Understanding Phase Change: Key Concepts & Applications
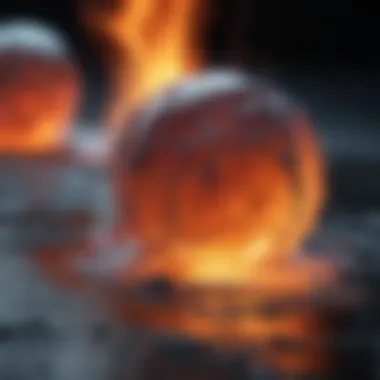
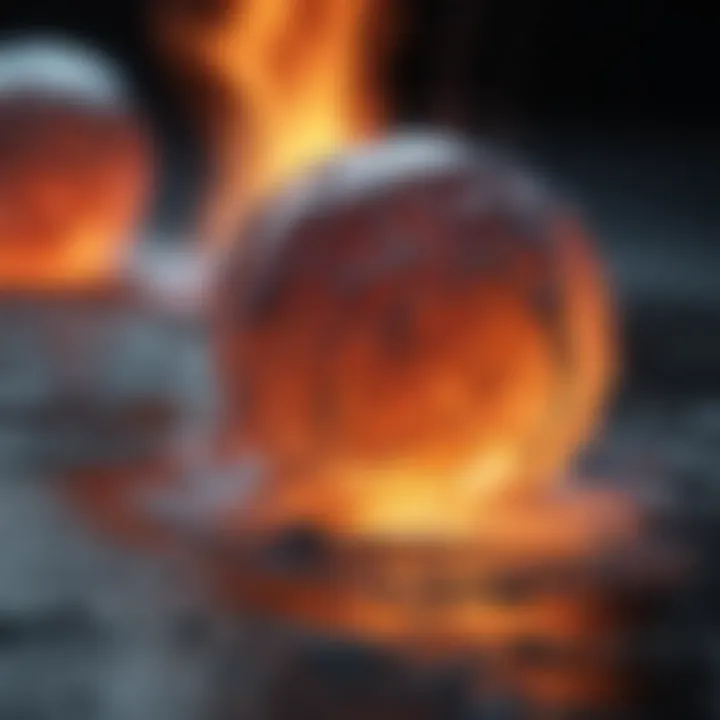
Article Overview
Phase change is a crucial concept in science that deals with the transformation of a substance from one state of matter to another, influenced by variations in temperature and pressure. Understanding these changes can provide insights into physical laws and applications in various fields. This overview aims to articulate why phase change matters and how it intersects with multiple disciplines.
Purpose of the Article
The primary purpose of this article is to dissect the fundamental principles of phase change and its types. By comprehending these concepts, readers can appreciate the significance of phase transitions in day-to-day life and advanced scientific endeavors. The discussion will include notable examples that demonstrate how these principles manifest in real-world scenarios.
Relevance to Multiple Disciplines
Phase change is an interdisciplinary concept relevant in physics, chemistry, engineering, and even environmental sciences. Understanding phase transitions is essential in diverse applications, including the development of new materials, energy systems, and understanding atmospheric phenomena.
- For example, in chemistry, the process is crucial for reactions occurring in different states.
- In physics, it plays a significant role in thermodynamics and statistical mechanics.
- In engineering, materials science heavily relies on the principles of phase transitions to design innovative products.
This interconnectedness highlights the value of a comprehensive understanding of phase change across various scientific landscapes.
Research Background
To appreciate the complexities of phase change, one must look into its historical context and fundamental concepts.
Historical Context
Historically, scientists have observed phase changes for centuries. Ancient civilizations recognized the transformations of water into ice and steam through natural phenomena, though they lacked an understanding of the underlying principles. It was during the 19th century, with the work of researchers like James Clerk Maxwell, that a more formal conceptual framework emerged. The development of thermodynamics also contributed significantly to our understanding of phase transitions, providing mathematical descriptions of energy exchanges.
Key Concepts and Definitions
In the context of phase change, several key concepts warrant explanation:
- States of Matter: Primarily, matter exists in four states - solid, liquid, gas, and in some cases, plasma. Each state has distinct properties regarding shape and volume.
- Phase Transition: This is the process where a substance shifts from one state to another. Common examples include melting, freezing, condensation, and sublimation.
- Critical Point: This refers to a specific temperature and pressure at which phase distinctions disappear. Beyond this point, a substance can be in a single phase regardless of the varying conditions.
- Latent Heat: During a phase transition, energy is absorbed or released without changing the temperature of the substance, termed latent heat.
Understanding these definitions lays the groundwork for the more advanced implications of phase change discussed in further sections.
Prologue to Phase Change
Understanding phase change is crucial in the study of materials and their behaviors under different conditions. Phase change encompasses the transformation of substances between solid, liquid, and gas states. This aspect of science not only affects our daily lives but also plays an essential role in various industries and research fields. Recognizing how substances react at various temperatures and pressures can lead to key innovations and improvements in technology, energy efficiency, and materials science.
Definition of Phase Change
Phase change refers to the transition of a substance from one state of matter to another as a result of changes in temperature or pressure. This process involves rearranging molecules, which can significantly alter the physical properties of the substance. For instance, water can exist as ice, liquid water, or steam, depending on the environmental conditions.
Historical Context
The understanding of phase change has evolved significantly over time. Early scientists like Robert Boyle and Joseph Louis Gay-Lussac laid the groundwork for the study of gases and their behavior, contributing foundational principles still in use today. In the 19th century, the work of Lord Kelvin advanced our knowledge of thermodynamics and phase transitions. These scientific milestones have established a framework that informs our current understanding of phase change phenomena.
Importance in Scientific Research
Phase change is a fundamental concept that underpins many scientific explorations. It is critical in fields such as material science, environmental science, and chemistry. For instance, the design of phase change materials (PCMs) is increasingly relevant in energy storage technologies, offering solutions for thermal management. Additionally, the study of phase change enhances our comprehension of natural processes, such as the water cycle, which affects climate and weather patterns.
"Understanding phase change leads to both practical applications and theoretical advancements, connecting multiple disciplines."
"Understanding phase change leads to both practical applications and theoretical advancements, connecting multiple disciplines."
In summary, comprehending phase change allows scientists and researchers to innovate and address practical challenges. This article will explore these facets in detail, emphasizing their implications in various contexts.
Fundamental Principles of Phase Change
Understanding the fundamental principles of phase change is crucial in grasping how substances transition between different states of matter. This section delves into the molecular behavior that drives these transitions and how external factors like temperature and pressure influence these processes.
A firm comprehension of these principles aids in various scientific domains, from materials science to environmental studies. Moreover, it helps to elucidate everyday phenomena, such as why ice melts at room temperature or how clouds form. Thus, the principles of phase change are not only foundational to physical sciences but also to practical applications in technology and engineering.
Molecular Behavior and State Changes
At the molecular level, phase changes occur due to the interactions among molecules. In solids, molecules are closely packed and vibrate around fixed positions. The energy is low in this state, resulting in a defined shape and volume. When energy is added, often through heat, the molecules begin to vibrate more vigorously. This added energy can lead to melting, where the solid transforms into a liquid.
In a liquid state, molecules are more freely moving, albeit still close together. They have enough energy to overcome some intermolecular forces, allowing them to flow. As more heat is applied, molecules gain enough energy to enter the gas phase through vaporization. This transition can happen at various points depending on the pressure conditions.
Conversely, when cooling occurs, the reverse takes place. Molecules lose energy, slow down, and can lead to condensation when a gas becomes a liquid. For instance, dew forms in the early morning as the temperature drops and air humidity condenses. Understanding these behaviors illustrates how phase changes are intimately linked to molecular motion.
Effects of Temperature and Pressure
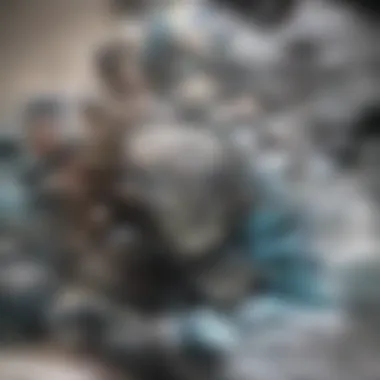
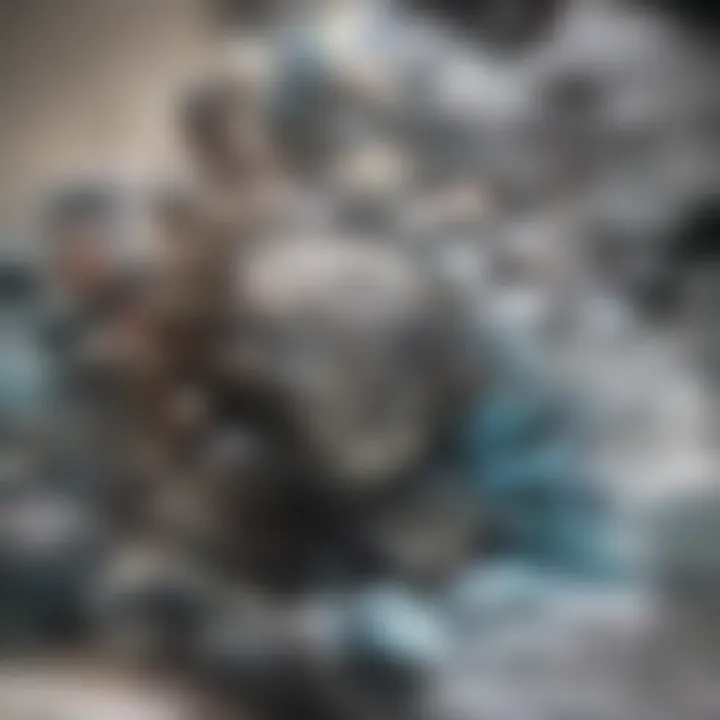
Temperature and pressure are pivotal factors in determining the phase of a substance. For example, water boils at 100 degrees Celsius at standard atmospheric pressure. However, if one increases the pressure, this boiling point rises. This relates to the concept of vapor pressure, where the exertion of pressure on a liquid can prevent it from vaporizing easily.
On the other hand, lowering pressure can enable boiling at lower temperatures. This principle is fundamentally important in cooking and industrial processes.
- Temperature increases lead to:
- Pressure increases result in:
- Temperature decreases can cause:
- Enhanced molecular motion
- Transition from solid to liquid (melting)
- Transition from liquid to gas (vaporization)
- Higher boiling points for liquids
- Greater stability for gas phases
- Transition from gas to liquid (condensation)
- Transition from liquid to solid (freezing)
In summary, the complex relationship between temperature, pressure, and phase behavior forms the foundation of thermal dynamics of materials. These principles are crucial for both theoretical models and real-world applications, influencing everything from climate patterns to industrial design.
"The understanding of phase change is essential for developing new materials and optimizing existing processes across various scientific fields."
"The understanding of phase change is essential for developing new materials and optimizing existing processes across various scientific fields."
This exploration of molecular behavior and the effects of temperature and pressure strengthens our grasp of the intricacies of phase change, laying the groundwork for further examination of its implications across multiple scientific disciplines.
Types of Phase Transitions
Phase transitions are critical in the understanding of material properties and their applications. They describe the transformation of substances between solid, liquid, and gas states. Knowledge of these transitions is beneficial in various fields, from material science to environmental science. Recognizing the types of phase transitions allows us to interact with materials intelligently, optimizing processes in industrial settings or enhancing everyday technologies.
Solid to Liquid: Melting
Melting is the process that changes a solid into a liquid. This transition occurs when a substance absorbs heat, increasing the energy of its molecules. This energy allows molecules to move freely, breaking the rigid structure of the solid. Melting points vary significantly among materials; for example, ice melts at 0°C while iron requires temperatures above 1,538°C. Understanding melting helps in various applications like casting metals or making ice cream, where control over the melting process is essential.
Liquid to Gas: Vaporization
Vaporization is the process where a liquid becomes a gas. This can happen through boiling or evaporation. Boiling occurs at a specific temperature, where the vapor pressure equals the atmospheric pressure. Evaporation is a slower process that occurs at any temperature. The significance of this phase transition is profound in environmental science and meteorology. For instance, the water cycle is driven by vaporization, impacting climate and weather patterns.
Gas to Liquid: Condensation
Condensation is when gas transforms back into a liquid. This happens when vapor cools down, losing energy. The dew you see in the morning is an example of this process. It condenses when the temperature of the air drops. Condensation plays a vital role in various industries, from refrigeration systems to cloud formation in the atmosphere. Understanding this phase transition helps manage humidity and temperature in numerous applications.
Liquid to Solid: Freezing
Freezing is the transition where a liquid turns into a solid as it loses energy. This process often occurs at a substance's freezing point. For example, water freezes at 0°C. In industrial applications, controlling freezing processes is crucial for food preservation and material fabrication. The rate of freezing affects the quality of frozen goods and structural integrity of material products.
Solid to Gas: Sublimation
Sublimation refers to the direct transition of solid to gas without passing through the liquid phase. Common examples are dry ice turning into carbon dioxide gas. This phase transition is significant in processes like freeze-drying, which preserves food and biological samples effectively. Understanding sublimation aids in designing applications that depend on this unique transition, especially in pharmaceuticals and food technology.
Gas to Solid: Deposition
Deposition is the reverse of sublimation, where a gas changes directly into a solid. An example is the formation of frost when water vapor in the air solidifies on cold surfaces. It has applications in materials science, particularly in thin film deposition techniques used for electronics and coatings. Recognizing the conditions that lead to deposition can enhance product performance in various technological applications.
"Each type of phase transition has unique properties and applications, emphasizing their importance in science and technology."
"Each type of phase transition has unique properties and applications, emphasizing their importance in science and technology."
Overall, the study of phase transitions allows researchers and professionals to manipulate materials for desired outcomes, advancing knowledge in material development and practical applications.
Thermodynamics of Phase Changes
Thermodynamics deals with the laws governing energy and matter interactions. In the context of phase changes, it provides a critical cornerstone for understanding how various states of matter transition under different conditions. This section unpacks the key concepts in thermodynamics that are essential for comprehending phase changes, focusing on latent heat, phase diagrams, and critical points.
Latent Heat
Latent heat is the energy exchanged during a phase change without a change in temperature. This phenomenon is vital for understanding melting, freezing, vaporization, and condensation processes. In practical terms, when ice melts into water, or water vapor condenses into liquid, the energy involved is significant. This energy does not raise the temperature; instead, it facilitates the change between phases.
There are two main types of latent heat:
- Latent heat of fusion: This is the energy required for a substance to change from solid to liquid at its melting point, or vice versa. For water, the latent heat of fusion is approximately 334 joules per gram.
- Latent heat of vaporization: This is the energy absorbed when a liquid becomes a gas. For water, the latent heat of vaporization is significant, at around 2,256 joules per gram.
Understanding latent heat is essential for disciplines such as meteorology and engineering. For example, it plays a key role in weather patterns, as energy absorbed or released during phase changes can influence atmospheric conditions.
Phase Diagrams
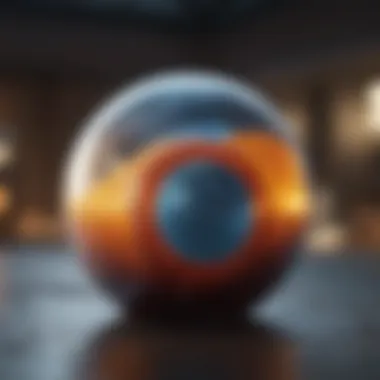
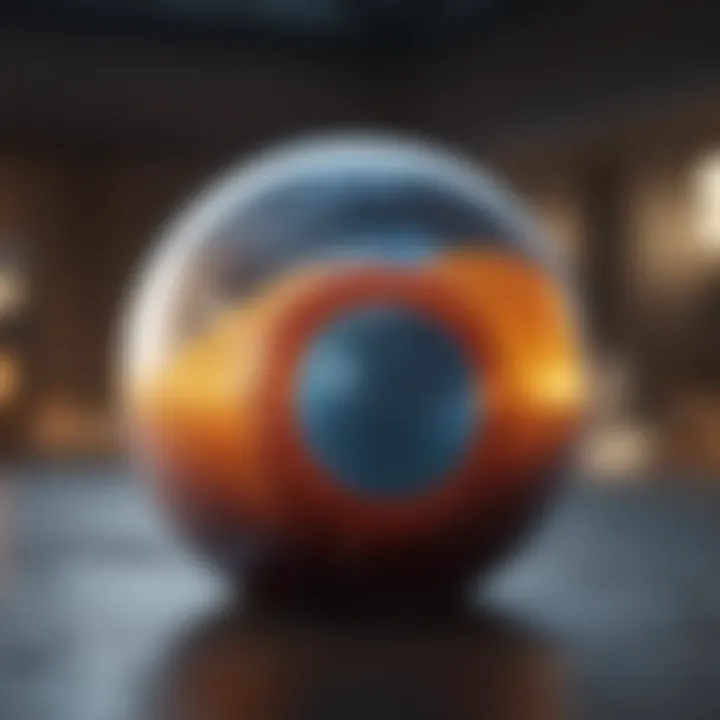
Phase diagrams graphically represent the relationship between temperature, pressure, and the states of a substance. They are instrumental in visualizing how phase changes occur at different conditions. Each section of the diagram indicates a specific state: solid, liquid, or gas. Additionally, it displays the boundaries (lines) that mark phase transitions. Understanding phase diagrams can enhance one's ability to predict material behaviour under varying conditions.
Key components of phase diagrams include:
- Triple point: The unique condition where all three phases coexist in equilibrium.
- Critical point: The end point of a phase equilibrium curve, beyond which distinct liquid and gas phases do not exist.
Phase diagrams are often used in various scientific fields, including materials science and chemistry, to inform manufacturing and research processes. They help professionals determine operational parameters for different substances.
Critical Points
The critical point refers to the highest temperature and pressure at which a substance can exist as a liquid and a gas. Above this critical point, the substance enters a supercritical fluid state, possessing properties of both liquid and gas. This unique situation allows for innovative applications in various fields, from drug delivery systems to extraction processes.
Understanding critical points helps professionals manipulate conditions effectively to achieve desired phase states. In research, this knowledge can lead to advancements in technologies such as supercritical fluid extraction, which is gaining attention for its benefits in creating pure extracts without chemical solvents.
"Understanding thermodynamics is crucial for comprehending how phase changes manifest in various materials and processes."
"Understanding thermodynamics is crucial for comprehending how phase changes manifest in various materials and processes."
In summary, thermodynamics offers significant insights into phase changes. Concepts such as latent heat, phase diagrams, and critical points allow for a deeper appreciation of material behavior under various conditions. As research advances, the implications of these principles will continue to resonate across scientific disciplines, enhancing the understanding and application of phase change phenomena.
Phase Change in Nature
Phase change plays a critical role in natural systems. Understanding this concept allows us to comprehend various ecological and environmental processes. The transformations between solid, liquid, and gas states are essential for maintaining life on Earth. Without these phase changes, ecosystems as we know them would not function properly.
Water Cycle
The water cycle is one of the most vital processes that illustrate phase change in nature. It encompasses evaporation, condensation, precipitation, and infiltration.
- Evaporation occurs when water from oceans, rivers, and lakes turns into vapor due to increased temperature. This phase change from liquid to gas is crucial as it contributes to the formation of clouds.
- Condensation follows. Water vapor cools and transforms back into liquid droplets, forming clouds. This change from gas to liquid is necessary for precipitation.
- Precipitation can take forms such as rain, snow, or sleet, which ultimately falls back to the earth's surface. This renews freshwater sources, essential for all forms of life.
Without these phase changes in the water cycle, arid environments would dominate, limiting biodiversity and affecting climatic conditions. The water cycle thus exemplifies how phase change is not merely a scientific concept, but a critical driver of ecological balance.
Weather Patterns
Phase changes significantly influence weather patterns and climate systems. Temperature variations leading to different states of matter impact atmospheric phenomena.
- Cloud formation is the result of condensation. Understanding how water vapor transitions back to liquid informs us about predicting storms and rain.
- Snowfall occurs when the atmospheric conditions cause liquid water to freeze rapidly. This can lead to a winter environment, affecting travel and agriculture.
- Heatwaves often arise when air masses stay stagnant and increase temperatures, leading to more evaporation. The consequences affect health, agriculture, and energy consumption.
The interactions of different phases are prime factors in shaping local and global weather conditions. By understanding these relationships, we can better predict and prepare for varying weather events, which have significant implications for environmental management and public safety.
The understanding of phase change is critical in predicting and managing natural processes.
The understanding of phase change is critical in predicting and managing natural processes.
In summary, phase change in nature underpins the fundamental processes essential for life and various environmental systems. From the water cycle to weather phenomena, these transformations affect everything from climatic patterns to ecological sustainability.
Applications of Phase Change
The applications of phase change are fundamental in various domains, impacting both industrial advancements and daily life. Understanding these applications allows for the harnessing of phase transitions to improve efficiency, sustainability, and functionality of many processes. The significance of phase change extends from manufacturing to climate control and energy management. As we examine the practical uses of phase change, it becomes clear that these principles are integral to modern technology and environmental science.
Industrial Processes
In the industrial sector, phase change is pivotal in processes like casting, welding, and metallurgy. During the solidification of metals, knowing how a material behaves while transitioning from liquid to solid can determine the strength and durability of the finished product. For instance, steel production relies on phase changes to achieve the desired material properties. Techniques such as quenching employ rapid cooling to control the phase transformation, promoting structural alterations that enhance hardness.
Furthermore, crystallization processes in pharmaceuticals utilize controlled cooling to ensure the consistency and efficacy of drugs. Proper understanding of phase change can lead to significant cost savings and quality improvements in manufacturing.
Refrigeration and Air Conditioning
Phase change plays a central role in refrigeration and air conditioning systems. These systems utilize refrigerants that absorb heat during vaporization and release it during condensation. This process is essential for maintaining desired indoor temperatures and preserving food products. By leveraging the latent heat of these substances, engineers design systems that are both efficient and effective.
Refrigeration cycles such as the Carnot cycle exemplify how optimal phase transitions can minimize energy consumption. It is crucial to select refrigerants with favorable thermodynamic properties. As environmental regulations shift towards greener alternatives, phase change research focuses on developing eco-friendly refrigerants.
Energy Storage Technologies
The realm of energy storage has seen innovation through the application of phase change materials (PCMs). These materials store and release thermal energy during phase transitions, specifically during melting and solidifying. PCMs are used in building materials to regulate indoor temperatures, capturing excess heat during the day and releasing it at night.
Another application involves the integration of PCMs in battery systems. They help manage heat, thus enhancing battery performance and lifespan. The ability to maintain optimal temperatures is pivotal as the energy sector moves towards sustainable solutions.
"Phase change materials bridge the gap between energy generation and consumption, optimally balancing both aspects."
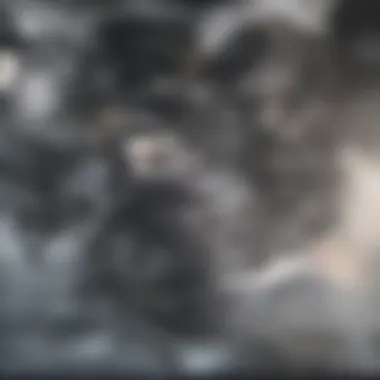
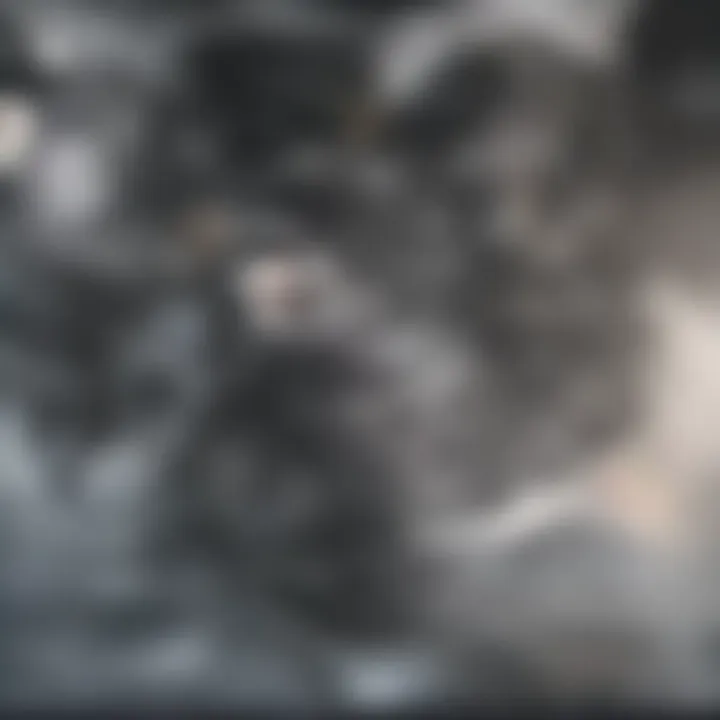
"Phase change materials bridge the gap between energy generation and consumption, optimally balancing both aspects."
In summary, the applications of phase change extend far beyond basic science. They encompass critical elements of industrial processes, climate control, and energy management. Understanding these applications reveals their importance in addressing the challenges of modern society. The ongoing research in phase change ensures that these principles evolve, paving the way for more efficient and sustainable systems.
Phase Change in Various Materials
Phase change in various materials is a significant aspect of understanding how different substances transition between states of matter. This section will discuss the role of phase change in metals and alloys, polymers, and biological materials. Each of these categories presents unique characteristics and implications when undergoing phase transitions. Understanding these differences is essential for applications in material science, engineering, and biology.
Metals and Alloys
Metals and alloys exhibit notable phase transitions that can significantly influence their mechanical properties and performance. For instance, steel, a common alloy, undergoes transformations during heating and cooling, affecting its toughness and ductility. The phase diagram of iron-carbon alloys illustrates this complex behavior, where various phases, such as ferrite and austenite, coexist.
The concept of latent heat is particularly relevant here. When a metal is heated, energy input leads to changes in structure without a temperature increase due to latent heat. The cooling process follows a different path, influencing the microstructure and, therefore, the material’s physical properties.
In industrial applications, understanding these transformations guides processes like heat treatment, which optimizes the strength and durability of metals and alloys.
Polymers
Polymers display unique phase change behaviors due to their long-chain molecular structure. Unlike metals, polymers can exhibit transitions such as glass transition temperature and melting temperature, both affecting their usability in various applications. Below the glass transition temperature, polymers are in a hard, glassy state. Above this temperature, they become more amorphous and flexible.
The phase transitions in polymers often affect their thermal and mechanical properties significantly, guiding their applications in industries from packaging to aerospace. For example, the melting transition of polyethylene allows it to be molded and shaped in production processes, while its glass transition point defines its usability in temperature-sensitive environments.
Biological Materials
Biological materials, such as proteins and lipids, undergo phase changes that are crucial for their functionality. These materials can display various phase transitions, significantly impacting their biological processes. For instance, when proteins unfold due to temperature increases, their functionality can be lost, leading to denaturation. Understanding these transitions can inform strategies for preserving biological samples across temperature variations.
Lipids, another category of biological materials, display phase change behavior that influences cell membrane fluidity. The extent of this behavior can affect cellular processes and is vital in research fields that study cell function.
Modern Research in Phase Change
Modern research into phase change plays a critical role in expanding our understanding of material behavior under varying conditions. This area of study seeks to improve how substances transition between states of matter by examining their underlying processes and applications. Researchers are particularly focused on advancing technology, enhancing energy efficiency, and facilitating innovative solutions in numerous fields. Understanding these changes can lead to breakthroughs in industries such as materials science, environmental sciences, and energy systems.
This section will delve into two significant areas driving modern research: nanomaterials and phase change materials (PCMs). Each represents a frontier in how we can manipulate phase change phenomena to address global challenges.
Nanomaterials
Nanomaterials are materials with structures at the nanoscale, typically ranging from 1 to 100 nanometers. The unique properties of these materials arise from their small size and large surface area relative to their volume. When it comes to phase change, nanomaterials can significantly alter the thermal properties of substances. For instance, silver nanoparticles have been used to enhance the thermal conductivity of certain polymer-based materials.
The advantages of incorporating nanomaterials in phase change studies include:
- Enhanced thermal management: Their increased surface area allows for improved heat transfer, which can lead to faster melting or solidification processes.
- Controlled phase transitions: Researchers can fine-tune the conditions under which phase changes occur, potentially leading to materials that change states at desired temperatures or energies.
- Applications in energy storage: Nanomaterials can be used to build more efficient energy storage systems. These systems can revolutionize how we harness and utilize renewable energy sources.
Phase Change Materials (PCMs)
Phase change materials (PCMs) are substances that absorb or release thermal energy during phase transitions. They are pivotal in thermal energy storage applications. PCMs can be solid or liquid and are designed to melt and solidify at specific temperatures, which makes them valuable in various industries. For instance, in building materials, PCMs can regulate indoor temperatures by absorbing excess heat during the day and releasing it when temperatures drop at night.
Key considerations in the research of PCMs include:
- Thermal efficiency: PCMs can improve energy efficiency in heating and cooling systems, reducing overall energy consumption.
- Environmental impacts: Implementing PCMs in construction and manufacturing can lower carbon footprints of buildings.
- Future developments: Ongoing research seeks to create new PCMs with better thermal properties and durability, expanding their practical applications.
Research in nanomaterials and phase change materials is essential. By manipulating material properties, scientists can address challenges in energy storage, thermal management, and material science.
Research in nanomaterials and phase change materials is essential. By manipulating material properties, scientists can address challenges in energy storage, thermal management, and material science.
By examining the interplay between nanomaterials and phase change materials, we can enhance our comprehension and capabilities regarding phase changes. This understanding not only benefits scientific inquiry but also has significant implications for practical applications across various domains.
The End
In the study of phase change, we find essential lessons that resonate across various scientific disciplines. Understanding how substances change from one state of matter to another offers insight into both theoretical and practical applications. The significance of this topic extends beyond mere academic interests; it plays a critical role in industries such as materials science, environmental studies, and engineering.
Recap of Key Concepts
Throughout this article, we have explored several key concepts related to phase change:
- Definition of Phase Change: A fundamental concept highlighting the transformation from solid to liquid, liquid to gas, and so forth.
- Thermodynamics: The importance of latent heat and phase diagrams helps us understand the energy aspects of transitions.
- Applications: From the water cycle in nature to innovative technologies like refrigeration and energy storage, phase change has widespread implications.
These discussions illustrate that the understanding of phase change not only assists in scientific research but also informs everyday applications that affect our daily lives.
Future Directions in Research
As we look to the future, several exciting opportunities for research and development emerge in the field of phase change. Potential areas for investigation include:
- Nanomaterials: The unique properties of nanomaterials during phase transitions can uncover new applications in electronics and energy storage.
- Software Modeling: Advances in computer simulations can provide deeper insights into phase change processes, accelerating discovery in materials science.
- Sustainable Technologies: Research into phase change materials (PCMs) can lead to improvements in energy efficiency and sustainability, especially in renewable energy systems.
Understanding the implications of phase change will continue to be a pivotal aspect of scientific inquiry. The interplay of temperature, pressure, and material properties is a dynamic area that promises to yield groundbreaking advancements.