore, animals' movement facilitates the transfer of nutrients in the soil, supporting plant growth. The unique aspect of animals is their consumption of plants, which creates a direct link in the carbon flow. However, large populations can lead to increased respiration, further impacting CO2 levels.
Microorganisms
Microorganisms, such as bacteria and fungi, are essential in the decomposition process. They break down organic matter, releasing carbon back into the soil and atmosphere. This ability makes microorganisms key players in nutrient cycling within ecosystems. Their significant characteristic is their rapid reproduction and adaptability, which allows them to dominate various environments. One advantage of microorganisms is their role in soil health and fertility. However, if excessive organic matter is introduced, it can lead to imbalances in the carbon cycle.
Oceanic Carbon Dynamics
The oceans play a vital role in regulating atmospheric CO2. Marine life, particularly phytoplankton, absorbs CO2 during photosynthesis. Oceans also store vast amounts of carbon, acting as a carbon sink. They release CO2 through natural processes, such as respiration and oceanic outgassing. Changes in ocean temperature and chemistry can affect these dynamics, impacting global climate patterns.
Soil Carbon Storage
Soil serves as a major carbon reservoir. Carbon is stored in the soil as organic matter from decomposed plants and animals. This biological process contributes significantly to soil health and fertility. However, land-use changes, such as agriculture and urbanization, can lead to carbon loss from the soil. Understanding soil carbon storage is essential for developing strategies to enhance carbon sequestration and mitigate climate change.
Processes of the Carbon Cycle
Understanding the processes of the carbon cycle is crucial for grasping how carbon moves through various environmental systems. These processes are the foundation that sustains life and impacts climate conditions on Earth. Each process involves dynamic interactions that contribute to the regulation of carbon levels in the atmosphere, oceans, and terrestrial environments. This section outlines the key processes, highlighting their advantages and considerations.
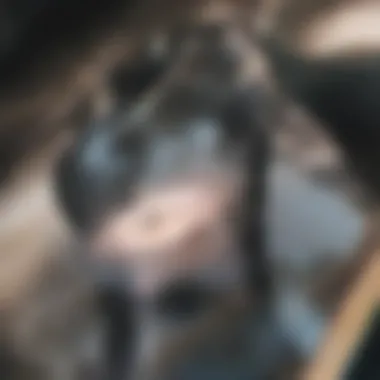
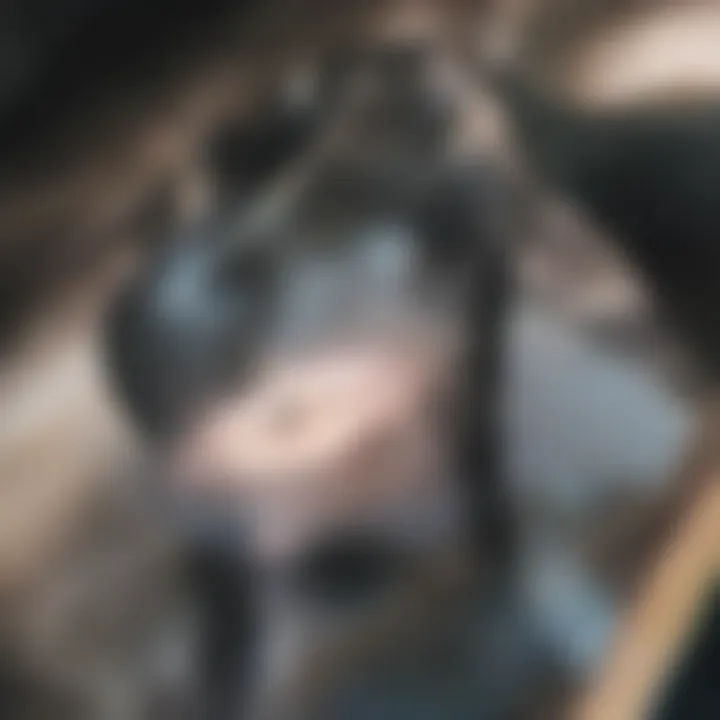
Photosynthesis
Photosynthesis is a vital process primarily carried out by plants, algae, and some bacteria. In this process, these organisms convert carbon dioxide from the atmosphere into organic matter using sunlight as energy. The formula can be summarized as follows:
CO2 + O + light โ C62O6 + O2
Through photosynthesis, carbon is assimilated into biomass, contributing to the growth of plants and providing energy for other living organisms through food chains. This process not only helps to sequester atmospheric CO2 but also generates oxygen, which is essential for aerobic life. Consequently, promoting photosynthesis can be beneficial for climate change mitigation by reducing greenhouse gas concentrations.
Respiration
Respiration is the process whereby living organisms, including animals and microorganisms, consume organic carbon compounds and release carbon dioxide back into the atmosphere. This process occurs in all aerobic organisms and plays a significant role in the carbon cycle. The equation is:
C62O6 + O2 โ CO2 + O + energy
During respiration, energy stored in organic molecules is released, making it available for cellular processes. While respiration returns CO2 to the atmosphere, it is essential for maintaining the balance within ecosystems. Increased respiration rates can occur as temperatures rise, which leads to higher CO2 emissions, further influencing climate dynamics.
Decomposition
Decomposition is the breakdown of organic matter by decomposers, such as fungi and bacteria. This process plays an important role in nutrient cycling and the carbon cycle, as it returns stored carbon back to the soil and atmosphere. Decomposers convert dead organic material into simpler compounds, releasing carbon dioxide in the process. Furthermore, they also produce nutrients that are essential for plant growth.
Key aspects of decomposition include:
- Breakdown of complex organic molecules
- Release of nutrients for uptake by plants
- Contribution to soil carbon storage
Without decomposition, ecosystems would be overwhelmed by organic waste. Therefore, promoting healthy decomposition processes is vital for maintaining soil health and carbon balance.
Oceanic Uptake and Release
The oceans function as one of the largest carbon sinks on the planet, absorbing significant amounts of atmospheric CO2. The uptake of carbon occurs through physical processes and biological activities. When CO2 dissolves in seawater, it reacts with water to form carbonic acid, which subsequently dissociates to produce bicarbonate and carbonate ions.
Important points about oceanic carbon dynamics include:
- Oceans regulate atmospheric CO2 levels
- Marine organisms utilize carbon for photosynthesis and calcification
- Changes in temperature and acidity can affect absorption capacity
However, anthropogenic activities and climate change can disrupt these processes, leading to ocean acidification and impacting marine life. Thus, maintaining the health of our oceans is critical for sustaining the balance of the carbon cycle.
Anthropogenic Influence on the Carbon Cycle
Human actions have significant ramifications on the global carbon cycle. This section focuses on various activities that contribute to the disruption and alteration of natural carbon processes. Understanding these influences is crucial for recognizing how our decisions impact climate and ecosystems.
Fossil Fuel Combustion
Fossil fuel combustion is perhaps the most substantial factor in anthropogenic emissions of carbon dioxide. This process releases carbon that has been stored underground for millions of years, drastically increasing the atmospheric CO2 levels. Combustion occurs primarily in power plants, vehicles, and industrial processes. As a result, it accelerates climate change, contributing to global warming.
"The burning of fossil fuels is responsible for over 70% of global CO2 emissions."
"The burning of fossil fuels is responsible for over 70% of global CO2 emissions."
Efforts to transition to renewable energy sources are critical. Options such as solar, wind, and hydroelectric power can significantly reduce our reliance on fossil fuels. Mitigating fossil fuel combustion will be a significant step in slowing the rate of climate change and restoring balance to the carbon cycle.
Deforestation
Deforestation is another critical contributor to the anthropogenic influence on the carbon cycle. When forests are cleared in large scales for agriculture or urban development, they release stored carbon dioxide into the atmosphere. Trees act as carbon sinks, absorbing CO2 during photosynthesis. The removal of forests not only reduces this capacity but also contributes additional carbon release.
Some significant drivers of deforestation include:
- Agriculture expansion
- Urban sprawl
- Logging
Conservation and responsible management of forests can help mitigate these impacts. Reforestation practices can restore ecosystems and regain lost carbon storage capacity, making it essential for addressing climate change.
Agricultural Practices
Agriculture plays a dual role in the carbon cycle. It can be a source of significant emissions while also providing opportunities for carbon sequestration. Fertilizer use and livestock farming are two areas of concern.
The use of synthetic fertilizers releases nitrous oxide, a potent greenhouse gas. Similarly, methane emissions from livestock are a significant contributor to climate change.
Conversely, adopting sustainable agricultural practices can reduce emissions. Methods such as:
- Crop rotation
- Reduced tillage
- Agroforestry
can enhance soil carbon storage and improve carbon management in farming systems. These practices promote healthier soils and ecosystems, contributing to overall balance in the carbon cycle.
Urbanization
Urbanization has dramatically altered landscapes and ecosystems, with significant impacts on the carbon cycle. The replacement of natural habitats with urban areas leads to increased energy consumption and greenhouse gas emissions. Urban areas tend to have higher concentrations of vehicles and industries, resulting in elevated carbon output.
Strategies to combat urban contributions to carbon emissions include:
- Promoting public transportation
- Implementing green building practices
- Enhancing urban green spaces
Such initiatives can help cities adapt to sustainable practices, reducing their carbon footprint and fostering healthier environments. Understanding the interconnection of urban development and the carbon cycle is vital for crafting effective solutions.
Recognizing the anthropogenic influences on the carbon cycle is essential for mobilizing effective responses to climate change. Each action, whether in energy production, land use, or urban planning, carries implications that can either exacerbate or mitigate the evolving climate crisis.
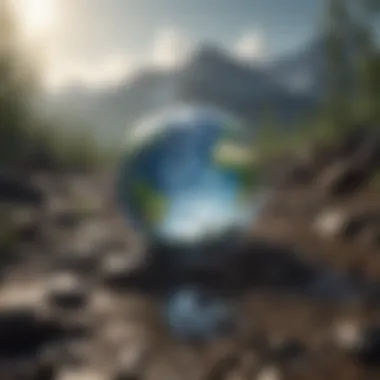
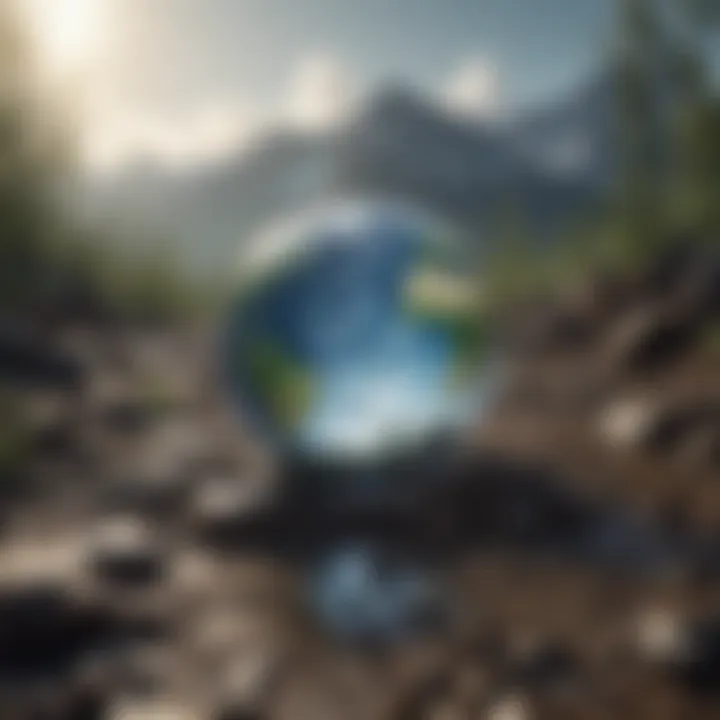
The Role of Carbon Sinks
The importance of carbon sinks extends beyond just lowering carbon levels in the atmosphere. They also support biodiversity, improve soil health, and contribute to water regulation in their ecosystems. Therefore, the understanding of carbon sinks goes hand in hand with recognizing their capacity to sequester carbon and influence climate systems.
Forests as Carbon Sinks
Forests serve as significant carbon sinks, absorbing vast amounts of carbon dioxide through the process of photosynthesis. They store carbon in the form of biomass, which includes trees, plants, and soil organic matter. In fact, it is estimated that forests contribute to about 80% of terrestrial carbon storage. This storage capacity varies among forest types, with tropical forests generally having higher sequestration rates compared to boreal or temperate forests.
Forests are not just passive absorbers. They actively contribute to carbon cycling by supporting diverse flora and fauna that enhance soil fertility. However, factors such as deforestation and forest degradation threaten this ability. Logging, agricultural expansion, and urban encroachment release stored carbon back into the atmosphere, worsening climate change. The protection and restoration of forests are crucial strategies in addressing these issues.
Soil as a Carbon Sink
Soil is often overlooked but plays a key role as a carbon sink, storing approximately seventy-five percent of the Earth's terrestrial carbon. Organic matter, which includes decomposed plants and animal residues, is a major component of soil carbon. When plants die, their carbon is incorporated into the soil, where it can remain for decades.
Agricultural practices can greatly influence soil carbon storage. Sustainable practices, such as cover cropping and reduced tillage, improve soil structure, enhancing its capacity to sequester carbon. Conversely, industrial farming can deplete soil by disrupting its natural balance, leading to increased carbon release. Increasing awareness and implementation of sustainable practices is essential to optimize soil's role in the carbon cycle.
Oceans as Carbon Sinks
Oceans are the largest carbon sink on the planet, absorbing about 30% of total atmospheric carbon dioxide. Through biological processes, such as photosynthesis by phytoplankton, and physical processes like dissolution, oceans play a fundamental role in regulating global carbon levels.
Marine ecosystems, particularly coastal wetlands and coral reefs, enhance this sink capability. However, oceans are facing threats from climate change, such as acidification, which hinders their ability to absorb carbon. As sea temperatures rise and ecosystems alter, understanding and protecting marine carbon sinks becomes a pressing issue. The health of oceanic systems directly correlates with our global efforts to manage carbon levels effectively.
"The preservation of carbon sinks is not merely an environmental issue; it is central to our survival and the stability of the planet's climate system."
"The preservation of carbon sinks is not merely an environmental issue; it is central to our survival and the stability of the planet's climate system."
Maintaining and enhancing the functionality of these carbon sinks through sustainable practices and policies remains crucial for mitigating climate change.
Carbon Cycle Feedback Mechanisms
Feedback mechanisms in the carbon cycle are crucial in understanding how different components of the cycle influence each other. These mechanisms can significantly affect the balance of carbon in the atmosphere, oceans, and soil, thereby influencing climate change. Feedback loops either amplify (positive feedback) or diminish (negative feedback) the effects of changes in the carbon cycle. Understanding these mechanisms provides insight into potential climate scenarios and informs mitigation strategies.
Positive Feedback Loops
Positive feedback loops in the carbon cycle accelerate changes. One primary example is the melting of Arctic ice. As temperatures rise due to increased atmospheric CO2, more ice melts. Ice reflects sunlight, while dark ocean water absorbs it. This absorption raises the Earth's temperature further, causing more ice to melt.
Another significant positive feedback involves permafrost. As global temperatures increase, permafrost thaws, releasing stored carbon dioxide and methane. These gases contribute to further warming, leading to additional permafrost thawing. This cycle has the potential to release massive amounts of greenhouse gases, exacerbating climate change.
Some key elements of positive feedback loops include:
- Increased greenhouse gas emissions: More emissions lead to higher temperatures.
- Loss of reflective surfaces: Darker surfaces absorb more heat.
- Ecosystem alterations: Changes in species distribution can disrupt carbon storage capabilities.
These phenomena underscore the urgency for effective climate policies.
Negative Feedback Mechanisms
Negative feedback mechanisms act as a counterbalance, moderating the effects of change. One common example is the role of plants in carbon uptake. As atmospheric CO2 levels rise, plants often increase photosynthesis, absorbing more carbon dioxide. This process can help mitigate the effects of increased greenhouse gases by drawing carbon back into biomass and soil.
Moreover, increased ocean temperatures can enhance the ocean's ability to sequester carbon. Warmer waters might initially release CO2, but an increase in phytoplankton growth due to nutrient runoffs can lead to more carbon being absorbed by these organisms.
Key considerations of negative feedback mechanisms:
- Natural carbon sinks: Healthy ecosystems that grow can offset carbon emissions.
- Restoration efforts: Reforestation or wetland restoration can enhance carbon capture.
- Species adaptation: Ecosystems that adapt may adjust their carbon sequestration capabilities.
Understanding these mechanisms highlights pathways for creating resilience against climate change. It demonstrates that while feedback loops can escalate climate challenges, strategic environmental management can provide counteracting solutions.
"Effective climate action must recognize both feedback mechanisms, as they reveal the complexity of carbon dynamics and our interconnected role within this system."
"Effective climate action must recognize both feedback mechanisms, as they reveal the complexity of carbon dynamics and our interconnected role within this system."
Impacts of Climate Change on the Carbon Cycle
The impacts of climate change on the carbon cycle are profound and multifaceted. Understanding these impacts is crucial for developing effective environmental policies and mitigation strategies. Climate change affects various components of the carbon cycle, altering not only carbon sources and sinks but also the overall dynamics that govern carbon exchanges between the atmosphere, oceans, and land.
Increasing Atmospheric CO2 Levels
The concentration of carbon dioxide in the atmosphere has been rising dramatically due to human activities, particularly the burning of fossil fuels. This increase in atmospheric CO2 levels leads to a broader warming effect, which can further affect the carbon cycle. Higher CO2 levels can enhance photosynthesis, resulting in faster plant growth. However, this is not a straightforward benefit.
As plants absorb more CO2, they may not necessarily store more carbon long-term, especially if climate conditions become less favorable. Additionally, warmer temperatures can lead to increased respiration rates in plants and soil, releasing CO2 back into the atmosphere. Thus, the net effect can potentially shift the carbon balance towards greater greenhouse gas emissions.
Changes in Ocean Chemistry
The oceans are significant carbon sinks, absorbing about a quarter of the CO2 emissions produced by human activity. However, increasing levels of CO2 lead to ocean acidification, a decline in pH levels in seawater. This change in chemistry can impact marine organisms, especially those with calcium carbonate shells or skeletons, such as corals.
- Ocean acidification impacts:
- Coral Reefs: Weaken structures and hinder growth.
- Shellfish: Reduce the ability to create shells.
- Ecosystem Health: Disrupt food webs and biodiversity.
As marine life struggles to adapt, these changes can reduce the ocean's ability to sequester carbon, further exacerbating the problem.
Alterations in Ecosystem Functions
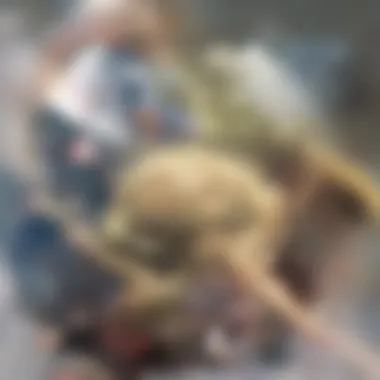
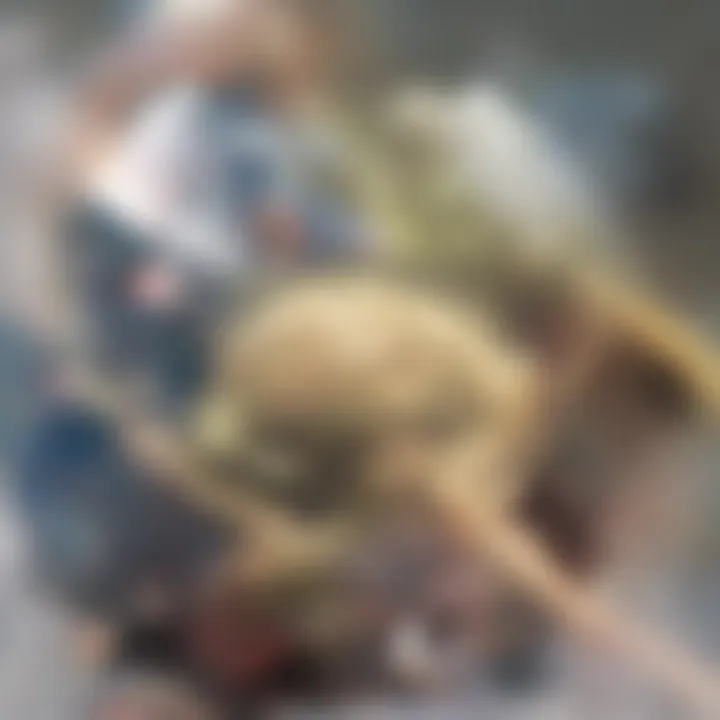
Climate change leads to substantial shifts in ecosystem functions, including alterations in species distributions and interactions. Forests, wetlands, and grasslands are essential for carbon storage. However, rising temperatures, shifts in precipitation patterns, and extreme weather events can threaten these ecosystems.
- It causes shifts in:
- Species Composition: Some species may thrive while others decline.
- Carbon Storage: A loss of biodiversity can decrease resilience and carbon storage capacity.
- Fires and Pest Infestations: Increased temperatures can heighten fire risks and pest populations, contributing to carbon release.
These shifts in ecosystem functions underscore the complex interplay between climate change and the carbon cycle, highlighting the urgent need for holistic approaches to environmental management.
These shifts in ecosystem functions underscore the complex interplay between climate change and the carbon cycle, highlighting the urgent need for holistic approaches to environmental management.
Mitigation Strategies
Mitigation strategies are critical in addressing the challenges posed by the carbon cycle. As the consequences of climate change become more evident, it is essential to implement effective measures to minimize greenhouse gas emissions. This section focuses on three key strategies: carbon capture and storage, reforestation and afforestation, and sustainable agricultural practices. Each of these strategies offers unique benefits and considerations for both the environment and society.
Carbon Capture and Storage
Carbon capture and storage (CCS) is a technology designed to trap carbon dioxide emissions from power plants and industrial processes. This technique prevents CO2 from entering the atmosphere by capturing it at the source. The captured CO2 is then transported and stored deep underground in geological formations.
The significance of CCS lies in its ability to significantly reduce net emissions from high-output sectors. This strategy can be particularly valuable in industries that are difficult to decarbonize, such as cement or steel production. However, CCS presents challenges, including the need for substantial upfront investment and ensuring the long-term security of stored carbon.
"The implementation of CCS can play a vital role in meeting national and international climate goals."
"The implementation of CCS can play a vital role in meeting national and international climate goals."
Reforestation and Afforestation
Reforestation and afforestation represent two complementary approaches for enhancing carbon sinks. Reforestation involves replanting trees in areas where forests have been depleted. Afforestation, on the other hand, refers to planting trees in previously non-forested areas.
These strategies not only sequester carbon but also restore biodiversity and improve water quality. Forests provide essential habitat for wildlife and can enhance soil health. However, land use conflicts can arise. Considerations must be made regarding local communities and existing ecosystems before implementing such projects. Additionally, ensuring that the new forests are resilient to climate change is crucial for their long-term effectiveness.
Sustainable Agricultural Practices
Sustainable agricultural practices play an essential role in reducing carbon emissions from the agricultural sector. These practices aim to enhance soil health, optimize water use, and improve crop yields while minimizing the environmental footprint. Strategies include crop rotation, cover cropping, and reduced tillage.
By implementing these practices, farmers can enhance soil carbon storage. Moreover, sustainable agriculture can result in better food security and livelihoods for communities. However, scaling these practices requires education, resources, and sometimes, a shift in agricultural policy. It is important to recognize that the success of these practices depends on collaboration among farmers, policymakers, and researchers.
In summary, mitigation strategies are crucial for ensuring the balance of carbon within the global cycle. Carbon capture and storage, reforestation, afforestation, and sustainable agricultural practices offer pathways to reducing emissions. Each strategy has its unique benefits and challenges. A comprehensive approach that combines these strategies will be essential in addressing the carbon cycle disruptions caused by human activities.
Future Directions in Carbon Cycle Research
As our understanding of the carbon cycle deepens, the importance of future research directions becomes paramount. These directions not only enhance our comprehension of carbon dynamics but also offer pathways to mitigate climate change. Effective exploration into innovative technologies, policy initiatives, and interdisciplinary approaches can yield significant benefits not just for environmental science but for global sustainability efforts as well.
Emerging Technologies
Emerging technologies represent a frontier in carbon cycle research. Innovations in remote sensing, for example, allow researchers to monitor carbon flux in real-time. This capability provides detailed insights into carbon sources and sinks with high temporal resolution. Additionally, advancements in artificial intelligence and machine learning can play a vital role in modeling carbon cycle processes more accurately, aiding predictive analytics regarding climate variations and carbon emissions.
Moreover, biotechnology offers tools for enhanced carbon sequestration. Practices such as bioengineering plants for increased efficiency in carbon uptake are being researched intensively. The development of carbon capture and storage (CCS) technologies is another area of focus. These technologies aim to capture CO2 emissions from industrial sources and securely store them underground, minimizing atmospheric release. The adoption of such technologies could significantly reduce the carbon footprint of various industries, contributing to global carbon balance.
Policy and Global Initiatives
Effective policy-making is crucial for implementing sustainable practices informed by carbon cycle research. Global initiatives, such as the Paris Agreement, underscore the importance of international cooperation in combating climate change. Policymakers must consider research findings to develop evidence-based regulations that enforce limits on fossil fuel emissions and promote greenhouse gas reductions.
Carbon pricing mechanisms, including carbon taxes and cap-and-trade systems, are gaining traction worldwide. These initiatives provide financial incentives for reducing carbon emissions, thus stimulating innovation in clean energy technologies. Furthermore, the role of governmental and non-governmental organizations in promoting educational campaigns is essential. Raising public awareness about the significance of the carbon cycle encourages individual and community actions that support carbon neutrality.
Interdisciplinary Approaches
Interdisciplinary approaches are fundamental in advancing our understanding of the carbon cycle. A synthesis of insights from ecology, atmospheric science, and social sciences can foster a holistic perspective. For example, integrating ecological research with socioeconomic studies can evaluate how land-use changes affect carbon dynamics.
Additionally, collaboration between scientists, engineers, and policymakers can enhance problem-solving strategies. These collaborations can lead to innovative solutions that are both scientifically sound and socially accepted. It is crucial to develop partnerships across various sectors to ensure that research findings translate into effective practices and policies.
"An interdisciplinary approach can catalyze the integration of diverse knowledge systems, thus amplifying the impacts of carbon cycle research on policy and community practices."
"An interdisciplinary approach can catalyze the integration of diverse knowledge systems, thus amplifying the impacts of carbon cycle research on policy and community practices."
To sum up, future directions in carbon cycle research hold great promise. By focusing on emerging technologies, implementing effective policies, and fostering interdisciplinary collaboration, we can effectively address the challenges posed by climate change and strive for a more sustainable future.
Ending
The conclusion serves as a critical summation of the myriad elements discussed throughout the article. It underscores the intricate design and functionality of the global carbon cycle, highlighting its essential role in sustaining life on Earth. As the article has explored, the carbon cycle is not merely a collection of processes; it is a dynamic system that links every aspect of our environment. Understanding these connections is vital, especially in the context of articulating effective responses to climate change.
Summary of Key Findings
The research showcases that:
- Carbon Movement: Carbon moves between the atmosphere, biosphere, hydrosphere, and geosphere through various processes such as photosynthesis and respiration.
- Human Impact: Anthropogenic activities, like fossil fuel combustion and deforestation, disrupt natural carbon sinks, increasing atmospheric carbon levels.
- Ecosystem Functionality: Different ecosystems, including forests, oceans, and soils, play unique roles in carbon storage.
- Climate Feedbacks: The system feedback loops can exacerbate or mitigate climate change effects.
- Mitigation Options: Strategies, such as carbon capture and reforestation, present avenues to mitigate human impacts on the carbon cycle.
Understanding these findings enables stakeholders to grasp the importance of sustainable practices and policies that can aid in maintaining the balance of the carbon cycle. Through responsible action, humanity can contribute positively to the planetโs ecological integrity.
Final Thoughts
The exploration of the global carbon cycle illuminates the urgent need for deeper awareness and action. As researchers, students, and professionals engage with this topic, they are not merely studying a scientific phenomenon. They are tapping into a pressing global issue that necessitates collaborative solutions across various disciplines.
Awareness of the intricate relationships within the carbon cycle can lead to informed decisions, influencing policy creation and community efforts to reduce carbon footprints.
"The most effective way to address climate change is through the interconnectedness of science and policy."
"The most effective way to address climate change is through the interconnectedness of science and policy."